DOI:
10.1039/C2RA00542E
(Paper)
RSC Adv., 2012,
2, 3274-3280
Biotinylated boronic acid fluorophore conjugates: Quencher elimination strategy for imaging and saccharide detection
Received
1st August 2011
, Accepted 22nd November 2011
First published on 24th February 2012
Abstract
A biotinylated boronic acid fluorophore conjugate bound to a diol-quencher via a boronic ester linkage demonstrated a fluorescence increase upon exposure to model saccharides. The sensing motif was attached to SuperAvidin microspheres where the sensing regime was imaged by fluorescence microscopy.
Introduction
The reversible interaction of boronic acids with 1,2- and 1,3-diol motifs (Scheme 1) have allowed boronic acids to find many applications in self-assembly,1,2 sensing and separation science.3–12
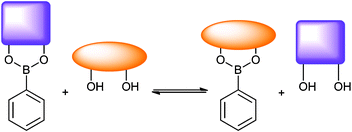 |
| Scheme 1 Equilibrium depicting boronic ester diol exchange. Relative stability constant K determines whether one diol displaces another in aqueous media. | |
Dye displacement assays exploit competitive binding in a sensor system where dye and analyte molecules are exchanged by a receptor, the dye release results in some colour change thus revealing the amount of analyte present.13–26 Singaram used fluorophore–viologen quencher interactions for diol recognition,20,24,27–35 and it was proposed by us that the ‘molecular beacon’ fluorophore—quencher pair technology used in PCR assays such as Taqman™ may be used in the study of boron diol interactions.36 In order to study this premise a signalling regime was developed where the emission of fluorescent boronic acid, would be reduced upon exposure to a quencher appended analyte diol, since a static fluorophore–quencher pair is formed by boronic ester formation, the presence of the quencher analyte is signalled by fluorescence quenching. It was also shown by us that a fluorescein boronic acid derivative partnered with by a series of quencher diols functioned as a FRET quenching/sensing regime based upon boronate ester formation.36 We also showed how the presence of nucleosides could be similarly revealed by a quenching mechanism as a result of boronate ester formation.36
A bis-boronic acid derivative of fluorescein has also been used in the detection of saccharides,37,38 and found application in delineating selective recognition of protein tetraserine motifs.39 Zhong and co-workers found that boronic acid appended polyethylenimine greatly enhanced gene delivery efficiency to cells.40
Extending the scope of the quencher diol boronic acid interaction led us to investigate a self-assembled,41 boronic acid-based system for surface plasmon enhanced fluorescence detection of quencher diols.42 We demonstrated that SPR and fluorescence spectroscopy simultaneously signalled quencher binding through boronic ester formation.42
The molecule coined FLAB 1 (Fluorophore Linker boronic Acid Biotin, Fig. 1) which bears a terminal biotin for attachment to streptavidin and matched quencher-diol, (2, Fig. 2) was prepared and studied.42 Exposure of a streptavidin-FLAB functionalised gold surface to a quencher diol manifested both a fluorescence quenching and an SPR response (Scheme 2).
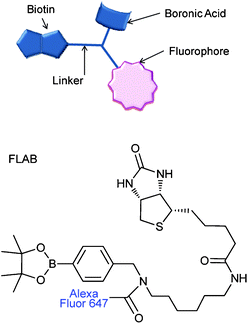 |
| Fig. 1 Boronic acid-fluorophore conjugate, linked to biotin for avidin attachment. FLAB = Fluorphore-Linker-boronic Acid-Biotin, 1. | |
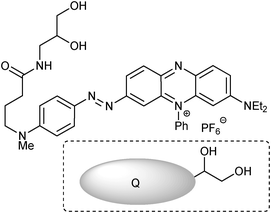 |
| Fig. 2 Diol appended BHQ (Biosearch Tech) quencher, 2. | |
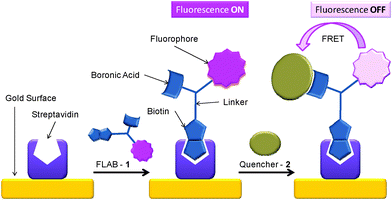 |
| Scheme 2 Schematic of surface attachment of FLAB (1) to gold and its exposure to 2, resulting in fluorescence loss. | |
Herein a biotin-appended, fluorophore, boronic ester, quencher conjugate was employed as a saccharide sensor exploiting a quencher elimination strategy. Boronic acid probes bearing a biotin group were prepared that served as an anchoring unit or a model targeted delivery moiety. Further, this permitted a quencher-elimination assay to be performed and imaged on commercial avidin-functionalised microsphere supports (10 μm size) in aqueous media.
