DOI:
10.1039/C1AY05581J
(Paper)
Anal. Methods, 2012,
4, 177-182
A novel high selectivity sensor for tetradifon residues based on double-side hollow molecularly imprinted materials
Received
13th September 2011
, Accepted 2nd November 2011
First published on 6th December 2011
Abstract
A sensitive and rapid technique for high selectivity detection of tetradifon has been developed. This method is based on a new kind of hollow shell molecularly imprinted (MI) polymer. In this method, the new hollow shell MI polymer has bigger specific surface areas compared with the traditional one based on the double-sides hollow microspheres structure. The proposed method has the advantage of wider linear range and lower limit of detection compared with the traditional one. The change in chemiluminescence (CL) intensity is linearly proportional with the concentration of tetradifon in the range 0.1∼12 μg mL−1 and the detection limit has reached 0.0483 μg mL−1. The new MI-CL sensor has been successfully applied to the determination of residual tetradifon in food and the results obtained compare well with those by other methods.
1. Introduction
At present, large scale use of pesticides is among a variety of main factors which contribute to high crop yields in intensive agriculture, for example paraquat in potatoes; fenamiphos in oranges; carbendazim and benomyl in apples. However, a number of pesticides remain on the surface of the crop which can seriously harm human health. Moreover, the residual pesticides have caused environmental contamination. As a result, the occurrence and significance of bound pesticide residues in food have become critical in dealing with the persistence. Tetradifon (4-chlorophenyl 2,4,5-trichlorophenyl sulfone), one of the most potent organochlorine insecticides, can penetrate plant tissue and exhibit contact action on eggs and larvae of many pests, for example, Brevipalpus, Tetranychus, Bryobia, Panonychus and other species of tetranychid mites, including the resistant strains. So it is widely used to resist pests in agriculture. But due to its persistence, it is important to establish a simple and sensitive method to monitor tetradifon in food. Some methods, such as gas chromatography, high performance liquid chromatography, gas chromatography mass spectrometry, thin-layer chromatography and capillary electrophoresis have been reported for the determination of residual tetradifon. But these methods are time-consuming and require complicated pretreatment and expensive instruments.1–3 Since the appearance of highly sensitive photomultipliers (PMT) in the 1950s, which is a simple instrument with low detection limit, large calibration ranges and rapid analysis speed, chemiluminescence (CL) has become one of the most sensitive detection methods,4 and has been widely used in practical applications during the last 50 years.5–13 However, traditional CL suffers from poor selectivity and in some circumstances a screening agent has to be used to erase interference from coexisting substances. Thus, it is important to develop the CL technology to detect tetradifon residues in the environment. Meanwhile, flow-injection chemiluminescence (FI-CL) is known to be a powerful analytical technique that promises high sensitivity, a wide dynamic concentration range, simple instrumentation, and reproducible means of detection, and has been applied successfully to the determination of many kinds of environmental pollutants.14–16
In recent years, molecular imprinting first put forward by Wulff and Mosbach has attracted considerable interest in many areas of chemistry,17,18 biochemistry,19–21 and biotechnology.22,23 Molecularly imprinted polymers (MIPs) are synthetic porous materials, which are selectively capable of rebinding a target analyte or a group of structurally related compounds based on a combination of recognition mechanisms including size, shape, and functionality. The advantageous properties, including the achievable specific affinity, the relative ease of preparation, and their mechanical and chemical robustness, provide a general and versatile means to generate specific binding sites in polymer matrices.24 In our work, the new tetradifon-imprinting hollow shell microspheres (TIHSMs) were successfully prepared by a surface-modified template strategy. The new microspheres possess highly dense imprinting binding sites full of internal and external surfaces. Morphology of TIHSMs was recorded using scanning electron microscopy. The new mesoporous microsphere has bigger specific surface area compared with the traditional one because of the double-sides hollow microsphere structure. Then, a new, highly sensitive flow injection-chemiluminescence-molecular imprinting (FI-CL-MI) sensor based on luminol-hydrogen peroxide-sodium hydroxide system is proposed for the quantification of tetradifon. This new sensor offers the advantages of simplicity of apparatus, less reagent consumption, high sensitivity and sampling efficiency, a wider linear range, slightly lower detection limit, and ease of handling.25 The proposed method was applied to the determination of tetradifon in samples with satisfactory results.
