DOI:
10.1039/C1AN15600D
(Paper)
Analyst, 2012,
137, 133-139
High-performance liquid chromatographic separation of position isomers using metal–organic framework MIL-53(Al) as the stationary phase
Received
15th July 2011
, Accepted 27th September 2011
First published on 28th October 2011
Abstract
Metal–organic framework MIL-53(Al) was explored as the stationary phase for high-performance liquid chromatographic separation of position isomers using a binary and/or polar mobile phase. Baseline separations of xylene, dichlorobenzene, chlorotoluene and nitrophenol isomers were achieved on the slurry-packed MIL-53(Al) column with high resolution and good precision. The effects of mobile phase composition, injected sample mass and temperature were investigated. The separation of xylene, dichlorobenzene, chlorotoluene and nitrophenol isomers on MIL-53(Al) were controlled by entropy change.
1. Introduction
Metal–organic frameworks (MOFs) are a novel class of microporous materials constructed from clusters or chains of metal ions connected by organic linkers.1–4 The large diversity in structures and pore topologies, high surface areas and adsorption affinities make MOFs attractive as advanced media in various fields.5–16 MOFs with sufficiently large pore windows and cages, and in most of them an important fraction of the pore walls consists of aromatic rings which have stimulated their promising applications as stationary phases for chromatography.17–19 However, researches on the applications of MOFs in high-performance liquid chromatography (HPLC) have lagged behind.20–27 Since the first example of MOFs for the HPLC separation of xylene isomers and ethylbenzene (EB) on a dry-packed MIL-47 column using hexane as the mobile phase,21 the selective separation of ortho-alkyl aromatics on MIL-53(Al) columns using hexane as the mobile phase,22 organic compounds based on size and shape selectivity on MOF-5 and HKUST-1,26 EB and styrene on silica–Cu3(BTC)2 composites, MIL-47- and MIL-53-packed columns have been reported.25,27 However, the above-mentioned studies on the utilization of MOFs as the stationary phases for HPLC separation have been performed using a single non-polar or low polar solvent as the mobile phase, even though the composition of the mobile phase can play a significant role in retention, resolution and selectivity.28
To explore the potential of MOFs for wide applications in HPLC, here we report the MIL-53(Al)-packed column using a binary and/or polar mobile phase for high resolution and selective separation of position isomers. MIL-53(Al) consists of AlIII metal ions, terephthalate ligands and OH– groups. The terephthalate ligands point in four directions to form one-dimensional lozenge-shaped pores (8.5 Å) and aromatic ring walls. Its high surface area, aromatic pore walls, excellent chemical and solvent stability make MIL-53(Al) promising as the stationary phase for HPLC.29
EB and xylene, dichlorobenzene, chlorotoluene and nitrophenol position isomers were chosen as the targets because of their significant roles in industry.21–24,30,31 For instance, p-xylene is oxidized to terephthalic acid for poly(ethylene terephthalate) (PET) production; EB is dehydrogenated to styrene for polystyrene production. Dichlorobenzene, chlorotoluene and nitrophenol isomers are also widely used as solvents or intermediates in the production of insecticides or other pesticides. Selective separation of these isomers is of great importance and high value.
In this work, the slurry-packed MIL-53(Al) column was investigated for HPLC separation of xylene and EB, dichlorobenzene, chlorotoluene, and nitrophenol position isomers using a binary mobile phase. The MIL-53(Al) column offered baseline separations of these position isomers with high column efficiency and good precision.
2. Experimental
Reagents
All chemicals and reagents used were at least of analytical grade. Al(NO3)3·9H2O, terephthalic acid, xylene, dichlorobenzene and chlorotoluene isomers were purchased from the Shanghai Aladdin Chemistry Co. Ltd. (Shanghai, China). Ultrapure water (18.2 MΩ cm) was obtained from a WaterPro Water Purification System (Labconco Corp., Kansas City, USA). Ethylbenzene, nitrophenol isomers, hexane, dichloromethane (DCM), and methanol (MeOH) were purchased from Tianjin Guangfu Fine Chemical Research Institute (Tianjin, China).
