DOI:
10.1039/B9PY00324J
(Paper)
Polym. Chem., 2010,
1, 663-669
Poly(3-dodedyl-2,5-thienylenevinylene)s from the Stille coupling and the Horner–Emmons reaction
Received
29th October 2009
, Accepted 8th December 2009
First published on
11th February 2010
Abstract
Regioregular (RR) conjugated polymers are critical for electronic and optoelectronic properties of polymer based semiconducting devices. Monosubstituted polythienylvinylene (PTV), a relatively low band-gap conjugated polymer, has been reportedly synthesized using the Stille coupling reaction between 3-dodecyl-2,5-dibromothiophene and (E)-1,2-bis(tributylstannyl)ethylene. Although a small fraction of the product shows good (∼90%) regioregularity, the whole product (S-C12-PTV) consists of mostly unidentified structures. To gain better control of the polymer structure and regioregularity, a difunctionalized C12-thiophene monomer, 3-dodecyl-5-formyl-thiophen-2-ylmethyl)-phosphonic acid diethyl ester, has been polymerized in near quantitative yield via the Horner–Emmons reaction. The full (100%) regioregularity of the resulting polymer (HE-C12-PTV) has been confirmed by its 1H and 13C NMR spectra. The full regioregularity is also reflected in its strong tendency to crystallize and practically no solubility in boiling hexane, in sharp contrast to S-C12-PTV. UV-vis absorption spectroscopy, fluorescence spectroscopy, cyclovoltammetry, thermal analysis (DSC & TGA) and X-ray diffraction have been used to characterize both polymers. UV-vis absorption spectra of the HE-C12-PTV chloroform solutions of different concentrations show well-resolved vibronic structures with an absorption maximum at 577 nm and a prominent shoulder at 614 nm. The optical bandgaps are 1.80 eV in chloroform solution and 1.65 eV in film. The HOMO/LUMO energy levels of the HE-C12-PTV film were found to be at −4.98 eV and −2.88 eV, respectively. The electrochemical bandgap of the polymer in the film is estimated to be 2.1 eV. The DSC curve shows a pronounced melting peak at 205 °C. XRD study shows that a decent crystalline structure is formed without any annealing of the as-cast films.
Introduction
Polythienylenevinylenes (PTVs) are an important class of low bandgap polymers and have been investigated for optoelectronic applications.1 Substituted PTVs have been synthesized using several methods, including Ni-catalyzed Grignard coupling of 1,2-bis(3,4-dibutoxythiophene)ethylene with 1,2-dichloroethylene,2 a precursor polymer route via bis(sulfoxomethyl) derivatives of 3,4-dialkoxythiophenes,3 Stille coupling reactions,4 Heck coupling reactions,4a and Horner–Emmons reactions.5 Most of the reported substituted PTVs have two side chains on each thiophene unit. Disubstitution improves solubility, but tends to prohibit tight π-stacking6 which is crucial to some opto-electronic applications such as photovoltaics and LEDs.
Mono n-dodecyl-substituted PTVs have been reportedly synthesized by the Stille coupling reaction between dibromothiophene and 1,2-bis(tributyltin)ethylene and by the Heck coupling reaction (Scheme 1).4a The Stille coupling was considered a better method in terms of structural regioregularity.4a However, even with this method, a decent regioregularity (∼90%) was reported only for a small fraction (9.5%, hexane-insoluble) of the Stille coupling product (S-C12-PTV).4a
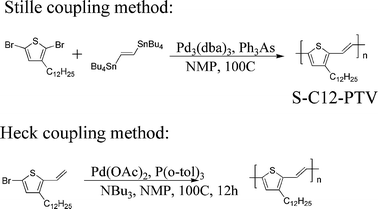 |
| Scheme 1 Literature synthesis of C12-PTV.4a | |
The Horner–Emmons reaction has been widely used to synthesize vinylene-based polymers, including symmetrically substituted poly(p-phenylenevinylene)s (PPVs),7 regioregular RO-PPVs8 and 3,4-disubstituted PTV.9 In this work, this highly reliable reaction is utilized to synthesize monosubstituted C12-PTV (HE-C12-PTV) from a difunctionalized monomer, 3-dodecyl-5-formyl-thiophen-2-ylmethyl)-phosphonic acid diethyl ester (Scheme 2) in a nearly quantitative yield. This unsymmetrically functionalized monomer, plus the high reliability of the reaction, leads to full head-to-tail regioregularity. Full content S-C12-PTV is also synthesized using the literature procedure.4a HE-C12-PTV and S-C12-PTV are compared by using a number of analysis techniques, including NMR, UV-vis absorption, thermal analysis, cyclovoltammetry, and XRD. The results indicate that S-C12-PTV has very low structural regularity, much lower crystallinity (in film) and contains structural defects that cause the polymer to decompose at much lower temperatures. The chemistry reported here for fully regioregular C12-PTV can be modified for the synthesis of regioregularity-controllable C12-PTV which allows for further investigation of this polymer for optoelectronic applications.10
