DOI:
10.1039/C0CC02152K
(Communication)
Chem. Commun., 2011,
47, 469-471
Dinuclear Ni2–Schiff base complex-catalyzed asymmetric 1,4-addition of β-keto esters to nitroethylene toward γ2,2-amino acid synthesis†
Received
28th June 2010
, Accepted 16th September 2010
First published on 4th October 2010
Abstract
A homodinuclear Ni2–Schiff base 1 complex (1–10 mol%) promoted the catalytic asymmetric 1,4-addition reactions of β-keto esters and an N-Boc oxindole to nitroethylene, giving products in 98–75% ee.
Catalytic asymmetric 1,4-additions of carbon nucleophiles are highly useful synthetic transformations for the construction of quaternary carbon stereocenters.1,2 Over recent years, there has been tremendous progress in both metal catalysis and organocatalysis for the asymmetric 1,4-additions of β-keto esters and related compounds.2 β-Substituted nitroalkenes are most often used as acceptors, giving β-substituted γ-nitro esters with α-quaternary carbon stereocenters.3 The use of a β-unsubstituted acceptor (nitroethylene), however, is much less common,4,5 possibly due to potential difficulties of enantiocontrol and competitive polymerization of nitroethylene. Gellman et al. and Wennermers et al. independently reported highly enantioselective organocatalytic asymmetric 1,4-additions of aldehydes to nitroethylene and their applications to γ2-amino acid synthesis,4 but α,α-disubstituted aldehydes were not utilized as donors. Barbas was the first to successfully construct a quaternary carbon stereocenter viacatalytic asymmetric 1,4-addition of an N-Boc oxindole to nitroethylene.5 Because γ2-amino acids are potentially useful building blocks for folder research and the synthesis of biologically active compounds,6,7 further studies to expand the scope of donors, especially for the synthesis of adducts with a quaternary stereocenter adjacent to a carbonyl group γ2,2,8,9 are highly desirable. To address this issue, we herein report the utility of a homodinuclear Ni2–Schiff base 1 complex (Fig. 1). Ni2–1 (1–10 mol%) promoted the reactions of β-keto esters to nitroethylene, affording products in 98–75% ee.
As part of our ongoing studies of bifunctional Lewis acid/Brønsted base catalysis in collaboration with Shibasaki,10 one of authors (S.M.) and Shibasaki recently reported the utility of dinuclear Schiff base complexes.11–15 Among them, homodinuclear Co2–112a,b and Mn2–112c complexes were suitable for 1,4-additions with β-substituted nitroalkenes. Thus, we first applied Co2–1 and Mn2–1 complexes for the reaction of β-keto ester 2a and nitroethylene. Both of these complexes, however, gave an unsatisfactory yield and enantioselectivity (Table 1, entries 1 and 2, less than 30% NMR yield and 67% ee). Among the homodinuclear12,13 and heterodinuclear14Schiff base complexes screened, the Ni2–1catalyst13 afforded promising results. The reaction of β-keto ester 2a with 1.2 equiv. of nitroethylene in THF at 0 °C gave product 3a in 75% yield and 97% ee (entry 3). Among the solvents screened (entry 3–8), AcOEt was the best in terms of yield and enantioselectivity (entry 8, 96% yield and 98% ee). We then tried to reduce catalyst loading in entries 9–12. With 2.5 mol% catalyst, reactivity was decreased at 0 °C and the reaction did not complete after 48 h (entry 10). Raising the reaction temperature to 40 °C drastically improved the reactivity and the reaction completed within 5 h with high enantioselectivity (entry 12, 93% ee).
Table 1 Optimization of reaction conditionsa
Entry |
M |
x
|
Solvent
b |
Temp/°C |
Time/h |
% Yieldc |
% ee |
1.2 equiv. of nitroethylene (in toluene) were used.
Reactions were run in solvent/toluene = 10 ∶ 1, because nitroethylene stored in toluene was used.
Yield was determined by 1H NMR analysis of the crude reaction mixture.