Synthesis, activation and characterization of MIL-53(Al)
MIL-53(Al) was hydrothermally synthesized according to Loiseau et al.29 Typically, 1300 mg of Al(NO3)3·9H2O and 288 mg of terephthalic acid were mixed with 5 mL of ultrapure water in a Teflon-lined stainless steel autoclave, which was heated at 493 K for 3 days. After cooling down, the white powder obtained was washed with ultrapure water. Then, the solid was dried and activated upon heating in air at 330 °C for 3 days to remove the unreacted terephthalic acid from the pores. Scanning electron microscopy (SEM), X-ray diffraction spectrometry (XRD) and thermal gravimetric analysis (TGA) were employed to characterize the synthesized MIL-53(Al).
SEM images were recorded on a Shimadzu SS-550 scanning electron microscope at 15.0 kV. XRD patterns were recorded with a D/max-2500 diffractometer (Rigaku, Japan) using Cu-Kα radiation (λ = 1.5418 Å). The TGA experiments were performed on a PTC-10A thermal gravimetric analyzer (Rigaku, Japan) from room temperature to 800 °C at a ramp rate of 10 °C min−1. BET surface area was measured on an ASAP 2010 micropore physisorption analyzer (Micromeritics, Norcross, GA) using nitrogen adsorption at 77 K in the range 0.02 ≤ P/P0 ≤ 0.20, respectively.
2.50 g of MIL-53(Al) was dispersed in a vessel with 50 mL DCM under ultrasonication for 5 min. The suspension was then downward packed into a 15-cm long stainless steel column with an internal diameter of 4.6 mm under 6000 psi for 10 min. Stainless steel frits with a pore size of 1 μm were used to keep the stationary phase intact. The MIL-53(Al) packed column was conditioned with DCM at a flow of 1 mL min−1 for 2 h before chromatographic experiments.
HPLC separation and measurement of thermodynamic parameters
The HPLC system consisted of a Waters 510 HPLC pump and a 486 tunable UV detector. Data acquisition and processing were performed on an N2000 chromatography data system. Hexane (99.5%), DCM (99.5%) and MeOH (99.9%) were used to prepare the mobile phases.
To measure the enthalpy change (ΔH) and entropy change (ΔS) for transfer of the solutes from the mobile phase to the stationary phase MIL-53(Al), an Ameritech CO-5060 column heater (±0.1 °C) was used to control the column temperature. The mobile phase was preheated to the same temperature as the column in a water bath during HPLC separation. HPLC experiments were carried out at six different temperatures in the range of 25–37.5 °C. The following van't Hoff equation (eqn (1)) was then used to calculate ΔH and ΔS for transfer of the solutes from the mobile phase to the stationary phase MIL-53(Al):
| 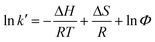 | (1) |
where
k′ is the
retention factor,
R the gas constant, and
T the absolute temperature. The
k′ value was calculated according to
eqn (2):
where
t is the
retention time,
t0 the column void time which was determined by injecting a small plug of
hexane and recording the perturbation signal.
Φ is the phase ratio of the column, which was calculated according to
eqn (3):
where
VS is the volume of the
stationary phase in the column, which was calculated based on
eqn (4).
V0 is the void volume of the column, which was evaluated according to
eqn (5),
where
VCOL is the geometrical volume of the column, and
F the flow rate of the
mobile phase.
3. Results and discussion
Characterization of the synthesized MIL-53(Al)
The SEM image shows the lozenge shaped crystals of MIL-53(Al) (Fig. 1A). The XRD pattern of the synthesized MIL-53(Al) was in good agreement with the simulated one, indicating the successful preparation of MIL-53(Al) (Fig. 1B). The TGA curve reveals that the MIL-53(Al) crystals are stable up to 330 °C (Fig. 1C). The BET surface area of the prepared MIL-53(Al) is 904 m2 g−1.