 |
| Scheme 2 Synthesis of the fully regioregular C12-PTV. | |
Results and discussion
Synthesis of C12-PTVs
HE-C12-PTV is synthesized in six steps (Scheme 2) from 2-bromo-3-dodecylthiophene11 (1). The selectivity of the reaction for 2-bromination is 97% according to the 13C NMR analysis of the crude product. After the bromide is converted into carboxaldehyde, the two isomeric aldehydes were separated by column chromatography. Conversion of 3 into 4 is very fast and neat with thionyl chloride at ∼10 °C. However, due to the similarity between the NMR spectra of 3 and 4, misjudgment of the reaction progress can happen if the 1H NMR spectrum of the reaction product is not examined carefully. 4 is converted via the Arbuzov reaction into the phosphonate 5 which is formylated by Cl2CHOMe/TiCl4 to give the monomer 6 in a quantitative yield.12 Polymerization of monomer 6 is very fast at room temperature with t-BuOK as the base and is completed in less than half an hour. Yields above 90% are routinely obtained. However, it was found that the reverse addition resulted in no polymer product or an incomplete reaction. This can be explained by the following: when the monomer is added to the base, all monomer molecules are deprotonated at the Th-CH2–P carbon immediately, and the CHO group in a deprotonated 6 is no longer reactive toward the carbanion in another monomer or an oligomer.
S-C12-PTV is synthesized using the literature procedure.4a The full content of the product (ppt in MeOH, 65% yield) is used in the characterization and comparison below.
Characterization of HE-C12-PTV and S-C12-PTV
Solubility.
S-C12-PTV is almost completely soluble in hexane, while HE-C12-PTV is only soluble in solvents such as chloroform and 1,2-dichlorobenzene (DCB). At elevated temperatures (e.g.90 °C), HE-C12-PTV can be dissolved in 1,2-dichlorobenzene at a concentration of 2 wt/vol%, sufficiently high for film casting. However, the polymer crystallizes as soon as the solution is applied onto glass substrates. This high tendency for crystallization is a result of its high structural regularity and high purity as discussed below.
NMR spectra and regioregularity.
The polymerization for HE-C12-PTV only involves one monomer with unsymmetrical difunctionalities. This, coupled with high reliability of the Horner–Emmons reaction,7 ensured head-to-tail linkage and full regioregularity. This is supported by the appearance of a clean singlet for the thienylenic proton (c) and two sets of clean doublets for the two ethenic protons (a and b) in 1H NMR spectrum (Fig. 1) and only six peaks in the aromatic region of the 13C NMR spectrum (Fig. 1). Although the Horner–Emmons reaction is known to produce cis C
C bonds (as a minor product) in certain polymers such as PPV,7 only trans C
C bonds exists in the synthesized HE-C12-PTV. This is supported by two observations: (1) only one Th–CH2 peak is seen in the 1H NMR spectrum. (2) The 1H NMR peaks in the aromatic region matches with the main peaks of C12-PTV produced via the Heck coupling reaction, which is known to produce predominantly trans C
C bonds (and, unfortunately, a significant amount of α-linkage).4a
In the 1H NMR spectrum of S-C12-PTV (Fig. 2, an NMR spectrum of full-content Stille C12-PTV, not available in the literature), although three peaks at 6.77, 6.88 and 6.93 ppm from the regioregular segments in the polymer can be seen (corresponding to protons c, a and b), a broad bump, where the three peaks sit on, is dominant in the aromatic region. This bump and the broad Th–CH2 peak (proton d) around 2.65 ppm are indications of structural complexity in the polymer.
Molecular weight (MW) by GPC.