|
1 |
Co(OAc)
|
10 |
THF
|
0 |
25 |
<30 |
67 |
2 |
Mn(OAc)
|
10 |
THF
|
0 |
25 |
<20 |
67 |
3 |
Ni
|
10 |
THF
|
0 |
25 |
75 |
97 |
4 |
Ni
|
10 |
Toluene
|
0 |
25 |
94 |
68 |
5 |
Ni
|
10 |
CH2Cl2 |
0 |
25 |
>95 |
92 |
6 |
Ni
|
10 |
CHCl3 |
0 |
25 |
>95 |
90 |
7 |
Ni
|
10 |
CH3CN
|
0 |
25 |
78 |
78 |
8 |
Ni
|
10 |
AcOEt
|
0 |
25 |
95 |
98 |
9 |
Ni
|
5 |
AcOEt
|
0 |
23 |
91 |
96 |
10 |
Ni
|
2.5 |
AcOEt
|
0 |
48 |
83 |
89 |
11 |
Ni
|
2.5 |
AcOEt
|
rt |
48 |
87 |
92 |
12 |
Ni
|
2.5 |
AcOEt
|
40 |
5 |
>95 |
93 |
The substrate scope and limitations of the reaction are summarized in Table 2.16 Because the reactivity of β-keto esters 2 depends on the structure, the reaction conditions, such as catalyst loading and reaction time, were optimized for each β-keto ester. The best results for each substrate are summarized in Table 2. Indanone-derived β-keto esters 2b–2d showed good reactivity, and products 3b–3d were obtained in 92–90% yield and 98–94% ee after 4.5 to 10 h using 2.5 mol% of Ni2–1 (entries 2, 4–5). In entry 3, the reaction was performed with 1 mol% Ni2–1catalyst, but both the reactivity and enantioselectivity decreased (18 h, 84% yield, 87% ee). The reactivity of β-keto esters 2e and 2f with a six-membered ring was lower than that of β-keto ester 2a, and 10 mol% of Ni2–1 was required to obtain products 3e and 3f in 98–73% yield and 91–75% ee after 24 h (entries 6 and 7). The reactivity of acyclic β-keto esters 2g and 2h was much lower than that of cyclic β-keto esters, and products 3g and 3h were obtained in only 35–51% yield even using 10 mol% of the catalyst (entries 8–9, 82–85% ee). We are speculating that the modest to poor reactivity in entries 7–10 is due to poor nucleophilicity of Ni–enolates. The Ni2–1catalyst was also applicable to N-Boc oxindole 2i, giving product 3i in 99% yield and 80% ee after 24 h. To demonstrate the synthetic utility of the reaction, transformation of the products was performed (Scheme 1). Reduction of 3c with RANEY® Ni under H2 (1 atm) in the presence of Boc2O gave γ2,2-amino ester 4c in 72% yield. Reduction of 3a with Pd/C under H2 (1 atm) in MeOH gave bicyclic amino ester, which was isolated after Boc-protection in 82% yield (5a, 2 steps).
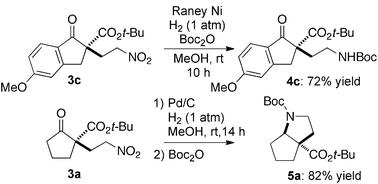 |
| Scheme 1 Transformation of 1,4-adducts. | |
Entry |
2
|
cat. (x mol%) |
Time/h |
% Yieldb |
% eec |
The reactions were run with 1.2 equiv. of nitroethylene (in toluene).
Isolated yield after purification by silica gel column chromatography.
Determined by HPLC analysis.
|
1 |
|
2.5 |
5 |
92 |
93 |
2 |
|
2.5 |
4.5 |
92 |
97 |
3 |
|
1 |
18 |
84 |
87 |
4 |
|
2.5 |
5 |
90 |
98 |
5 |
|
2.5 |
10 |
90 |
94 |
6 |
|
10 |
18 |
98 |
91 |
7 |
|
10 |
24 |
73 |
75 |
8 |
|
10 |
120 |
35 |
85 |
9 |
|
10 |
126 |
51 |
82 |
10 |
|
10 |
24 |
99 |
80 |
In the present reaction, we assume that the two Ni centers function cooperatively as observed in other related reactions using Ni2–1.13 The postulated reaction mechanism is summarized in Fig. 2. One of the Ni–O bonds in the outer O2O2 cavity is speculated to work as a Brønsted base to generate Ni–enolate in situ.17 The other Ni in the inner N2O2 cavity functions as a Lewis acid to control the position of nitroethylene, similar to conventional metal–salen Lewis acid catalysis. The C–C bond-formation via the transition state (TS in Fig. 2), followed by protonation, affords product and regenerates the Ni2–1catalyst.
In summary, we developed a homodinuclear Ni2–Schiff base-catalyzed enantioselective 1,4-addition of β-keto esters to nitroethylene. The reaction proceeded with 1–10 mol% catalyst, and products bearing a quaternary carbon stereocenter adjacent to an ester were obtained in 98–75% ee and 99–35% yield (TON = up to 84). Further studies to improve the poor reactivity for acyclic β-keto esters through ligand modification are ongoing.
This work was supported by Takeda Science Foundation, Inoue Science Research Award from Inoue Science Foundation, and Grant-in-Aid for Young Scientists (A) from JSPS. We thank Prof. M. Shibasaki at Institute of Microbial Chemistry, Tokyo and Prof. M. Kanai at the University of Tokyo for their generous supports, fruitful discussion, and unrestricted access to analytical facilities.