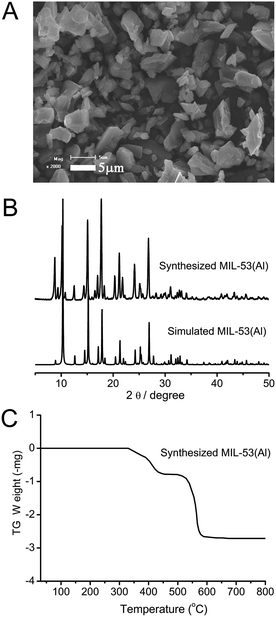 |
| Fig. 1 (A) SEM image of the prepared MIL-53(Al); (B) comparison of the XRD pattern of the prepared MIL-53(Al) with the simulated one; (C) TGA curve of the prepared MIL-53(Al). | |
Effect of the composition of mobile phase
The mobile phase composition significantly affected the HPLC separation of EB and xylene (Fig. 2A). The use of hexane/DCM (90
:
10) as the mobile phase gave poor resolution and a long retention time for the separation of p-xylene and m-xylene (Fig. 2A). As the content of DCM in the binary hexane/DCM mobile phase increased, the retention time of the analytes decreased accompanied by improved resolution and separation efficiency, as a consequence of an increasing competition from solvent adsorption via van der Waals forces.24 The mobile phase of hexane/DCM (60
:
40) led to a rapid and high-resolution separation of the xylene and EB isomers on the MIL-53(Al) packed column.
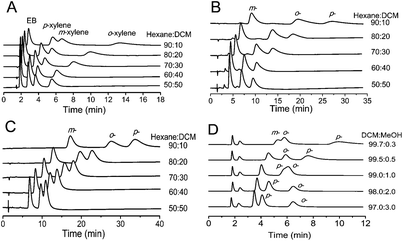 |
| Fig. 2 HPLC chromatograms for the separation of: (A) EB, p-, m-, and o-xylene (5 μL, 1 g L−1 each); (B) m-, o-, and p-dichlorobenzene (5 μL, 1 g L−1 each); (C) m-, o-, and p-chlorotoluene (5 μL, 1 g L−1 each); (D) m-, o-, and p-nitrophenol (5 μL, 1 g L−1 each) on MIL-53(Al) packed column (15-cm long × 4.6-mm i.d.) using different ratios of hexane/DCM and DCM/MeOH as the mobile phase at a flow rate of 1 mL min−1 at room temperature. The signals were monitored with a UV detector at 254 nm. | |
The mobile phase composition also affected the HPLC separation of dichlorobenzene and chlorotoluene isomers (Fig. 2B and 2C). Baseline separations of dichlorobenzene and chlorotoluene isomers were achieved by using hexane/DCM (90
:
10) as the mobile phase. As the content of DCM in the binary hexane/DCM mobile phase increased, the retention time decreased accompanied by an improved separation efficiency for dichlorobenzene isomers, but with the decreasing of resolution and column efficiency for chlorotoluene isomers. Thus, hexane/DCM (50
:
50) and hexane/DCM (90
:
10) were used as the mobile phase for the separation of dichlorobenzene and chlorotoluene isomers.
To get further insight into the effect of the composition of the mobile phase on the separation of nitrophenol isomers on the MIL-53(Al) packed column, the content of MeOH in the DCM/MeOH mobile phase was varied. The increase of the polarity of the mobile phase significantly reduced the retention of m-nitrophenol and p-nitrophenol, but slightly increased the retention of o-nitrophenol on the MIL-53(Al) column (Fig. 2D). m-Nitrophenol always showed the weakest retention regardless of the content of MeOH in the mobile phase. o-Nitrophenol eluted before p-nitrophenol with the DCM/MeOH containing less than 1.0% (v/v) MeOH as the mobile phase, showing higher selectivity for p-nitrophenol. However, the elution order of o-nitrophenol and p-nitrophenol was reversed with greater than 1.0% (v/v) MeOH contained within DCM/MeOH as the mobile phase. Baseline separation of nitrophenol isomers was achieved on the MIL-53(Al) column either with 0.5% or 2.0–3.0% (v/v) MeOH contained within DCM/MeOH as the mobile phase.