Due to the limited solubility of HE-C12-PTV in THF at the ambient temperature, the polymer product precipitates from the reaction mixture. The number average MW (Mn) of HE-C12-PTV is relatively low (7.2 K). Its polydispersity is 2.9. On the other hand, S-C12-PTV has a Mn of 8.7 K and polydispersity of 2.4.
Optical and electrochemical properties.
UV-vis absorption spectra of HE-C12-PTV in chloroform solution (which has a violet blue color) of different concentrations (0.01 mM and 1 mM in chlolroform) and in thin film (drop-cast from the o-dichlorobenzene solution) are shown in Fig. 3. The absorption curves of the solutions of two concentrations show maxima at the same wavelength (577 nm) and both have a prominent shoulder at 614 nm. A shoulder peak in the absorption spectrum of the high concentration (1 mM) is seen at 665 nm, which is absent when the concentration is low. The shoulder peak is attributed to aggregation of the polymer in chloroform solution,13 and matches well with the similar shoulder peak in the film absorption spectrum (666 nm). The thin film has a λmax of 574 nm, and a band edge at 752 nm (1.65 eV), red-shifted by 62 nm (0.15 eV) from the band edge (690 nm, 1.80 eV) of the chloroform solution (0.01 mM).
UV-vis absorption spectra of S-C12-PTV chloroform solutions (which have a violet color) and film are shown in Fig. 4. No fine structure is seen and a 22 nm blue shift in the peak wavelength is observed for the optical absorption in the film compared to that in the chloroform. The blue shift is an indication of a lack of π–π interaction in the solid state, which is likely to be a result of poor regularity and structural defects in the polymer. Compared to the absorption peak of HE-C12-PTV (574 nm) or the hexane-insoluble fraction of S-C12-PTV (576 nm) in chloroform,4a the λmax of S-C12-PTV in chloroform is blue shifted by 22–24 nm. This indicates that the absorption peak of hexane-soluble fraction of S-C12-PTV is at a shorter wavelength and dominates the absorption profile of the whole product. Accordingly, the absorption band edge of the film (735 nm, 1.69 eV) is blue-shifted by 17 nm as compared to that of the HE-C12-PTV film.
C12-PTVs have very weak photoluminescence, not measurable on a commercial fluorescence spectrometer. No PL spectra have been reported in the literature. Using an ISA Fluoromax-3 luminescence spectrofluorometer and a customary photoinduced absorption spectroscopic setup, which consists of a Newport Oriel Cornerstone 1/4 m monochrometer together with various diffraction gratings and optical filters to span the spectrum from 0.2 to 3.0 eV, PL spectrum of HE-C12-PTV was successfully obtained (Fig. 5) from its dilute solution in o-dichlorobenzene. The shoulder peaks on the long wavelength slope are due to the vibronic structures of the polymer. Aggregation of the polymer, another possible origin of the shoulder peaks, is ruled out as the concentration of the solution is below 0.01 mM.
Cyclovoltammetry (CV) is used to identify the HOMO/LUMO energies based on the correlation between oxidation/reduction potentials and the HOMO/LUMO levels. The CV scan of C12-PTV film coated on a Pt working electrode is shown in Fig. 6. The HOMO/LUMO values were found to be −4.98 eV and −2.88 eV respectively. The electrochemical energy gap (Eg,el) is estimated to be 2.07 eV, which is larger than the optical bandgap (1.65 eV) Eg,el is larger because the electrode potential must be lower than the HOMO of the neutral polymer for the oxidation to happen and must be higher than the LUMO of the neutral polymer for the electron to transfer from the electrode into the LUMO orbital. The redox of the polymer in the potential range from 0 to 0.8 V is completely reversible, at least for the nine cycles performed, while in the range from 0 to −2.2 V, the redox is irreversible. This indicates that the polymer is stable at oxidized state and not stable at the reduced state. The CV behavior of S-C12-PTV (as shown in Fig. 7) is similar to HE-C12-PTV (the results listed in Table 1).