Notes and references
- Recent reviews on catalytic asymmetric construction of quaternary carbon stereocenters:
(a) J. Christoffers and A. Baro, Adv. Synth. Catal., 2005, 347, 1473 CrossRef CAS;
(b) B. M. Trost and C. Jiang, Synthesis, 2006, 369 CrossRef CAS;
(c) P. G. Cozzi, R. Hilgraf and N. Zimmermann, Eur. J. Org. Chem., 2007, 5969 CrossRef CAS.
- General reviews on asymmetric 1,4-addition to electron-deficient alkenes with metal catalysis:
(a) J. Christoffers, G. Koripelly, A. Rosiak and M. Rössle, Synthesis, 2007, 1279 CrossRef CAS; With organocatalysis:
(b) S. B. Tsogoeva, Eur. J. Org. Chem., 2007, 1701 CrossRef CAS.
- A review on asymmetric 1,4-addition to nitroalkenes: O. M. Berner, L. Tedeschi and D. Enders, Eur. J. Org. Chem., 2002, 1877 Search PubMed.
-
(a) Y. Chi, L. Guo, N. A. Kopf and S. H. Gellman, J. Am. Chem. Soc., 2008, 130, 5608 CrossRef CAS;
(b) M. Wiesner, J. D. Revell, S. Tonazzi and H. Wennemers, J. Am. Chem. Soc., 2008, 130, 5610 CrossRef CAS.
- T. Bui, S. Syed and C. F. Barbas, III, J. Am. Chem. Soc., 2009, 131, 8758 CrossRef CAS.
- Reviews on the foldamer research:
(a) C. M. Goodman, S. Choi, S. Shandler and W. F. Degrado, Nat. Chem. Biol., 2007, 3, 252 CrossRef CAS;
(b) D. Seebach, M. Brenner, M. Rueping and B. Jaun, Chem.–Eur. J., 2002, 8, 573 CrossRef CAS;
(c) S. H. Gellman, Acc. Chem. Res., 1998, 31, 173 CrossRef CAS.
- A general review on asymmetric synthesis of γ-amino acids: M. Ordóñez and C. Cativiela, Tetrahedron: Asymmetry, 2007, 18, 3 Search PubMed.
- For diastereoselective 1,4-addition of a chiral enamino ester derived from a cyclic β-keto ester and chiral amine to nitroethylene, see: J. d'Angelo, C. Cavé, D. Desmaële, A. Gassama, C. Thominiaux and C. Riche, Heterocycles, 1998, 47, 725 Search PubMed.
- For alternative highly enantioselective catalytic asymmetric approaches to γ2,2-amino acids with α-quaternary carbon stereocenters via ring-opening reactions of aziridines and cyclic sulfamidates with β-keto esters, see:
(a) T. A. Moss, D. R. Fenwick and D. J. Dixon, J. Am. Chem. Soc., 2008, 130, 10076 CrossRef CAS;
(b) M. W. Paixão, M. Nielsen, C. B. Jacobsen and K. A. Jørgensen, Org. Biomol. Chem., 2008, 6, 3467 RSC;
(c) T. A. Moss, B. Alonso, D. R. Fenwick and D. J. Dixon, Angew. Chem., Int. Ed., 2010, 49, 568 CAS.
- Recent reviews on bifunctional Lewis acid/Brønsted base asymmetric metal catalysis:
(a) S. Matsunaga and M. Shibasaki, Bull. Chem. Soc. Jpn., 2008, 81, 60 CrossRef CAS;
(b) M. Shibasaki, S. Matsunaga and N. Kumagai, Synlett, 2008, 1583 CrossRef CAS.
- For a general review on bimetallic Schiff base catalysts: R. M. Haak, S. J. Wezenberg and A. W. Kleij, Chem. Commun., 2010, 46, 2713 Search PubMed.
- Co2–1catalyst for 1,4-addition of β-keto esters to β-substituted nitroalkenes:
(a) M. Furutachi, Z. Chen, S. Matsunaga and M. Shibasaki, Molecules, 2010, 15, 532 Search PubMed;
(b) Z. Chen, M. Furutachi, Y. Kato, S. Matsunaga and M. Shibasaki, Angew. Chem., Int. Ed., 2009, 48, 2218 CrossRef CAS. Mn2–1catalyst for 1,4-addition of N-Boc oxindoles to β-substituted nitroalkenes:
(c) Y. Kato, M. Furutachi, Z. Chen, H. Mitsunuma, S. Matsunaga and M. Shibasaki, J. Am. Chem. Soc., 2009, 131, 9168 CrossRef CAS.