The elution for xylene isomers followed an increasing order of the p- < m- < o-isomer. The high preference for o-xylene was attributed to the molecular geometry of o-xylene which allows the interaction of both methyl groups with the carboxylate groups of the frameworks resulting in greater retention on MIL-53(Al). The m- and p-xylene molecules can only interact with the carboxylate groups via one methyl group simultaneously, and thus eluted faster than o-xylene.22 The easiest elution of p-xylene likely resulted from its lowest dipole moment compared to the other two xylene isomers. The elution sequence of dichlorobenzene and chlorotoluene isomers followed an increasing order of m- < o- < p-isomer (Fig. 2B and 2C). The faster elution of the m-isomer than the other two isomers can be attributed to the size exclusion of the larger m-isomers.24 The favorable affinity of MIL-53(Al) for the p-isomers of dichlorobenzene and chlorotoluene resulted from the molecular packing effect, as reported for the easier and more effective adsorption of p-dichlorobenzene inside the MIL-53(Al) pores than the other isomers.24 A similar molecular packing effect was also observed on the preferable adsorption of the p-isomer of xylene on MIL-47.21
The retention of the analytes on MIL-53(Al) also depended on the nature of the substituent on the benzene ring. Chlorine-substituted isomers (e.g. dichlorobenzene and chlorotoluene) were more strongly retained on the MIL-53(Al)-packed column than methyl-substituted isomers (e.g. xylene) (Fig. 2A–C), indicating the stronger interaction between the chlorine-substituted isomers and MIL-53(Al) as a result of the polar character of the MIL-53(Al) pores, in which hydroxyl ligands are protruding from the pore corners, enhancing the uptake of polar adsorbates.24 The nitryl- and hydroxyl-substituted nitrophenol isomers showed the strongest retention on the MIL-53(Al) column, probably due to the hydrogen bonding interactions between the nitrophenol isomers and the hydroxyl ligands in the MIL-53(Al) frameworks. Therefore, MeOH should be included in the mobile phase as the polar additive for the baseline separation of nitrophenol isomers.
The separation of nitrophenol isomers was probably controlled by the hydrogen bonding interaction between the hydroxy groups in the MIL-53(Al) framework and nitrophenol isomers.30,31 The relative position of the hydroxyl and nitryl groups on the benzene ring in the three isomers results in different dissociation abilities of the hydroxyl group (different acidity), and m-nitrophenol shows the weakest acidity among the three isomers because of the lack of resonance effect in the meta position compared with the ortho and para positions.32 Therefore, the hydrogen bonding interaction between m-nitrophenol and the hydroxy ligands in MIL-53(Al) should be the weakest among the three isomers, accounting for the fastest elution of m-nitrophenol. The MeOH in the mobile phase should compete for the hydrogen bonding interaction sites. An increase of the content of MeOH in the mobile phase not only inhibited the hydrogen bonding interaction of m- and p-nitrophenol isomers with MIL-53(Al), but also enhanced their solubility in the mobile phase, thereby weakening the retention of these isomers due to MeOH competition for hydrogen bonding with MIL-53(Al) and these isomers. However, the retention of o-nitrophenol even increased with the content of MeOH in the mobile phase because of its decreased solubility in the mobile phase resulting from the intramolecular hydrogen bonding of o-nitrophenol and its lowest dipole moment (3.74 D) among the three isomers.30,31 The opposite effects of MeOH on the retention of o-nitrophenol and the other two isomers make the separation of these position isomers easy on the MIL-53(Al) packed column by controlling the content of MeOH in the mobile phase. The above results indicated that the mobile phase played significant roles in the high-performance separation of the position isomers on MIL-53(Al).
Performance of MIL-53(Al) packed column for the HPLC separation of position isomers
The MIL-53(Al) packed column gave good reproducibility (Fig. 3, Table 1) and high selectivity (Table 2 and Table 3) for the HPLC separation of EB and xylene, dichlorobenzene, chlorotoluene, and nitrophenol isomers. The relative standard deviations (RSDs) for nine replicate separations of EB and xylene, dichlorobenzene, chlorotoluene, and nitrophenol isomers are 0.1–0.4%, 1.1–2.9%, 0.5–2.7% and 0.6–1.8% for the retention time (t), peak area, peak height and half peak width (W1/2), respectively (Fig. 3 and Table 1). In addition, the MIL-53(Al) column offered a column efficiency of 10
200 plates m−1 for EB. The asymmetrical peaks and the band broadening likely resulted from the large particle size distribution and the irregular shape of the MIL-53(Al) particles (Fig. 1A).