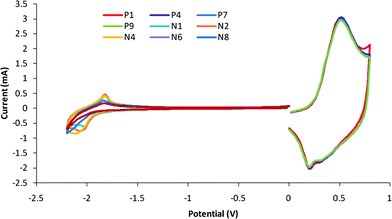 |
| Fig. 6 Cyclovoltammograph of HT-HT C12-PTV film coated on Pt wire. Reference electrode: Ag in 0.1M AgNO3. | |
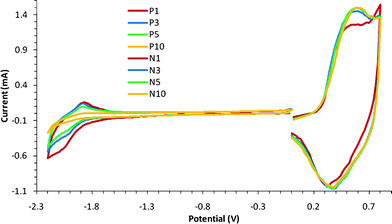 |
| Fig. 7 Cyclovoltammograph of S-C12-PTV film coated on Pt wire. Reference electrode: Ag in 0.1M AgNO3. | |
Table 1 Properties of C12-PTVs
|
Mn/PDI |
λ
max CHCl3/film |
Egopt (film) |
Eox/Ered onset/V |
HOMO/LUMO and Eg,el/eV (film) |
T
d/°C |
HE-C12-PTV |
7.2 K/2.9 |
577/574 |
1.65 |
0.16/−1.94 |
2.07 |
326 |
S-C12-PTV |
8.7 K/2.4 |
552/530 |
1.69 |
0.22/−1.76 |
1.98 |
158 |
Thermal analysis and stability tests.
The phase transition and thermal stability were examined by differential scanning calorimetry (DSC), thermal gravimetric analysis (TGA) combined with NMR analysis. The DSC curve (Fig. 8) shows a pronounced melting peak at 205 °C for HE-C12-PTV. The melting is confirmed by visual inspection of a sample heated to a temperature (230 °C) above the melting range. 1H NMR analysis of a sample heated at 280 °C both for 10 min in the DSC under N2 atmosphere shows no decomposition. So, the endothermic slope between 230 °C and 280 °C is not due to a chemical reaction process, but rather a physical transition (presumably a transition from liquid crystal phase to isotropic liquid). The onset at 326 °C of an exothermic transition (with a peak at 358 °C) indicates the beginning of chemical decomposition of the polymer, most likely crosslinking reactions involving non-aromatic C
C bonds as confirmed by the insolubility of the sample after heating at 350 °C. The weight loss onset temperature is determined from TGA scan (Fig. 9) to be 373 °C. In the DSC curve of S-C12-PTV, no melting peak can be seen. Its TGA curve shows an early weight loss with an onset temperature at about 158 °C and 10% weight loss at 219 °C. It was also found that the polymer becomes insoluble after 3 months of storage at room temperature in dark (not observed for HE-C12-PTV under the same condition). The fact that S-C12-PTV has a much lower thermal stability suggests that S-C12-PTV contains significant amount of structure defect which is not stable and can cause the polymer to crosslink even at room temperatures.
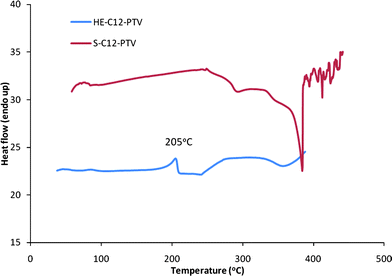 |
| Fig. 8 DSC curve of HT-HT C12-PTV power sample in N2 at a scan rate of 10 °C min−1. | |
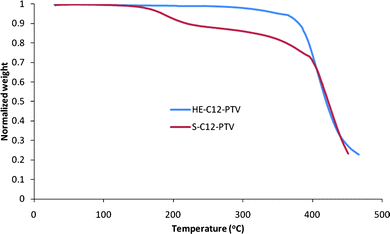 |
| Fig. 9 TGA curve of HT-HT C12-PTV powder sample in N2 at a scan rate of 10 °C min−1. | |
X-Ray diffraction (XRD) measurements.
Wide angle out-of-plane diffraction pattern is obtained on XRD Spectra are recorded using Rigaku X-Ray Diffractometer (Model D/max-2200) (Cu Kα radiation, λ = 1.5406 Å) for as-cast C12-PTV films on glass substrate from hot o-dichlorobenzene solution (Fig. 10). The average thickness of the films is ∼2 μm. Similar peaks are seen for both HE- and S- C12-PTV films. However, the peaks for S-C12-PTV film is much weaker, in agreement with the lack of distinct melting peak in the DSC scan. A calculation based on the first diffraction peak (100) gives an interlayer spacing of 17.6 Å, which is smaller than the d-spacing of 27.19 Å in HT-poly(3-dodecylthiophene) (P3DDT) film14 by a factor of 1.54. The distance is consistent with structural models, where the side-chains of C12-PTV are interdigitated or closed packed and tilted toward the backbone.