2. Experimental
2.1 Reagents
Methacrylic acid (MAA) and 2,2-azobis (2-methylpropinitrile) (AIBN) were purchased from Tian Jin Da Mao Chemical Reagent Factory (Tian Jin, China). Ethylene glycol dimethacrylate (EGDMA), 3-aminopropyltriethoxysilane (APTS) and luminol were purchased from Alfa Aesar. Toluene, methanol, acetonitrile, and ethanol were purchased from Fu Yu Fine Chemical Co., Ltd. (China). Acryloyl chloride was purchased from Ding Sheng Fine Chemical Co., Ltd. (China). Acetic acid was purchased from Kant Chemical Co., Ltd. Lai Yang (Lai Yang, China). Tetradifon was purchased from Lü Zhou Chemindustry Co., Ltd, (Shan Dong, China). Molecular sieve was purchased from Sinpharm Chemical Reagent Co., Ltd (China). All other chemicals were analytical reagent grade. Double distilled water was used throughout the experiments.
2.2 Apparatus
The IFFM-E flow injection CL analyzer (Xi'an Remex Electronic Instrument High-Tech Ltd, China) was hooked up with an automatic injection system and a detection system. The flow cell was a “Y” shape polymethyl methacrylate column (2.5 mm i.d. ×15 mm length, Fig. 2D) that was positioned in front of the detection window of the photomultiplier tube (PMT). UV absorption spectra were measured with UV-3101 spectrometer (SHIMADZU, Japan). The SEM images were shot by scanning electronic microscope (HITACHI, Japan).
2.3 Synthesis of the double-sides hollow tetradifon-imprinted microsphere
In the research reported here, Fig. 1 illustrates the major steps involved as follows:
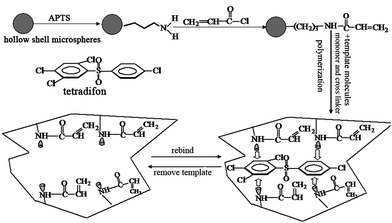 |
| Fig. 1 The structure of tetradifon and the molecular imprinting process based on hollow shell microspheres. | |
2.3.1 Chemical modification of hollow silica mesoporous microsphere (HSMM).
The HSMMs were synthesized according to ref. 26 and then the monodisperse HSMMs were chemically modified using a two-step procedure to obtain the acrylamide-monomer-capping hollow silica mesoporous microsphere. First, aminopropyl modification of hollow silica mesoporous microsphere was carried out with 3-aminopropyltriethoxysilane (APTS). Typically, 0.3 g of HSMMs and 7 ml of APTS were added into anhydrous toluene to make 50 ml of mixture solution. The mixture was refluxed for 12 h under dry nitrogen. In this process, the aminopropyl modification of hollow silica mesoporous microsphere was achieved by attaching aminopropyl to hollow silica mesoporous microsphere. The resulting aminopropyl-modified HSMMs were separated by centrifuge, washed with toluene, and redispersed in fresh anhydrous toluene. Then, the amino end groups of APTS monolayer were further acryloylated with acryloyl chloride (CH2
CHCOCl). 7 mL of acryloyl chloride was mixed with 50 mL of aminopropyl-modified HSMMs toluene solution, and anhydrous potassium carbonate was added into this reaction system as a catalyst. The mixture was vigorously stirred for 12 h at room temperature under dry nitrogen. The product was separated by centrifuge and washed with toluene, water, and ethanol, in that order. Finally, the AA-APTS-HSMMs were obtained.
2.3.2 The synthesis of TIHSMs.
The tetradifon-imprinting hollow shell microspheres were prepared using the following method: the obtained AA-APTS-HSMMs (20 mg) were dispersed in 50 ml acetonitrile in a one-necked flask by ultrasonic vibration, followed by the addition of acylamide (17 mg, 0.24 mmol), EGDMA (183 mg, 0.92 mmol), AIBN (10 mg), and tetradifon (30 mg). The mixing solution was purged with nitrogen for 10 min and sealed under vacuum while being cooled in an ice bath. A two-step-temperature polymerization reaction was carried out on constant-temperature bath oscillators. The prepolymerization was first done at 50 °C for 6 h, and the final polymerization was completed at 60 °C for 24 h and the resulting products were further aged at 85 °C for 6 h obtaining a high cross-linking density. The resultant TIHSMs were separated from the mixed solution by centrifugation, and then washed with acetonitrile and ethanol. At last the TIHSMs were obtained by centrifugation. The non-imprinted microspheres were prepared and treated in a similar way, except that the template molecule was omitted from the polymerization stage.