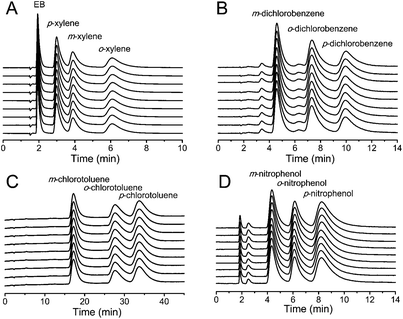 |
| Fig. 3 HPLC chromatograms on a slurry-packed MIL-53(Al) column (15-cm long × 4.6 mm i.d.) for nine replicate separations of: (A) EB, p-, m-, and o-xylene (5 μL, 1 g L−1 each) using hexane/DCM (60 : 40) as the mobile phase; (B) m-, o-, and p-dichlorobenzene (5 μL, 1 g L−1 each) using hexane/DCM (50 : 50) as the mobile phase; (C) m-, o-, and p-chlorotoluene (5 μL, 1 g L−1 each) using hexane/DCM (90 : 10) as the mobile phase; (D) m-, o-, and p-nitrophenol (5 μL, 1 g L−1 each) using DCM/MeOH (99.5 : 0.5) as the mobile phase. All the separations were performed at a flow rate of 1 mL min−1 and monitored with a UV detector at 254 nm at room temperature. | |
Table 1 Precision for nine replicate separations of position isomers on the MIL-53(Al) packed column
Analytes |
RSD (%) (n = 9) |
t
|
Peak area |
Peak height |
W
1/2
|
EB |
0.1 |
1.1 |
1.3 |
0.9 |
p-Xylene |
0.1 |
2.6 |
1.2 |
0.9 |
m-Xylene |
0.2 |
1.7 |
2.4 |
1.3 |
o-Xylene |
0.2 |
2.8 |
1.1 |
1.3 |
m-Dichlorobenzene |
0.1 |
1.7 |
0.5 |
0.8 |
o-Dichlorobenzene |
0.2 |
1.9 |
1.7 |
1.8 |
p-Dichlorobenzene |
0.3 |
1.7 |
2.7 |
1.7 |
m-Chlorotoluene |
0.3 |
2.1 |
0.9 |
0.9 |
o-Chlorotoluene |
0.2 |
1.3 |
1.2 |
0.6 |
p-Chlorotoluene |
0.3 |
2.8 |
1.9 |
1.3 |
m-Nitrophenol |
0.3 |
2.4 |
1.9 |
0.6 |
o-Nitrophenol |
0.4 |
2.3 |
1.6 |
1.1 |
p-Nitrophenol |
0.3 |
2.9 |
2.3 |
0.9 |
Analytes mass/μg |
Selectivity (α) |
EB and xylene |
Dichlorobenzene |
Chlorotoluene |
Nitrophenol |
p/EB |
m/p |
o/p |
o/m |
p/o |
o/m |
p/o |
o/m |
p/o |
2.5 |
3.15 |
1.54 |
1.94 |
2.08 |
1.45 |
1.75 |
1.13 |
1.64 |
1.49 |
5.0 |
3.15 |
1.54 |
1.94 |
2.08 |
1.45 |
1.74 |
1.13 |
1.67 |
1.47 |
7.5 |
3.15 |
1.54 |
1.94 |
2.08 |
1.45 |
1.75 |
1.13 |
1.68 |
1.45 |
10.0 |
3.15 |
1.54 |
1.94 |
2.08 |
1.45 |
1.75 |
1.13 |
1.69 |
1.44 |
15.0 |
3.15 |
1.54 |
1.94 |
2.08 |
1.45 |
1.75 |
1.13 |
1.71 |
1.43 |
20.0 |
3.15 |
1.54 |
1.94 |
2.08 |
1.46 |
1.75 |
1.13 |
1.72 |
1.42 |
T/°C |
Selectivity (α) |
EB and xylene |
Dichlorobenzene |
Chlorotoluene |
Nitrophenol |
p/EB |
m/p |
o/m |
o/m |
p/o |
o/m |
p/o |
o/m |
p/o |
25.0 |
3.29 |
1.52 |
1.93 |
1.98 |
1.50 |
1.69 |
1.18 |
1.61 |
1.69 |
27.5 |
3.26 |
1.58 |
1.94 |
1.98 |
1.49 |
1.69 |
1.17 |
1.62 |
1.69 |
30.0 |
3.25 |
1.54 |
1.96 |
1.99 |
1.49 |
1.71 |
1.18 |
1.62 |
1.70 |
32.5 |
3.26 |
1.52 |
1.99 |
2.02 |
1.48 |
1.72 |
1.16 |
1.61 |
1.69 |
35.0 |
3.22 |
1.49 |
1.97 |
2.02 |
1.47 |
1.70 |
1.15 |
1.61 |
1.71 |
37.5 |
3.24 |
1.49 |
1.99 |
2.03 |
1.48 |
1.71 |
1.14 |
1.61 |
1.72 |