Results and discussion
Whilst a surface appended system had already qualitatively confirmed quencher binding,42 we were eager to establish further data corresponding to FLAB's interaction with BHQ diol. As such we performed solution titrations, as summarised in Scheme 1. The results (shown in Fig. 3 and Fig. 4) indicate a one-to-one interaction in solution, as expected, thus confirming the formation of boronic ester conjugate 3 (Scheme 3).
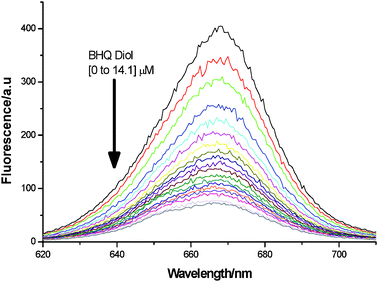 |
| Fig. 3 Fluorescence reduction upon conversion of 1 (2 μM) into 3, by addition of 2 (0 to 14.1 μM), at pH 8.23. | |
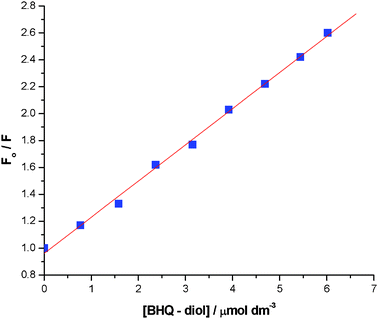 |
| Fig. 4
F
0/F (λem = 668 nm) for conversion of 1 (2 μM) into 3, by addition of 2 (0 to 6 μM), at pH 8.23. Stern–Volmer constant is 0.269 mmol−1 dm−3. | |
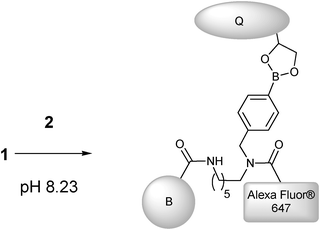 |
| Scheme 3 Preparation of 3, by addition of quencher (Q) (2) to Alexa Fluor FLAB (1) (B = Biotin). | |
With 3 in-hand we conceived of a quencher elimination strategy for carbohydrate detection (Scheme 4), whereby 3 would release quencher upon exposure to carbohydrate analytes resulting in a “fluorescence on” detection ensemble.
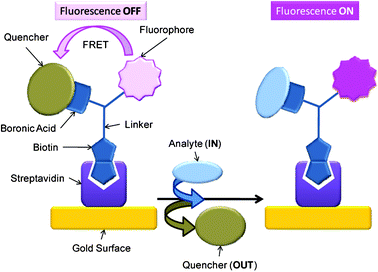 |
| Scheme 4 Schematic of quencher appended FLAB 3 attached to a support via biotin-avidin binding. Quencher bound to boronic acid inhibits fluorophore's emission, upon displacement of quencher with analyte (carbohydrate) fluorescence is recovered. | |
To test the fluorescence increase-quencher elimination strategy we took the solution of 3 obtained from Fig. 3 (including excess 2) and exposed it to fructose (Scheme 5). To our delight a significant increase of fluorescence was observed. So in spite of the excess quencher in solution the substitution of the static quencher (boronic ester) to dynamic (solution only) quencher (which is less efficient) produced a fluorescence increase as anticipated (Fig. 5 and Fig. 6). The apparent blue shift on fluorescence recovery might be due to the fact 1 is not regenerated upon fructose binding rather an alternative boronic ester (Scheme 5) is formed.