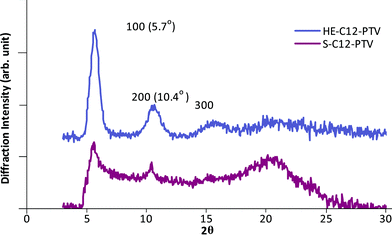 |
| Fig. 10 Wide angle X-Ray diffraction pattern of an as-cast C12-PTV film (thickness ∼2 μm) drop-cast from o-dichlorobenzene solutions. | |
Mobility measurement.
S-C12-PTV films of 300 nm thickness was spin-coated from 2 its 2 wt% solution in 1,2-DCB onto ITO/glass slides. The active area is ∼4 mm2 of thermally deposited Au film (50 nm thick). Under the step voltage of 20 V, the transient current is dominated by SCLC as demonstrated in the insert of Fig. 11(b). The time lapse at peak transient current (t1) is about 20 μs. The circuit response time (t = RC, R = 1 Kohm, and C = 0.3 nF) is about 0.3 μs, much smaller than t1. The mobility (μ)15 is calculated to be ∼2 × 10−6 cm2/Vs from with
d = 300 nm, t1 = 20 μs, and V = 20 V.
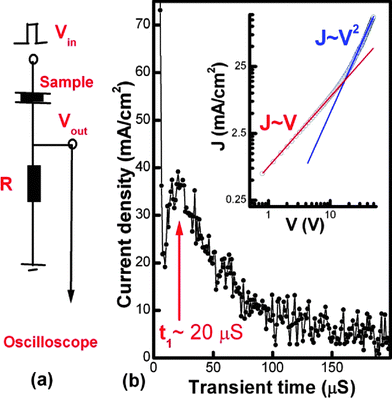 |
| Fig. 11 The measurement circuit and the plot of the transient current as a function of time. A step voltage (20 V with duration time of 1 ms and repetition rate of 100 Hz.) from signal generator (SRS model DS345) is applied to device as an input voltage (Vin). The transient current is measured through a serial resistor of 1 K Ohm. The correspondent output voltage (Vout) is then sent to a digital oscilloscope (Tektronix TDS360). | |
The mobility measurement was also attempted for HE-C12-PTV, however, the polymer crystallizes so fast during film casting that good quality films could not be prepared and reliable/meaningful data could not be obtained. The chemistry to control the regioregularity and processibility of HE-C12-PTV has been developed.10 More systematic investigation of the effect of regioregularity on mobility and solar cell device performance is currently underway.
Experimental
Starting materials, instrumentation, and general methods
All starting materials, reagents and solvents were purchased from commercial sources (mostly from Sigma-Aldrich and Fisher-Scientific) and used directly unless noted otherwise. NMR spectral data were obtained from a Bruker Avance 300 MHz spectrometer with TMS as the internal reference. Elemental analysis is performed by Atlantic Microlab Inc. UV-vis absorption data were collected on a Varian Cary-5 spectrophotometer. Thermal analysis is performed on a Perkin-Elmer TGA6/DSC6 system. The polymer molecular weights were measured on a Viscotek T60A/LR40 triple-detector GPC system at ambient temperature. Polystyrene standards were used for calibration.
Electrochemical studies were performed on a Bioanalytical (BAS) Epsilon-100w tri-electrode cell system. Three electrodes are a Pt working electrode, an ancillary Pt electrode, and a silver reference electrode (in a CH3CN solution of 0.01 M AgNO3 and 0.1 M TBA-HFP). The polymer samples were dissolved in hot solvents (such as o-dichlorobenzene) and then coated onto a Pt working electrode. The measurements were performed in 0.1 M TBA-HFP solution in acetonitrile. Between the experiments, the surface of the electrodes were cleaned or polished. Scan rate is 100 mV s−1. Ferrocene (2 mM in 0.10M TBA-HFP/CH3CN solution) is used as an internal reference standard and its HOMO level of −4.8 eV is used in calculations.
3-Dodecylthiophene-2-carboxaldehyde (2).