The chromatographic peak area and peak height of the analytes linearly increased as the analyte mass increased (Fig. 4 and 5). The retention time and selectivity for EB and xylene, dichlorobenzene and chlorotoluene isomers did not depend on the analyte mass though the increase in analyte mass resulted in a slight decrease of the retention and selectivity for p-nitrophenol, even a slight increase in the selectivity for o-nitrophenol (Fig. 4, Table 2). No significant effect of the analyte mass on the column efficiency of EB, xylene isomers, and nitrophenol isomers was observed. However, the column efficiency of dichlorobenzene and chlorotoluene isomers generally decreased as the analyte mass increased (Fig. 6).
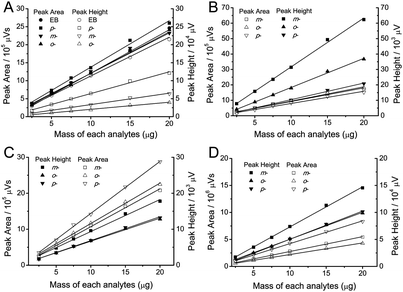 |
| Fig. 5 Effects of injected mass on the peak area and peak height: (A) EB and xylene; (B) dichlorobenzene; (C) chlorotoluene; (D) nitrophenol isomers. Separation conditions as shown in Fig. 4. | |
Thermodynamics of the HPLC separation of position isomers on MIL-53(Al) packed column
The separation of EB and xylene, dichlorobenzene, chlorotoluene and nitrophenol isomers was performed in the temperature range of 25.0–37.5 °C to investigate the thermodynamics of the separation on the MIL-53(Al) packed column. As the column temperature increased, the retention of the analytes substantially decreased on the MIL-53(Al) packed column (Fig. 7), indicating that the separation processes of these position isomers on MIL-53(Al) were exothermic. Even though there is a significant decrease in the analysis time, the selectivity of the position isomers only changed slightly (Table 4).
Analytes |
ΔH/kJ mol−1 |
ΔS/J mol−1 k−1 |
R
2
|
EB |
−6.8 ± 0.4 |
−37.8 ± 2.6 |
0.987 |
p-Xylene |
−11.1 ± 0.9 |
−42.3 ± 2.2 |
0.985 |
m-Xylene |
−11.6 ± 0.2 |
−42.5 ± 2.5 |
0.998 |
o-Xylene |
−11.6 ± 0.6 |
−34.6 ± 1.6 |
0.985 |
m-Dichlorobenzene |
−10.9 ± 0.8 |
−40.7 ± 2.9 |
0.992 |
o-Dichlorobenzene |
−10.9 ± 0.6 |
−38.9 ± 3.4 |
0.989 |
p-Dichlorobenzene |
−10.7 ± 0.7 |
−37.5 ± 1.6 |
0.987 |
m-Chlorotoluene |
−21.1 ± 0.7 |
−57.7 ± 2.9 |
0.994 |
o-Chlorotoluene |
−20.9 ± 0.4 |
−57.4 ± 2.6 |
0.997 |
p-Chlorotoluene |
−21.8 ± 0.8 |
−50.1 ± 2.9 |
0.993 |
m-Nitrophenol |
−5.9 ± 0.3 |
−21.0 ± 2.9 |
0.988 |
o-Nitrophenol |
−6.2 ± 0.5 |
−18.1 ± 3.4 |
0.988 |
p-Nitrophenol |
−6.1 ± 0.2 |
−13.7 ± 2.2 |
0.989 |
The temperature dependence of the retention factor k′ can be described by the van't Hoff equation (see eqn (1)).33 The interaction mechanism in relation to temperature in the studied temperature range did not change because the van't Hoff plots for the separation of xylene, dichlorobenzene, chlorotoluene isomers, and nitrophenol isomers showed good linearity (Fig. 8). The values of ΔH and ΔS obtained from the van't Hoff plots are summarized in Table 4. In general, the transfer of the solute from the mobile phase to the stationary phase was controlled by negative ΔH and ΔS. The highly selective separation of o-xylene, p-dichlorobenzene, p-chlorotoluene and p-nitrophenol on MIL-53(Al) was controlled by ΔS because these isomers gave comparable values of ΔH and the least negative values of ΔS in comparison with their corresponding other two isomers.