2.3.3 Elution of the TIHSMs.
The resulting TIHSMs were dried in a high vacuum. The TIHSMs were eluted by mixing CH3OH/HAC solvent (9
:
1, v/v) to remove the tetradifon templates. The UV-visible absorbance spectra of the mixing solution were measured with UV spectrophotometry at the best absorption wavelength of tetradifon. The old solution was replaced by the new one every 3 h until there was no ultraviolet absorption which demonstrated the tetradifon templates had been eluted completely. Finally, the microspheres were dried at 50 °C in vacuum.
2.3.4 Preparation of the TIHSM column.
A novel flow path of FI-CL (Fig. 2) was designed and used instead of the traditional one. In order to obtain a Y-shaped flow path, a PMMA module was drilled through, and three branches were signed as “A”, “B” and “C”, respectively. Through the Y-shaped flow path, three reactants were injected simultaneously. The “A” tube was plugged by 10.0 mg TIHSMs, and then plugged with a small quantity of glass wool at both ends. This new TIHSMs column was positioned in front of the detection window of the photomultiplier tube. It was used as recognition part of the CL sensor. The tetradifon solution was delivered through the TIHSMs and tetradifon in sample solution was then selectively adsorbed on the column by specific recognition. Subsequently the merged stream of luminal, hydrogen peroxide and sodium hydroxide flowed through the newly made TIHSMs column and reacted with the adsorbed tetradifon to produce CL. During this procedure the affinity binding between TIHSMs and tetradifon was destroyed and the cavities of binding sites were left empty on the TIHSMs column when the CL blank signal had become stable, water carrier was pumped through the column to clean the TIHSMs, preparing for the next concentration determination.
2.3.5 Process of detection.
The flow path of the determination of tetradifon is described in Fig. 2.
The experimental procedure includes four parts.
Step 1: Recognition and the adsorption of tetradifon
In this step, pump 2 was stopped. The tetradifon solution was delivered through the TIHSMs column by pump 1. Tetradifon in sample solution was selectively adsorbed on the column by specific recognition.
Step 2: Removal of other substances except tetradifon
In this step, the pump 2 was still stopped. The TIHSMs column was washed with methanol, which was delivered by the pump 1 to remove the impurities only leaving tetradifon in the recognition cavities.
Step 3: Chemiluminescence detection
In this step, pump 1 was stopped; the luminal, hydrogen peroxide and sodium hydroxide were simultaneously delivered through the TIHSMs column by pump 2 and reacted with the tetradifon in the hollow silica mesoporous microsphere's cavity to produce CL signal.
Step 4: Cleaning the TIHSMs column
In this step, after measurements of tetradifon, pump 1 was stopped. The methanol was delivered by pump 2 through TIHSMs column to clean the reaction products in the TIHSM column for the next determination.
3. Results and discussion
3.1 Characterization of TIHSMs
Fig. 3 presents the morphology of the resulting tetradifon imprinted (b, c, d) and non-imprinted (a) polymer synthesized with MAA as the functional monomer. We can see compact and much more porous morphology of the imprinted polymers. The non-imprinted polymer (a) had very small surface globules and is slicker than the imprinted polymer.
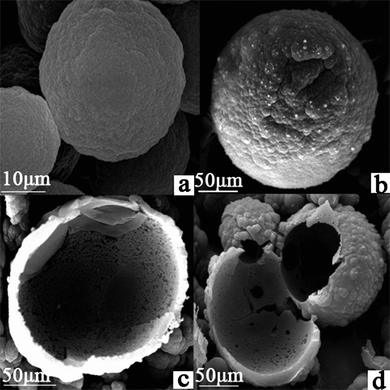 |
| Fig. 3 Higher magnification SEM images of TIHSMs. | |
The SEM micrographs of sample b, c, d (Fig. 3) indicated that lots of hollows are observed. After hydrofluoric acid treatment for removing the original hollow silica mesoporous microspheres, the shell morphology still keeps intact. The inside surface of the TIHSMs was exposed, specific surface area was enlarged greatly. Thus, the amount of binding sites has been increased remarkably due to the extremely high surface to volume ratio. Hollow-type structure with pores in shell could create microflow between the space of inside and outside of the microsphere through pores; this could be very helpful for tetradifon molecules to approach the inside and outside surface of the microsphere. This could increase the binding efficiency remarkably.