A literature procedure16 is used with minor modifications. A mixture of 2-bromo-3-dodecylthiophene (64.76 mmol, 21.45 g), Mg (1.652 g) and anhydrous THF (60 mL) is heated under reflux. Methylmagnesium bromide solution (3M in ether, 3 mL) is added to initiate the reaction. 2.5 h later, the mixture is cooled to RT, and DMF (5.5 mL, anhydrous) is then added via a syringe over 5 min. Fifteen minutes later, the reaction mixture is poured into a stirred mixture of hexanes (200 mL) and 2N HCl (70 ml). The hexanes is separated and washed with water two times and with aqueous NaHCO3 solution one time. The hexanes extract is dried with MgSO4, and then condensed. The crude product is purified by silica gel column chromatography using EtOAc/hexanes mixture (starting from pure hexane to 1
:
50 v/v) as the eluent. Yield: 92.4%. 1H NMR (CDCl3): the same as reported for 3-decylthiophene-2-carboxaldehyde, except the expected difference in aliphatic proton count.
2-Chloromethyl-3-dodecylthiophene (4).
Thionyl chloride (2.36 ml, 36.43 mmol) is added over 1.5 min to a solution of 3-dodecyl-thiophene-2-methanol (8.23 g, 29.2 mmol), triethylamine (12.9 mL, 87.5 mmol) and methylene chloride (50 mL) cooled in an ice bath. The solution is stirred for 30 min, then, poured to ice/hexanes. The mixture is acidified with 30 mL of 2N aq. HCl. The hexanes extract is washed with water to neutral, dried with MgSO4, and condensed to give 9.40 g of crude product (theoretical yield: 8.76 g), >95% pure by both proton and carbon NMR spectra. 1H NMR (CDCl3): δ 0.88 (t, 3H, J = 7.4 Hz), 1.2–1.4 (m, 18H), 1.63 (m, 2H), 2.61 (t, 2H, J 7.4Hz), 4.77 (s, 2H, CH2Cl), 6.85 (d, 1H, J = 5.1 Hz), 7.22 (d, 1H, J = 5.1 Hz). 13C NMR (CDCl3): δ 14.13, 22.71, 28.22, 29.55 (a few peaks overlapped), 30.90, 31.94, 46.20, 125.13, 129.00, 132.66, 142.49. The product is used in the next step without further purification (due to limited stability in silica gel column).
(3-Dodecyl-thiophen-2-ylmethyl)-phosphonic acid diethyl ester (5).
A mixture of 2-chloromethyl-3-dodecylthiophene (9.40 g crude, 29.2 mmol) and triethyl phosphite (8.10 g, 44.4 mmol) is stirred at 140 °C for 4.5 h. 1H NMR analysis of the reaction mixture indicated that the reaction is complete and neat. Excess triethyl phosphite is removed by vacuum distillation. The crude product, showing only a small number of impurities in aromatic region, is purified by column chromatography on silica gel using 1/12 (v/v) ethyl acetate/hexanes mixture as the eluent. Yield: 5.0 g, 43%. Some product may have decomposed in the column during the long separation process. 1H NMR (CDCl3): δ 0.88 (t, 3H, J = 7.4 Hz), 1.1–1.4 (m, 24H), 1.58 (m, 2H), 2.56 (t, 2H, J = 7.5 Hz), 3.25 (t, 2H, J = 20.7 Hz, –CH2P), 4.04 (m, 4H, P–O–CH2–), 6.80 (d, 1H, J = 5.3 Hz), 7.06 (d, 1H, J = 5.3 Hz). 13C NMR (CDCl3): δ 13.81, 16.20/16.27 (d, OCH2CH3), 25.61, 27.53, 28.35, 29.21, 28.45, 29.5, 30.34, 32.84, 62.11 (d, J = 7.1 Hz), 122.95 (d, J = 4.4 Hz), 126.05 (d, J = 11 Hz), 128.40 (d, J = 3.8 Hz), 140.64 (d, J = 9.9 Hz). Anal. Calcd.: C, 61.37; H, 9.13; S, 7.45. Found: C, 61.09; H, 9.22; S, 7.31.
3-Dodecyl-5-formyl-thiophen-2-ylmethyl)-phosphonic acid diethyl ester (6).