Conclusions
In summary, we have reported the feasibility of the slurry-packed MIL-53(Al) column for the HPLC separation of EB and xylene, dichlorobenzene, chlorotoluene and nitrophenol isomers using a binary mobile phase with high selectivity and precision. The mobile phase composition has a significant effect on the retention, resolution, and even the selectivity of these position isomers on MIL-53(Al). Baseline separation of these position isomers can be easily achieved by controlling the mobile phase composition. MOFs are very promising as novel stationary phases for HPLC. The high porosity, large specific surface area and high thermal stability make MOFs a good alternative stationary phase material that can bring out benefits in four-fold: allow high flow rates with low backpressure, high resolution and miniaturization of HPLC columns, and high temperature HPLC.
Acknowledgements
This work was supported by the National Natural Science Foundation of China (Nos 20935001, 21077057), and the Tianjin Natural Science Foundation (No. 10JCZDJC16300).
References
- H. Li, M. Eddaoudi, M. O'Keeffe and O. M. Yaghi, Nature, 1999, 402, 276–279 CrossRef CAS.
- M. Eddaoudi, D. B. Moler, H. L. Li, B. L. Chen, T. M. Reineke, M. O'Keeffe and O. M. Yaghi, Acc. Chem. Res., 2001, 34, 319–330 CrossRef CAS.
- G. Férey, C. Mellot-Draznieks, C. Serre and F. Millange, Acc. Chem. Res., 2005, 38, 217–225 CrossRef.
- G. Férey, C. Mellot-Draznieks, C. Serre, F. Millange, J. Dutour, S. Surble and I. Margiolaki, Science, 2005, 309, 2040–2042 CrossRef.
- L. J. Murray, M. Dinca and J. R. Long, Chem. Soc. Rev., 2009, 38, 1294–1314 RSC.
- M. Latroche, S. Surble, C. Serre, C. Mellot-Draznieks, P. L. Llewellyn, J. H. Lee, J. S. Chang, S. H. Jhung and G. Férey, Angew. Chem., Int. Ed., 2006, 45, 8227–8231 CrossRef CAS.
- J.-R. Li, R. J. Kuppler and H.-C. Zhou, Chem. Soc. Rev., 2009, 38, 1477–1504 RSC.
- L. Hamon, P. L. Llewellyn, T. Devic, A. Ghoufi, G. Clet, V. Guillerm, G. D. Pirngruber, G. Maurin, C. Serre, G. Driver, W. van Beek, E. Jolimaitre, A. Vimont, M. Daturi and G. Férey, J. Am. Chem. Soc., 2009, 131, 17490–17499 CrossRef CAS.
- B. Chen, S. Xiang and G. Qian, Acc. Chem. Res., 2010, 43, 1115–1124 CrossRef CAS.
- Z.-Y. Gu, Y.-J. Chen, J.-Q. Jiang and X.-P. Yan, Chem. Commun., 2011, 47, 4787–4789 RSC.
- X.-Y. Cui, Z.-Y. Gu, D.-Q. Jiang, Y. Li, H.-F. Wang and X.-P. Yan, Anal. Chem., 2009, 81, 9771–9777 CrossRef CAS.
- Z.-Y. Gu, G. Wang and X.-P. Yan, Anal. Chem., 2010, 82, 1365–1370 CrossRef CAS.
- J. Y. Lee, O. K. Farha, J. Roberts, K. A. Scheidt, S. T. Nguyen and J. T. Hupp, Chem. Soc. Rev., 2009, 38, 1450–1459 RSC.