3.2 Characterization of chemical modification of HSMMs
Here, we demonstrate that the organic modifying layer at HSMMs is very stable and of high productivity, ensuring that the imprinting polymerization selectively occurs at the surface of HSMMs and tetradifon templates can be sufficiently assembled into the shell of core-shell microspheres. Fig. 4 shows the infrared spectra of pure silicaFig. 4a, APTS-silica Fig. 4b, and AA-APTS-silica microspheres Fig. 4c. Compared with the infrared date of pure silica, the APTS-silica microsphere displayed the characteristic peaks of amino groups at the range of 1400–1460 cm−1, and the AA-APTS-silica microspheres displayed the relatively strong band of carbonylic groups at 1715 cm−1. The results reveal that the two-step chemical modification resulted in the organic AA-APTS monolayer at the surface of silica microspheres.
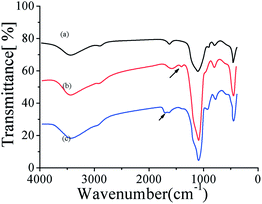 |
| Fig. 4
FT-IR to show the synthesis of aminopropyl-midfied HSMMs, AA-APTS-HSMMs, (a) pure silica, (b) APTS-silica, (c) AA-APTS-silica microspheres.. | |
3.3 Characterization of the adsorption ability of the TIHSM
The binding characteristics of imprinted and non-imprinted hollow shell microspheres were measured by equilibrium binding experiments. Varying tetradifon concentrations from 10.0 to 50.0 μg mL−1 in the presence of 10.0 mg hollow shell microspheres, the equilibrium binding experiments were carried out. Binding isotherms were plotted by the adsorption capacity (Q) of adsorbate bound to the polymerversus initial concentration of the adsorbate (Fig. 5).
 |
| Fig. 5 Binding isotherms were plotted by the adsorption capacity (Q) of adsorbate bound to the polymerversus initial concentration of the adsorbate. | |
As can be seen from Fig. 5, the adsorption capacity to tetradifon increased with the increase of initial concentration of tetradifon within a certain range. When the polymer was saturated and all the sites were filled, the adsorption capacity leveled off and remained constant. Adsorption capacity is determined by the initial concentration of analyte subtracting the concentration of un-adsorbed analyte remaining in the supernatant. The data of the TIHSMs obtained was used for the Scatchard analysis to estimate the binding parameters of the TIHSMs. Scatchard analysis equation is defined as Q/c = (Qmax − Q)/Kd, where c is the initial concentration, Kd is the equilibrium dissociation constant, Qmax is the apparent maximum amount. As shown in Fig. 6, the relationship between Q/c and Q can be expressed by two linear parts, suggesting that two different types of binding sites were formed in the PICSMs. According to Scatchard equation, Kd1 and Qmax1 values for the higher affinity binding sites and apparent maximum amount are calculated to be 44.5 μg mL−1 and 15.5 mg g−1. By the same treatment, Kd2 and Qmax2for the lower affinity binding sites are 21.1 μg mL−1 and 12.6 mg g−1 (Fig. 6).
 |
| Fig. 6 Scatchard plot to estimate the binding characteristic of TIHSMMs. | |
It was found in Fig. 6 that Scatchard plot is a single straight line and the Kd and Qmax of the affinity binding sites for tetradifon were calculated to be 37.4 μg mL−1 and 13.7 mg g−1, respectively.
In the experiment, we compared this method with others using normal imprinted material as sorbents,27 this method is superior in adsorption capacity due to double-side hollow molecularly imprinted materials. Briefly, 30.0 μg mL−1tetradifon in the presence of 10.0 mg hollow shell microspheres and normal imprinting materials, the binding experiments were carried out. The Qmax are 6.3 mg g−1 and 4.1 mg g−1, respectively, which would illustrate the better capacity of double-sided hollow molecularly over normal imprinted material.
3.3 The optimization of experimental variable in the determination
3.3.1 The investigation of adsorption time.
The time tetradifon solution or sample solution took to flow through the TIHSMs column is defined as the adsorption time. The adsorption amount was determined by the adsorption time, and the geodesic method is the most sensitive and precise when the adsorption amount is saturated. The adsorption time is relevant to concentration of the tetradifon, binding capacity of the polymer, and the flow rate. When the amount of the polymer is 10.0 mg and the flow rate is 2.4 mL min−1, the relation between the CL intensity and the adsorption time within the range 10–70 s were examined using 2.0 μg mL−1 of tetradifon solution. The CL intensity was increased with the increase of adsorption time up to 50 s. Above 50 s, the CL intensity remained constant. Considering analytical efficiency and the linear range of this method, 40 s was finally selected as adsorption time. It should be mentioned that for the analysis of a sample with lower tetrafion content, the sensitivity of the detection could be improved by increasing the adsorption time.