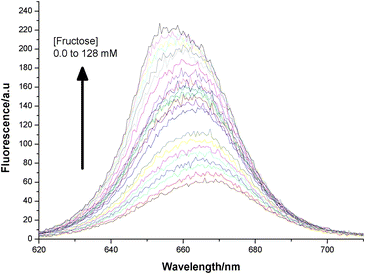 |
| Fig. 5 Fluorescence increase upon addition of fructose (0 to 128 mM) to 3 (2 μM plus excess BHQ diol) at pH 8.23. | |
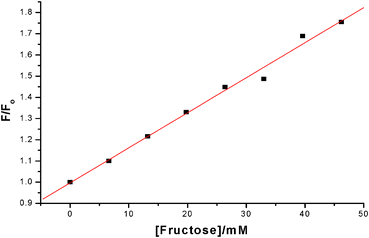 |
| Fig. 6 Plot of F/F0 (λemis = 668 nm) for addition of fructose to 3 (2 μM plus excess BHQ Diol) at pH 8.23 and linear fit over 0 to 50 mM range. | |
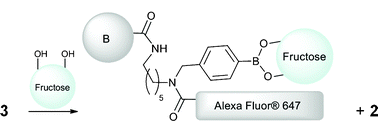 |
| Scheme 5 Quencher elimination upon addition of fructose to 3. | |
The Stern–Volmer eqn (1) suggests that the quotient of fluorescence intensity without quencher over fluorescence intensity with a quencher, should be linearly proportional to the quencher concentration, with the gradient giving the ratio of the quenching rate constant, kQ over the fluorescence rate constant kf and an intercept at [quencher] = 0 should be 1. This ratio, is the Stern–Volmer constant, KSV.
|  | (1) |
K
SV effectively quantifies the quenching ‘ability’ of the quencher–fluorophore system.
In the system described here, there is competitive binding for the boronic acid moiety of FLAB between fructose and the quencher diol. Measurement of the Stern–Volmer constant for different concentrations of fructose, shown in Fig. 7, displays the expected linear response and intercept at zero [quencher] = 1, the data obtained is summarised in Table 1. As expected, the higher the concentration of fructose, the lower the Stern–Volmer constant (Fig. 8). These results demonstrate that the FLAB-streptavidin-planar gold sensor construct functions as a dual mode detection platform.
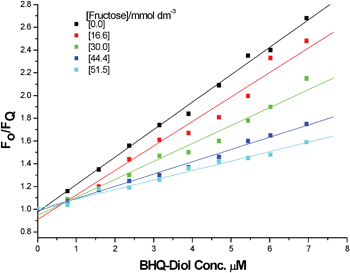 |
| Fig. 7
F
0/F (λemis = 662 nm) for five fixed concentrations of fructose and 1 (2 μM), titrating in 2. | |
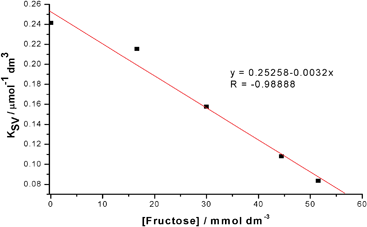 |
| Fig. 8 Stern–Volmer constants for 2 as a function of fructose concentration fixed 1 (2 μM), illustrating both a linear and negative proportionality. | |
Table 1 Stern–Volmer constants for FLAB 2 as a function of fructose concentration
[Fructose]/mol dm−3 |
0 |
16.6 |
30.4 |
44.4 |
51.5 |
K
SV/mmol−1 dm3 |
0.24146 |
0.21542 |
0.15776 |
0.10804 |
0.08351 |
± slope fit error |
± 0.00565 |
± 0.01224 |
± 0.00852 |
± 0.00346 |
± 0.00368 |
Imaging saccharide interactions with microspheres
Whilst surface appended sensing remains a key interest we were intrigued by the possibility of using FLAB as an imaging tool, so we exposed SuperAvidin Microspheres43 to FLAB. Microspheres find many applications in the biological arena, such as molecular/cell biology and immunology, and are particularly useful where the microsphere can be used to concentrate or immobilise biotinylated functional motifs to perform subsequent operations on solid support.44–48
FLAB-1 was prepared for use as an SPR enhanced fluorescence probe that was built to incorporate the ideal fluorophore for this scenario, Alexafluor 647. To probe the potential for using the FLAB construct in imaging a less expensive variant of FLAB was prepared using the same protocol but replacing the expensive fluorophore with SCN-fluorescein in the synthesis (see experimental section for a typical procedure). Such treatment of compound 4 with SCN-fluorescein delivered fluorescein-FLAB 5 (Scheme 6).