- L. Ma, C. Abney and W. Lin, Chem. Soc. Rev., 2009, 38, 1248–1256 RSC.
- K. M. L. Taylor-Pashow, J. Della Rocca, Z. G. Xie, S. Tran and W. B. Lin, J. Am. Chem. Soc., 2009, 131, 14261–14263 CrossRef CAS.
- P. Horcajada, C. Serre, G. Maurin, N. A. Ramsahye, F. Balas, M. Vallet-Regi, M. Sebban, F. Taulelle and G. Férey, J. Am. Chem. Soc., 2008, 130, 6774–6780 CrossRef CAS.
- B. L. Chen, C. D. Liang, J. Yang, D. S. Contreras, Y. L. Clancy, E. B. Lobkovsky, O. M. Yaghi and S. Dai, Angew. Chem., Int. Ed., 2006, 45, 1390–1393 CrossRef CAS.
- Z.-Y. Gu and X.-P. Yan, Angew. Chem., Int. Ed., 2010, 49, 1477–1480 CrossRef CAS.
- N. Chang, Z.-Y. Gu and X.-P. Yan, J. Am. Chem. Soc., 2010, 132, 13645–13647 CrossRef CAS.
- K. A. Cychosz, R. Ahmad and A. J. Matzger, Chem. Sci., 2010, 1, 293–302 RSC.
- L. Alaerts, C. E. A. Kirschhock, M. Maes, M. A. van der Veen, V. Finsy, A. Depla, J. A. Martens, G. V. Baron, P. A. Jacobs, J. E. M. Denayer and D. E. De Vos, Angew. Chem., Int. Ed., 2007, 46, 4293–4297 CrossRef CAS.
- L. Alaerts, M. Maes, L. Giebeler, P. A. Jacobs, J. A. Martens, J. F. M. Denayer, C. E. A. Kirschhock and D. E. De Vos, J. Am. Chem. Soc., 2008, 130, 14170–14178 CrossRef CAS.
- L. Alaerts, M. Maes, P. A. Jacobs, J. F. M. Denayer and D. E. De Vos, Phys. Chem. Chem. Phys., 2008, 10, 2979–2985 RSC.
- L. Alaerts, M. Maes, M. A. van der Veen, P. A. Jacobs and D. E. De Vos, Phys. Chem. Chem. Phys., 2009, 11, 2903–2911 RSC.
- M. Maes, F. Vermoortele, L. Alaerts, S. Couck, C. E. A. Kirschhock, J. F. M. Denayer and D. E. De Vos, J. Am. Chem. Soc., 2010, 132, 15277–15285 CrossRef CAS.
- R. Ahmad, A. G. Wong-Foy and A. J. Matzger, Langmuir, 2009, 25, 11977–11979 CrossRef CAS.
- R. Ameloot, A. Liekens, L. Alaerts, M. Maes, A. Galarneau, B. Coq, G. Desmet, B. F. Sels, J. F. M. Denayer and D. E. De Vos, Eur. J. Inorg. Chem., 2010, 3735–3738 CrossRef.
- C.-X. Yang and X.-P. Yan, Anal. Chem., 2011, 83, 7144–7150 CrossRef CAS.
- T. Loiseau, C. Serre, C. Huguenard, G. Fink, F. Taulelle, M. Henry, T. Bataille and G. Férey, Chem.–Eur. J., 2004, 10, 1373–1382 CrossRef CAS.
- D. J. Brookman and D. T. Sawyer, Anal. Chem., 1968, 40, 106–110 CrossRef CAS.
- B. Koubaissy, G. Joly and P. Magnoux, Ind. Eng. Chem. Res., 2008, 47, 9558–9565 CrossRef CAS.
- X.-D. Huang, L. Kong, X. Li, C.-J. Zheng and H.-F. Zou, J. Mol. Recognit., 2003, 16, 406–411 CrossRef CAS.
- M. Sliwka-Kaszynska, K. Jaszczolt, D. Witt and J. Rachon, J. Chromatogr., A, 2004, 1055, 21–28 CrossRef CAS.
|
This journal is © The Royal Society of Chemistry 2012 |
Click here to see how this site uses Cookies. View our privacy policy here.