Titanium tetrachloride (1.20 ml, 10.9 mmol) is added dropwise to a ice-cooled mixture of (3-dodecyl-thiophen-2-ylmethyl)-phosphonic acid diethyl ester (1.07 g, 2.66 mmol), α,α-dichloromethyl methyl ether (0.321 g, 2.81 mmol) and methylene chloride (10 ml) over 10 min. The solution is stirred in the ice bath for 3.5 h, and then transferred into a separation funnel with NaHCO3 solution and hexanes already added. The hexane phase is washed with brine two times and dried over anhydrous MgSO4. The solvent is removed by rotary evaporation. Proton NMR of crude product showed no side reactions, and the yield is quantitative. Still, a silica gel column chromatography using ethyl acetate/hexanes mixture as eluent (with Rf 1/8–16 for the product) is performed to ensure high purity for the product (>99% by NMR, supported by the elemental analysis result). 1H NMR (CDCl3): δ 0.88 (t, 3H, J = 7.4 Hz), 1.1–1.4 (m, 24H), 1.58 (m, 2H), 2.60 (t, 2H, J = 7.0 Hz), 3.34 (d, 2H, J = 21.66 Hz, –CH2P), 4.10 (m, 4H, P–O–CH2–), 7.55 (s, 1H), 9.81 (s, 1H, CHO). 13C NMR (CDCl3): δ 14.13, 16.41 (d, J = 6.0 Hz, CH2P), 22.69, 26.44, 28.20, 28.34, 29.35, 29.44, 29.49, 29.58, 29.64, 29.67, 30.18 (d, J = 1.6 Hz, OCH2CH3), 31.92, 62.62 (d, J = 6.1 Hz, OCH2CH3), 137.70 (d, J = 4.4 Hz), 138.11 (d, J = 12.1 Hz), 141.14 (d, J = 3.8 Hz), 142.65 (d, J = 8.8 Hz), 182.57. Anal. Calcd. C, 61.37; H, 9.10; S, 7.32. Found: C, 61.09; H, 9.22; S, 7.31.
C12-PTV.
In a glovebox, a solution of t-BuOK (1.2 eq, 88.6 mg) in THF (3mL) is added over 3 min to a solution of 3-dodecyl-5-formyl-thiophen-2-ylmethyl)-phosphonic acid diethyl ester (263 mg, 0.611 mmol) in THF (10 mL) at room temperature. The solution became blue almost immediately after addition of the base, and polymer precipitate appeared in less than five minutes. Thirty minutes after addition, the reaction mixture is dropped into methanol. The polymer product is collected by filtration. Yield: 0.157 g, 93%. 1H NMR (CDCl3): δ 0.88 (t, 3H), 1.2–1.4 (m, 18H), 1.62 (m, 2H), 2.62 (t, 2H), 6.77 (s, 1H), 6.86 (d, 1H, J = 15.8 Hz), 6.98 (d, 1H, J = 15.8 Hz). 13C NMR (CDCl3): δ 14.05, 22.73, 28.54, 29.41, 29.45, 29.57 (two peaks overlapped here), 29.68, 29.73, 29.77, 30.84, 32.01, 120.07, 121.03, 129.17, 135.63, 140.65, 142.49.
Summary, conclusions and future studies
Fully regioregular HT-HT C12-PTV has been synthesized and characterized by NMR, UV-vis absorption spectroscopy, fluorescence spectroscopy, cyclovoltammetry, thermal analysis and XRD. Full regioregularity is ensured by a combination of three measures: the polymerization scheme which only involves one monomer with asymmetric difunctionalities, high reliability of the Horner–Emmons reaction, and the high purity of the monomer. UV-vis absorption spectra of the HT-HT C12-PTV chloroform solutions of different concentrations show well-resolved vibronic structure with an absorption maximum at 577 nm and a prominent shoulder at 614 nm. The band edge is 690 nm (1.80 eV, optical bandgap). The band edge of the electronic transition in thin film is 752 nm (1.65 eV). The PL intensity of C12-PTV is very weak with a peak at 662 nm. The HOMO/LUMO energy levels of the C12-PTV film were found to be −5.03 eV and −3.63 eV respectively. The electrochemical bandgap is estimated to be 1.4 eV. The DSC curve shows a pronounced melting peak at 205 °C followed by an endothermic transition of LC phase to isotropic liquid in the temperature range of 242–280 °C. The onset temperature of chemical decomposition of the polymer is about 326 °C by DSC, while the onset temperature of polymer disintegration is about 373 °C as determined by TGA.