Following treatment of SuperAvidin Microspheres with fluorescein-FLAB, as depicted in Scheme 7, it was possible to obtain fluorescent microscopy images of the FLAB bound spheres. A composite image composed of a bright-field (greyscale) and a fluorescent (green) image is shown in Fig. 9.
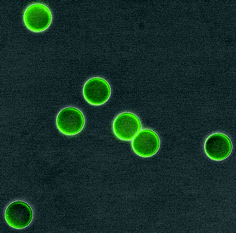 |
| Fig. 9 SuperAvidin Microspheres after exposure to FLAB, composite image obtained from DXM1200 digital camera coupled to a Nikon Eclipse TE2000-E microscope. A Nikon GFP blue-green filter cube was used for epifluorescence imaging, with lambda excitation 460-500 nm, emission 510-560 nm and dichroic 505 nm. | |
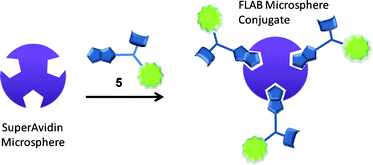 |
| Scheme 7 Generic scheme for microsphere-FLAB conjugation. | |
Encouraged by fluorescence microscopy images of SuperAvidin Microspheres bound by fluorescein-FLAB, which qualitatively revealed binding, we wished to develop a method to spatially record the fluorescence output, in order to assess the potential of microspheres to function as supports for carbohydrate sensing assays.
By tracking across the images obtained along a single direction, which bisects spheres, and recording pixel intensity it was possible to construct a plot representing cross-sphere fluorescence (Table 2).
Table 2
i Start situation: Left column shows the fluorescence of the FLAB-1 treated microspheres and the tracking fluorescence plot across spheres. ii Quencher added: Middle column shows the fluorescence of FLAB-1 bound microspheres after exposure to quencher diol 6 and the tracking fluorescence plot across spheres. iii Fructose added: Shows the fluorescence increase after quencher displacement resulting from addition of fructose to FLAB-Quencher conjugate appended microspheres, and the tracking fluorescence plot across spheres
Subsequently an on-microsphere quencher elimination experiment was conceived and in accordance with Scheme 4. Firstly, fluorescein-FLAB appended SuperAvidin Microspheres were visualised by fluorescence microscopy (Table 2, upper left) and a corresponding intensity plot tracking fluorescence across two spheres (Table 2, lower left). The edges of the spheres gave the strongest intensity output, up to ∼3000 counts versus zero background fluorescence. Microspheres thus prepared were then exposed to diol appended quencher 6 (Fig. 10, previously shown to be an effective quencher for a simple boronic acid-fluorescein conjugate),36 a new image (Table 2, upper middle) and corresponding intensity plot (Table 2, lower middle) were recorded. Across the linear track investigated which bisects two spheres no obvious fluorescence peaks were discernible, against a background fluorescence of less than 200 counts. Finally the FLAB-Quencher conjugate appended microspheres were treated with fructose and a third image was recorded (Table 2, upper right) again with a corresponding intensity plot (Table 2, lower right). Upon exposure to fructose the fluorescence intensity of the spheres bisected by a linear track had recovered fluorescence intensity up to a maximum of ∼3000 counts although background fluorescence had climbed to ∼1000 counts.