3.3.2 The investigation of time for washing off impurities.
A suitable washing time should be long enough to remove impurities adsorbed onto the polymer by non-specific interaction completely but can not cause loss of tetradifon adsorbed in TIHSMs column. Because rogor can react with luminol, hydrogen peroxide and sodium hydroxide, then it was selected as interference indictor to optimize the wash time. The effect of washing time on CL intensity was examined in the range of 20–90 s with flow rate at 2.4 mL min−1 (tetradifon: 2.0 μg mL−1, rogor: 20 μg mL−1). The experimental results showed that when the washing time was ≥50 s, the CL intensity showed no obvious change with that of the same concentration (2.0 μg mL−1) of tetradifon standard solution in the absence of rogor. It showed the inference indicator, rogor, was effectively removed. The optimum time is 50 s.
3.3.3 Response time.
When luminol, hydrogen peroxide, and sodium hydroxide flow through the TIHSMs column, they react with tetradifon adsorbed on the polymer to produce the CL signals. The CL signal intensity returned to baseline at 12 s. The results showed that the optimum response time was 15 s.
3.3.4 Cleaning time.
For recycling the TIHSMs, the cell should be cleaned. The cleaning time was optimized from 20 to 120 s with a blank TIHSMs column as control. A time of 70 s was selected as optimal for cleaning.
3.3.5
CL reaction conditions.
The concentration of chemiluminescent reagents has a great effect on CL intensity. To obtain the strongest CL intensity, the effects of reagents concentrations including hydrogen peroxide and sodium hydroxide on the CL reaction were also examined. The optimal concentrations for hydrogen peroxide and sodium hydroxide were 0.6 mol L−1 and 0.05 mol L−1 for further determination, respectively. And the optimal concentration for luminol is 4.0 × 10−5 mol L−1.
3.3.6 Analytical performance.
Under the optimum conditions, the analytical performance of the proposed method was obtained. The relative CL intensity (I) was linear to concentration (c) of tetradifon from 1.0 × 10−7 to 1.2 × 10−5 g mL−1. The linear regression equations were ΔI = 1984 + 225.8 c (c: μg mL−1) (r = 0.9957). The detection limit of tetradifon was found to be 4.83 × 10−8 g mL−1 according to 11 time parallel determination for the blank solution. The relative standard deviation (RSD) was 1.4% by 11 replicate determinations of 2.0 μg mL−1tetradifon (Fig. 7).
 |
| Fig. 7 Calibration graph. | |
3.3.7 The investigation of interfering effects.
In this work, coexisting substances were added into the tetradifon solution (2.0 μg mL−1) to investigate the effects on CL intensity respectively. The tolerable fold of coexisting substances in sample with TIHSMs was estimated when relative error was less than ±5%. The results are shown in Table 1.
Table 1 The tolerable ration of interfering species to tetradifon
Coexisting substances |
Non-imprinting column (fold) |
With TIHSMs column (fold) |
Al3+ |
0.2 |
5 |
Fe2+ |
1.5 |
100 |
Cu2+ |
Severe interfere |
5 |
Zn2+ |
Severe interfere |
2.5 |
B3+ |
Severe interfere |
5 |
Bi2+ |
0.05 |
10 |
Co2+ |
2 |
50 |
Vc
|
2 |
50 |
Fenpropathrin
|
5 |
100 |
Omethoate
|
2 |
5 |
Trichlorfon
|
2 |
500 |
Acephate
|
2 |
50 |
Pendimethalin
|
2 |
25 |
As can be seen from Table 1, interference of coexisting substance was greatly erased with TIHSMs as recognition material. Based on this, the deficiency of selectivity in CL was figured out by molecular imprinting chemiluminescence (MI-CL) sensor.
4. Application
In order to evaluate the applicability and reliability of the proposed method, it was applied to the determination of tetradifon in three samples: tomato, soybean and apple. 10.0 g sample (tomato, soybean and apple) was added into a conical flask, then 50 ml 5% methanol was added, mixed well for 24 h by ultrasonic oscillation. Finally, the mixture was filtrated and the filtrate was concentrated to 25 mL with rotary evaporator. The results of the determination of tetradifon in samples using the proposed method are listed in Table 2.