- Ni2–1catalyst:
(a) Z. Chen, H. Morimoto, S. Matsunaga and M. Shibasaki, J. Am. Chem. Soc., 2008, 130, 2170 CrossRef CAS;
(b) Y. Xu, G. Lu, S. Matsunaga and M. Shibasaki, Angew. Chem., Int. Ed., 2009, 48, 3353 CrossRef CAS;
(c) S. Mouri, Z. Chen, S. Matsunaga and M. Shibasaki, Chem. Commun., 2009, 5138 RSC;
(d) S. Mouri, Z. Chen, H. Mitsunuma, M. Furutachi, S. Matsunaga and M. Shibasaki, J. Am. Chem. Soc., 2010, 132, 1255 CrossRef CAS; For the use of Ni2–1catalyst in 1,4-addition to β-substituted nitroalkenes with other donors:
(e) N. E. Shepherd, H. Tanabe, Y. Xu, S. Matsunaga and M. Shibasaki, J. Am. Chem. Soc., 2010, 132, 3666 CrossRef CAS;
(f) Y. Xu, S. Matsunaga and M. Shibasaki, Org. Lett., 2010, 12, 3246 CrossRef CAS.
- Heterobimetallic transition metal/rare earth metal Schiff base catalysts:
(a) S. Handa, V. Gnanadesikan, S. Matsunaga and M. Shibasaki, J. Am. Chem. Soc., 2007, 129, 4900 CrossRef CAS;
(b) S. Handa, K. Nagawa, Y. Sohtome, S. Matsunaga and M. Shibasaki, Angew. Chem., Int. Ed., 2008, 47, 3230 CrossRef CAS;
(c) H. Mihara, Y. Xu, N. E. Shepherd, S. Matsunaga and M. Shibasaki, J. Am. Chem. Soc., 2009, 131, 8384 CrossRef CAS;
(d) S. Handa, V. Gnanadesikan, S. Matsunaga and M. Shibasaki, J. Am. Chem. Soc., 2010, 132, 4925 CrossRef CAS.
- For selected examples of related bifunctional bimetallic Schiff base complexes in asymmetric catalysis from other groups, see:
(a) V. Annamalai, E. F. DiMauro, P. J. Carroll and M. C. Kozlowski, J. Org. Chem., 2003, 68, 1973 CrossRef CAS and references therein;
(b) M. Yang, C. Zhu, F. Yuan, Y. Huang and Y. Pan, Org. Lett., 2005, 7, 1927 CrossRef CAS;
(c) J. Gao, F. R. Woolley and R. A. Zingaro, Org. Biomol. Chem., 2005, 3, 2126 RSC;
(d) W. Li, S. S. Thakur, S.-W. Chen, C.-K. Shin, R. B. Kawthekar and G.-J. Kim, Tetrahedron Lett., 2006, 47, 3453 CrossRef CAS;
(e) C. Mazet and E. N. Jacobsen, Angew. Chem., Int. Ed., 2008, 47, 1762 CrossRef CAS;
(f) J. Park, K. Lang, K. A. Abboud and S. Hong, J. Am. Chem. Soc., 2008, 130, 16484 CrossRef CAS;
(g) W. Hirahata, R. M. Thomas, E. B. Lobkovsky and G. W. Coates, J. Am. Chem. Soc., 2008, 130, 17658 CrossRef CAS;
(h) B. Wu, J. C. Gallucci, J. R. Parquette and T. V. RajanBabu, Angew. Chem., Int. Ed., 2009, 48, 1126 CrossRef CAS; For related early studies with dinuclear Ni2–Schiff base complexes as epoxidation catalysts, see also:
(i) T. Oda, R. Irie, T. Katsuki and H. Okawa, Synlett, 1992, 641 CrossRef CAS; For other examples, see ref. 11.
- The absolute configuration of 3b was determined by comparing with the reported data in ref. 9c after conversion into known γ2,2-amino ester. See ESI†.
-
1H NMR analysis of the bimetallic Ni2–1 complex does not show any peaks, suggesting that at least one of Ni metal centers has non-planar coordination mode. Based on the molecular model, we assume that the outer Ni center has cis-β configuration due to strain of the bimetallic complex. In other words, one of the Ni–O bonds of the outer Ni center is speculated to be in apical position. Thus, the weak Ni–O bond would work as a Brønsted base
to deprotonate β-keto esters to give the Ni–enolate intermediate. Detailed mechanistic studies to clarify the role of two Ni metal centers are ongoing. For the utility of cis-β metal complexes of salens in asymmetric catalysis, see a review: T. Katsuki, Chem. Soc. Rev., 2004, 33, 437 Search PubMed.
Footnote |
† Electronic supplementary information (ESI) available: General experimental information, spectral data of new compounds, and copies of NMR spectral data. See DOI: 10.1039/c0cc02152k |
|
This journal is © The Royal Society of Chemistry 2011 |
Click here to see how this site uses Cookies. View our privacy policy here.