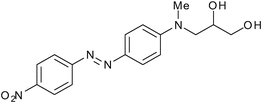 |
| Fig. 10 Quencher-Diol 6. | |
Gratifyingly, the fluorescence recovery measured in the FLAB-1 solution assays is visually corroborated in our microsphere system thus demonstrating a imaging/sensor platform for saccharides on a micro-scale.
Conclusions
A biotin appended fluorophore-boronic acid conjugate previously reported by us, coined FLAB, was exposed to a matched diol-appended quencher which delivers boronic ester statically quenched conjugates. Exposure of those conjugates to model saccharide, fructose, elicited quencher displacement resulting in a concomitant fluorescence recovery. This quencher elimination regime was transferred to a new scenario, SuperAvidin Microspheres, where the quencher elimination strategy could be visualised and measured on solid supports. We are currently investigating new mechanisms to enhance specificity in saccharide binding and hope to use systems inspired by those reported here in a variety of imaging scenarios.
Experimental
N–(6-Aminohexyl)-5-((3aS,4S,6aR)-2-oxohexahydro-1H-thieno[3,4-d]imidazol-4-yl)pentanamide
Biotin-NHS ester (0.55 g, 1.61 mmol) was added to a solution of N-Boc-hexamethylene diamine (0.35g, 1.6 mmol) and triethylamine (0.2 mL) in dichloromethane (5 mL). The reaction mixture was stirred overnight, then evaporated to dryness. The crude mixture was briefly refluxed in fresh dichloromethane to extract the product and filtered. The filtrate was evaporated to yield the N-Boc protected material as a yellow/orange oil, which was then taken up in dichloromethane (5 mL) and trifluoroacetic acid (5 mL) was added. The reaction was stirred for 4 h and then the mixture evaporated to dryness. Fresh dichloromethane was added, then removed in vacuo to give the title compound as a pale cream solid (0.5 g, 90% yield). δH (300 MHz, CD3OD) 7.98 (1H, s), 4.50 (1H, dd, J 7.8 & 4.7), 4.32 (1H, dd, J 7.8 & 4.4), 3.25–3.15 (3H, m), 2.97–2.91 (3H, m), 2.21 (3H, t, J 7.3), 1.87–1.36 (12H, m). MS (ESI+) [M + H] Found 343.2270 Required (C16H30N4O2S) 343.2089.
(4-(((6-(5-((3aS,4S,6aR)-2-Oxohexahydro-1H-thieno[3,4-d]imidazol-4-yl)pentanamido)hexyl)amino)methyl)phenyl)-boronic acid (4)
N-(6-Aminohexyl)-5-((3aS,4S,6aR)-2-oxohexahydro-1H-thieno[3,4-d]imidazol-4-yl)pentanamide (0.5 g, 1.45 mmol) was taken up in methanol (5 mL) and added to a solution of 4-formylbenzene boronic acid pinacol ester (0.37 g, 1.6 mmol) in dichloromethane (10 mL). 3 Å molecular sieves (1 g) were added and the reaction stirred overnight. Sodium borohydride (0.18 g, 4.8 mmol) was added at 0 °C and the reaction stirred at room temperature for 3 h. Ethyl acetate was added slowly until no further gas was evolved. The mixture was filtered and the solvents removed in vacuo. The crude mixture was dissolved in the minimum amount of methanol and then chloroform (100 mL) was added. The solution was washed with saturated bicarbonate solution and then dried over sodium sulfate. The solvent was removed in vacuo to give 4 as a waxy white solid (0.46 g, 67% yield). δH (300 MHz, CD3OD) 7.98 (1H, s), 7.48 (2H, d, J 7.6), 7.11 (2H, d, J 7.6), 4.47 (1H, dd, J 7.8, 4.7 Hz), 4.28 (1H, dd, J 7.8, 4.4), 3.70 (2H, s), 3.23-3.06 (3H, m), 2.97–2.87 (3H, m), 2.19 (3H, t, J 7.3), 1.88–1.26 (12H, m). MS (ESI+) [M] Found 476.2596 Required (C23H37BN4O4S) 476.2629.