Table 2 Determination results of tetradifon in foodstuff
Sample |
Found ± SDa/mg kg−1 |
Added/mg kg−1 |
Recovered/mg kg−1 |
Recovery (%) |
Average of six determination.
|
Apple |
0.585 ± 0.007 |
2.0 |
1.92 |
96.00 |
5.0 |
5.14 |
102.8 |
Tomato |
0.632 ± 0.008 |
2.0 |
2.07 |
103.5 |
5.0 |
5.12 |
102.4 |
As shown in Table 2, from the value of the RSD and the recovery, it can come to a conclusion that the method has good precision and accuracy. Then it can be seen that, the MI-CL sensor used in the determination of pesticides in foodstuff demonstrated good sensitivity and stability.
5. Conclusion
In this work, the FI-CL system was first successfully applied to determination of tetradifon and the double-sides hollow tetradifon-imprinted microsphere was first synthesized. So, a novel molecular imprinting-chemiluminescence sensor was proposed. And the new sensor offers the advantages of convenient operation and simple instrument. The sensor also has excellent sensitivity and rapid detection based on the combination of chemiluminescence and flow-injection. The new sensor achieved high-efficiency measurement of tetradifon residual in food with simple operation. Duo to the specific structure of the double-sides hollow shell, the detection limit has reached 0.0483 μg mL−1 and wider linear range was in the range 0.1 ∼ 12 μg ml−1. This method might be extended to determine other organochlorine insecticides.
Acknowledgements
This work was financially supported by the National Natural Science Foundation of People's Republic of China (No. 50972050 and 50972052) and Key Projects in the National Science and Technology Pillar Program during the Eleventh Five-year Plan Period, China (2006BAJ03A09).
References
- Z. A. Grosser, J. F. Ryan and M. W. Dong, Environmental chromatographic methods and regulations in the United States of America, J. Chromatogr. A, 1993, 642(1–2) CAS.
- W. W. Sans, Multiple insecticide residue determination using column chromatography, chemical conversion, and gas-liquid chromatography, J. Agric. Food Chem., 1967, 15(1) CrossRef CAS.
- S. R. Rissato, M. S. Galhiane, B. M. Apon and M. S. P. Arruda, Multiresidue Analysis of Pesticides in Soil by Supercritical Fluid Extraction/Gas Chromatography with Electron-Capture Detection and Confirmation by Gas Chromatography–Mass Spectrometry, J. Agric. Food Chem., 2005, 53(1) CrossRef CAS.
- J. M. Lin and M. Yamada, Chemiluminescent Reaction of Fluorescent Organic Compounds with KHSO5 Using Cobalt(II) as Catalyst and Its First Application to Molecular Imprinting, Anal. Chem., 2000, 72(6), 1148–1155 CrossRef CAS.
- M. J. Navas and A. M. Jimenez, Chemiluminescent Methods in Alcoholic Beverage Analysis, J. Agric. Food Chem., 1999, 47(1), 183–189 CrossRef CAS.
- L. J. Kricka, Chemiluminescence and Bioluminescence, Anal. Chem., 1999, 71(12), 305–308 CrossRef.
- C. Dodeigne, L. Thunus and R. Lejeune, Chemiluminescence as diagnostic tool, Talanta, 2000, 51(3), 415–439 CrossRef CAS.
- L. J. Kricka, Clinical applications of Chemiluminescence, Anal. Chim. Acta, 2003, 500(1–2), 279–286 CrossRef CAS.
- C. X. Sun, J. H. Yang, L. Li, X. Wu, Y. Liu and S. F. Liu, Advances in the study of luminescence probes for proteins, J. Chromatogr., B: Anal. Technol. Biomed. Life Sci., 2004, 803(2), 173–190 CrossRef CAS.
- I. Surugiu, B. Danielsson, L. Ye, K. Mosbach and K. Haupt, Chemiluminescence Imaging ELISA Using an Imprinted Polymer as the Recognition Element Instead of an Antibody, Anal. Chem., 2001, 73(3), 487–491 CrossRef CAS.