The polymerization scheme adopted here allows systematic tuning of regioregularity by simply adding a certain amount of co-monomer which is an isomer of compound 6. Systematic studies on the effect of regioregularity control versus C12-PTV solubility, film morphology, and performance of solar cells are currently under way and to be reported separately.
Acknowledgements
This work is supported, in part, by the following grants/contracts: NASA (NCC3-1035), DOD (W911NF0610488 and FA8650-07-C-5058). One of us (XJ) is grateful for the donors of the American Chemical Society Petroleum Research Fund for financial support and would also like to acknowledge Gayan Dedigamuwa and Robert Hyde for their help with the XRD measurement.
References and Notes
- A. P. Smith, R. R. Smith, B. E. Taylor and M. F. Durstock, Chem. Mater., 2004, 16, 4687–4692 CrossRef CAS
; K. Van De Wetering, C. Brochon, C. Ngov and G. Hadziioannou, Macromolecules, 2006, 39, 4289–4297 CrossRef
; F. Banishoeib, A. Henckens, S. Fourier, G. Vanhooyland, M. Breselge, J. Manca, T. J. Cleij, L. Lutsen, D. Vanderzande, L. H. Nguyen, H. Neugebauer and N. S. Sariciftci, Thin Solid Films, 2008, 516(12), 3978–3988 CrossRef CAS
; J. Hou, Z. Tan, Y. He, C. Yang and Y. Li, Macromolecules, 2006, 39(14), 4657–4662 CrossRef CAS
.
- M. L. Blohm, J. E. Pickett and P. C. Van Dort, Macromolecules, 1993, 26, 2704–2710 CrossRef CAS
.
- H. Cheng and R. L. Elsenbaumer, J. Chem. Soc., Chem. Commun., 1995, 1451–2 RSC
.
-
(a) R. S. Loewe and R. D. McCullough, Chem. Mater., 2000, 12, 3214 CrossRef CAS
;
(b) E. E. Havinga, C. M. J. Mutsaers and L. W. Jenneskens, Chem. Mater., 1996, 8, 769–776 CrossRef CAS
.
- K. Van De Wetering, C. Brochon, C. Ngov and G. Hadziioannou, Macromolecules, 2006, 39, 4289–4297 CrossRef
.
- Good crystal packing for dialkyl substituted thiophene polymers has not been reported in the literature..
- C. Zhang, S. Choi, J. Haliburton, T. Cleveland, R. Li, S. Sun, A. Ledbetter and C. BonnerJr, Macromolecules, 2006, 39, 4317–4326 CrossRef CAS
.
- Y. Suzuki, K. Hashimoto and K. Tajima, Macromolecules, 2007, 40, 6521–6528 CrossRef CAS
.
- K. Van De Wetering, C. Brochon, C. Ngov and G. Hadziioannou, Macromolecules, 2006, 39, 4289–4297 CrossRef
.
- C. Zhang, E. Annih, R. Li and S.-S. Sun, Photovoltaic and display materials, Proc. SPIE, 2009, 7213 Search PubMed
, paper 7213–8.
- P. Baeuerle, F. Pfau, H. Schlupp, F. Wuerthner, K. U. Gaudl, C. Balparda and F. Miguel, J. Chem. Soc., Perkin Trans. 2, 1993, 489–494 RSC
.
- C. Zhang, A. W. Harper and L. R. Dalton, Synth. Commun., 2001, 31(9), 1361–1365 CrossRef CAS
.
- A. V. Gavrilenko, T. D. Matos, C. E. Bonner, S.-S. Sun, C. Zhang and V. I. Gavrilenko, J. Phys. Chem. C, 2008, 112(21), 7908–7912 CrossRef CAS
.
- T.-A. Chen, X. Wu and R. D. Rieke, J. Am. Chem. Soc., 1995, 117, 233–244 CrossRef CAS
.
-
M. A. Lampert and P. Mark, Current Injection in Solids, Academic Press, New York, 1970, pp. 112–138 Search PubMed
; D. Poplavskyy, J. Nelson and D. C. Bradley, Macromol. Symp., 2004, 212, 415–420 Search PubMed
.
- M. He, T. M. Leslie and J. A. Sinicropi, Chem. Mater., 2002, 14, 4662–4668 CrossRef CAS
.
|
This journal is © The Royal Society of Chemistry 2010 |
Click here to see how this site uses Cookies. View our privacy policy here.