Typical procedure for fluorophore attachment.
Activated fluorphore, such as fluorescein isothiocyanate (FITC, 1 eq), 1 (1 eq) and triethylamine (2 eq) were combined in dry DMF (10 mL mmol−11) under N2 and allowed to stir overnight. The solvent was removed in vacuo and the crude mixture subjected to purification by flash chromatography (silica gel, 95
:
5 DCM/MeOH).
Acknowledgements
We are grateful to the University of Bath Research Innovation Services (RIS) for an Enterprise Development fund which initiated cooperative work. JSF and TDJ thank the Catalysis And Sensing for our Environment (CASE) consortium for networking opportunities, and the EPSRC (DT/F00267X/1). JSF thanks the University of Birmingham, ERDF AWM II, the Leverhulme Trust (F/00351/P and F/00094/BC) and the Royal Society (Research Grant 2007 R2). Colin Wright from Nikon Bioimaging Ltd is thanked for his assistance in recording and interpreting digital images, and Nikon Bioimaging UK Ltd for providing training and a loan instrument to SIP. SIP also thanks the MRC for funding (Reference: G0901654) and the Royal Society for a University Research Fellowship. Haobo Ge and Zhiyuan Hu are thanked for their assistance in finalising the experiments for manuscript.
References
- E. Galbraith, A. M. Kelly, J. S. Fossey, G. Kociok-Kohn, M. G. Davidson, S. D. Bull and T. D. James, New J. Chem., 2009, 33, 181–185 RSC
.
- R. Nishiyabu, Y. Kubo, T. D. James and J. S. Fossey, Chem. Commun., 2011, 47, 1124–1150 RSC
.
- M. P. Pereira Morais, J. D. Mackay, S. K. Bhamra, J. G. Buchanan, T. D. James, J. S. Fossey and J. M. van den Elsen, Proteomics, 2010, 10, 48–58 CrossRef CAS
.
- F. D'Hooge, D. Rogalle, M. J. Thatcher, S. P. Perera, J. M. H. van den Elsen, A. T. A. Jenkins, T. D. James and J. S. Fossey, Polymer, 2008, 49, 3362–3365 CrossRef CAS
.
- T. R. Jackson, J. S. Springall, D. Rogalle, N. Masumoto, H. C. Li, F. D'Hooge, S. P. Perera, A. T. A. Jenkins, T. D. James, J. S. Fossey and J. M. H. van den Elsen, Electrophoresis, 2008, 29, 4185–4191 CrossRef CAS
.
-
J. S. Fossey and T. D. James, in Reviews in Fluorescence, ed. C. D. Geddes and J. R. Lakowicz, Springer, 2009, 103–118 Search PubMed
.
-
J. S. Fossey and T. D. James, in Supramolecular Chemistry: From Molecules to Nanomaterials, ed. P. A. Gale and J. W. Steed, John Wiley & Sons Ltd., 2012 Search PubMed
.
- R. Nishiyabu, Y. Kubo, T. D. James and J. S. Fossey, Chem. Commun., 2011, 47, 1106–1123 RSC
.
- Y.-J. Huang, Y.-B. Jiang, S. D. Bull, J. S. Fossey and T. D. James, Chem. Commun., 2010, 46, 8180–8182 RSC
.
- A. M. Kelly, Y. Perez-Fuertes, J. S. Fossey, S. L. Yeste, S. D. Bull and T. D. James, Nat. Protoc., 2008, 3, 215–219 CrossRef CAS
.
- Y. Perez-Fuertes, A. M. Kelly, J. S. Fossey, M. E. Powell, S. D. Bull and T. D. James, Nat. Protoc., 2008, 3, 210–214 CrossRef CAS
.