- F. Fini, G. Gallinella, S. Girotti, M. Zerbini and M. Musiani, Development of a Chemiluminescence Competitive PCR for the Detection and Quantification of Parvovirus B19 DNA Using a Microplate Luminometer Parvovirus B19 DNA Using a Microplate Luminometer, Clin. Chem, 1999, 45, 1391–1396 CAS.
- J. Wang, D. Xing, Y. G. He and X. J. Hu, Localization of tumor by chemiluminescence probe during photosensitization action, Cancer Lett., 2002, 188(1–2), 59–65 CrossRef CAS.
- K. Miura, Localization of tumor by chemiluminescence probe during photosensitization action, Electrophoresis, 2001, 22(5), 801–813 CrossRef CAS.
- K. Robards and P. J. Worsfold, Analytical applications of liquid-phase chemiluminescence, Anal. Chim. Acta, 1992, 266(2), 147–173 CrossRef CAS.
- A. Townshend, Solution chemiluminescence—some recent analytical developments, Analyst, 1990, 115(5), 495–498 RSC.
- L. P. Palilis and A. C. Calokerinos, Anal. Chim. Acta, 2000, 413, 175–198 CrossRef CAS.
- L. Andersson, B. Sellergren and K. Mosbach, Imprinting of amino acid derivatives in macroporous polymers, Tetrahedron Lett., 1984, 25(45), 5211–5214 CrossRef CAS.
- X. Li and S. M. Husson, Two-Dimensional Molecular Imprinting Approach to Produce Optical Biosensor Recognition Elements, Langmuir, 2006, 22(23), 9658–9663 CrossRef CAS.
- J. Matsui, S. Goji, T. Murashima, D. Miyoshi, S. Komai, A. Shigeyasu, T. Kushida, T. Miyazawa, T. Yamada, K. Tamaki and N. Sugimoto, Molecular Imprinting under Molecular Crowding Conditions: An Aid to the Synthesis of a High-Capacity Polymeric Sorbent for Triazine Herbicides, Anal. Chem., 2007, 79(4), 1749–1757 CrossRef CAS.
- H. J. Liang, T. E. Angelini, J. Ho, P. V. Braun and G. C. L. Wong, Molecular Imprinting of Biomineralized CdS Nanostructures: Crystallographic Control Using Self-Assembled DNA–Membrane Templates, J. Am. Chem. Soc., 2003, 125(39), 11786–11787 CrossRef CAS.
- L. Ye and K. Mosbach, Polymers Recognizing Biomolecules Based on a Combination of Molecular Imprinting and Proximity Scintillation: A New Sensor Concept, J. Am. Chem. Soc., 2001, 123(12), 2901–2902 CrossRef CAS.
- C. Jin Tan and Y. W. Tong, The Effect of Protein Structural Conformation on Nanoparticle Molecular Imprinting of Ribonuclease A Using Miniemulsion Polymerization, Langmuir, 2007, 23(5), 2722–2730 CrossRef.
- C. Y. Yu, S. Chen, X. Quan, X. X. Ou and Y. B. Zhang, Separation of Phthalocyanine-like Substances from Humic Acids Using a Molecular Imprinting Method and Their Photochemical Activity under Simulated Sunlight Irradiation, J. Agric. Food Chem., 2009, 57(15), 6927–6931 CrossRef CAS.
- C. J. Tan, H. G. Chua, K. H. Ker and Y. W. Tong, Preparation of Bovine Serum Albumin Surface-Imprinted Submicrometer Particles with Magnetic Susceptibility through Core–Shell Miniemulsion Polymerization, Anal. Chem., 2008, 80(3), 683–692 CrossRef CAS.
- L. P. Palilis and A. C. Calokerinos, Analytical applications of chemiluminogenic reaction, Anal. Chim. Acta, 2000, 413(1–2), 175–186 CrossRef CAS.
- S. Q. Liu, J. C. Rao, X. Y. Sui, P. Cool, E. F. Vansant, G. V. Tendeloo and X. Cheng, Preparation of hollow silica spheres with different mesostructures, J. Non-Cryst. Solids, 2008, 354, 826–830 CrossRef CAS.
- S. G. Ge, M. Yan, X. L. Cheng and C. C. Zhang, On-line molecular imprinted solid-phase extraction flow-injection fluorescence sensor for determination of florfenicol in animal tissues, J. Pharm. Biomed. Anal., 2010, 52, 615–619 CrossRef CAS.
|
This journal is © The Royal Society of Chemistry 2012 |
Click here to see how this site uses Cookies. View our privacy policy here.