- T. A. Schuller, M. Kuball, S. E. Flower, T. D. James, J. S. Fossey, D. Marcon, J. Das, S. Degroot, M. Germain and A. Sarua, Sens. Actuators, B, 2011, 160, 1078–1081 CrossRef CAS
.
- S. L. Wiskur, H. Ait-Haddou, J. J. Lavigne and E. V. Anslyn, Acc. Chem. Res., 2001, 34, 963–972 CrossRef CAS
.
- S. C. McCleskey, P. N. Floriano, S. L. Wiskur, E. V. Anslyn and J. T. McDevitt, Tetrahedron, 2003, 59, 10089–10092 CrossRef CAS
.
- A. Goodey, J. J. Lavigne, S. M. Savoy, M. D. Rodriguez, T. Curey, A. Tsao, G. Simmons, J. Wright, S. J. Yoo, Y. Sohn, E. V. Anslyn, J. B. Shear, D. P. Neikirk and J. T. McDevitt, J. Am. Chem. Soc., 2001, 123, 2559–2570 CrossRef CAS
.
- Y. S. Sohn, A. Goodey, E. V. Anslyn, J. T. McDevitt, J. B. Shear and D. P. Neikirk, Biosens. Bioelectron., 2005, 21, 303–312 CrossRef CAS
.
- A. P. Umali, E. V. Anslyn, A. T. Wright, C. R. Blieden, C. K. Smith, T. Tian, J. A. Truong, C. E. Crumm, J. E. Garcia, S. Lee, M. Mosier and C. P. Nguyen, Journal of Chemical Education, 2010 Search PubMed
.
- B. T. Nguyen and E. V. Anslyn, Coord. Chem. Rev., 2006, 250, 3118–3127 CrossRef CAS
.
- A. Buryak and K. Severin, Angew. Chem., Int. Ed., 2004, 43, 4771–4774 CrossRef CAS
.
- B. Vilozny, A. Schiller, R. A. Wessling and B. Singaram, Anal. Chim. Acta, 2009, 649, 246–251 CrossRef CAS
.
- S. Gamsey, A. Miller, M. M. Olmstead, C. M. Beavers, L. C. Hirayama, S. Pradhan, R. A. Wessling and B. Singaram, J. Am. Chem. Soc., 2007, 129, 1278–1286 CrossRef CAS
.
- A. Schiller, R. A. Wessling and B. Singaram, Angew. Chem., Int. Ed., 2007, 46, 6457–6459 CrossRef CAS
.
- Z. Sharrett, S. Gamsey, J. Fat, D. Cunningham-Bryant, R. A. Wessling and B. Singaram, Tetrahedron Lett., 2007, 48, 5125–5129 CrossRef CAS
.
- J. N. Camara, J. T. Suri, F. E. Cappuccio, R. A. Wessling and B. Singaram, Tetrahedron Lett., 2002, 43, 1139–1141 CrossRef CAS
.
- D. B. Cordes, A. Miller, S. Gamsey and B. Singaram, Anal. Bioanal. Chem., 2007, 387, 2767–2773 CrossRef CAS
.
- W. M. J. Ma, M. P. Pereira Morais, F. D'Hooge, J. M. H. van den Elsen, J. P. L. Cox, T. D. James and J. S. Fossey, Chem. Commun., 2009, 532–534 RSC
.
- J. T. Suri, D. B. Cordes, F. E. Cappuccio, R. A. Wessling and B. Singaram, Langmuir, 2003, 19, 5145–5152 CrossRef CAS
.
- J. T. Suri, D. B. Cordes, F. E. Cappuccio, R. A. Wessling and B. Singaram, Angew. Chem., Int. Ed., 2003, 42, 5857–5859 CrossRef CAS
.
- F. E. Cappuccio, J. T. Suri, D. B. Cordes, R. A. Wessling and B. Singaram, J. Fluoresc., 2004, 14, 521–533 CrossRef CAS
.
- D. B. Cordes, S. Gamsey, Z. Sharrett, A. Miller, P. Thoniyot, R. A. Wessling and B. Singaram, Langmuir, 2005, 21, 6540–6547 CrossRef CAS
.
- D. B. Cordes, A. Miller, S. Gamsey, Z. Sharrett, P. Thoniyot, R. Wessling and B. Singaram, Org. Biomol. Chem., 2005, 3, 1708–1713 CAS
.
- S. Gamsey, N. A. Baxter, Z. Sharrett, D. B. Cordes, M. M. Olmstead, R. A. Wessling and B. Singaram, Tetrahedron, 2006, 62, 6321–6331 CrossRef CAS
.
- Z. Sharrett, S. Gamsey, P. Levine, D. Cunningham-Bryant, B. Vilozny, A. Schiller, R. A. Wessling and B. Singaram, Tetrahedron Lett., 2008, 49, 300–304 CrossRef CAS
.
- Z. Sharrett, S. Gamsey, L. Hirayama, B. Vilozny, J. T. Suri, R. A. Wessling and B. Singaram, Org. Biomol. Chem., 2009, 7, 1461–1470 CAS
.
- A. Schiller, B. Vilozny, R. A. Wessling and B. Singaram, Anal. Chim. Acta, 2008, 627, 203–211 CrossRef CAS
.
- S. A. Elfeky, S. E. Flower, N. Masumoto, F. D'Hooge, L. Labarthe, W. B. Chen, C. Len, T. D. James and J. S. Fossey, Chem.–Asian J., 2010, 5, 581–588 CrossRef CAS
.
- K. K. Kim, J. O. Escobedo, N. N. St. Luce, O. Rusin, D. Wong and R. M. Strongin, Org. Lett., 2003, 5, 5007–5010 CrossRef CAS
.
- S. Jiang, J. O. Escobedo, K. K. Kim, O. Alptürk, G. K. Samoei, S. O. Fakayode, I. M. Warner, O. Rusin and R. M. Strongin, J. Am. Chem. Soc., 2006, 128, 12221–12228 CrossRef CAS
.
- T. L. Halo, J. Appelbaum, E. M. Hobert, D. M. Balkin and A. Schepartz, J. Am. Chem. Soc., 2009, 131, 438–439 CrossRef CAS
.
- Q. Peng, F. Chen, Z. Zhong and R. Zhuo, Chem. Commun., 2010, 46, 5888–5890 RSC
.
- N. S. Murray, S. P. Jarvis and T. Gunnlaugsson, Chem. Commun., 2009, 4959–4961 RSC
.
- S. A. Elfeky, F. D'Hooge, L. Poncel, W. B. Chen, S. P. Perera, J. M. H. van den Elsen, T. D. James, A. T. A. Jenkins, P. J. Cameron and J. S. Fossey, New J. Chem., 2009, 33, 1466–1469 RSC
.
- Bangs Laboratories, Inc. product number CP01N/9721.
- G. Fry, E. Lachenmeier, E. Mayrand, B. Giusti, J. Fisher, L. Johnstondow, R. Cathcart, E. Finne and L. Kilaas, Biotechniques, 1992, 13, 124–131 CAS
.
- H. M. Ji and L. M. Smith, Anal. Chem., 1993, 65, 1323–1328 CrossRef CAS
.
- R. Fujita and A. Swaroop, Biotechniques, 1995, 18, 796–800 CAS
.
- X. C. Tong and L. M. Smith, Anal. Chem., 1992, 64, 2672–2677 CrossRef CAS
.
- A. Palma, M. Tasior, D. O. Frimannsson, T. T. Vu, R. Meéallet-Renault and D. F. O'Shea, Org. Lett., 2009, 11, 3638–3641 CrossRef CAS
.
Footnote |
† These authors contributed equally to this work |
|
This journal is © The Royal Society of Chemistry 2012 |
Click here to see how this site uses Cookies. View our privacy policy here.