DOI:
10.1039/C2RA00996J
(Paper)
RSC Adv., 2012,
2, 2504-2507
CO2 capture from flue gas at high temperatures by new ionic liquids with high capacity
Received
1st November 2011
, Accepted 2nd January 2012
First published on 7th February 2012
Abstract
Room-temperature ionic liquids (ILs) are widely investigated to capture CO2 from flue gas and can capture a large amount of CO2. However, almost all of the previous studies on the absorption of CO2 by ILs were carried out at low temperatures, less than 60 °C and even at room temperature. When the temperatures reach the real flue gas temperatures, from 110 °C to 140 °C, these ILs might hardly absorb CO2, as high temperatures decrease the CO2 capacity. Therefore, it is necessary to design new task specific ILs to capture CO2 at flue gas temperatures with high absorption capacity. In this work, a new type of polyamine based ILs were developed to absorb CO2 from simulated flue gas at high temperatures and ambient pressure with large absorption capacities up to 0.944 mole ratio or 0.176 mass ratio of CO2 to IL at 110 °C. These ILs could be easily synthesized by neutralization of polyamines and organic acids, and the number of amido groups on the ILs can be easily controlled. The CO2 absorption is influenced by temperature, CO2 volume fraction in the flue gas, and the number of amido groups on the ILs. Also a possible mechanism for the CO2 absorption has been proposed.
Introduction
Carbon dioxide, which is mainly emitted from the burning of fossil fuels, may cause global warming and climate change. The most efficient way to reduce the emission of CO2 may be CO2 capture after the burning of fuels. For years, many kinds of materials have been used to capture CO2, such as methanol, diethanolamine and N-methyldiethanolamine.1–3 Although they can capture CO2 on a large-scale, these materials cannot be used at high temperatures because they have high volatility. Besides, the difficulty of desorption and the high viscosity during absorption may also limit the reuse of these solvents.
Due to their extremely low vapor pressure, tunable structure, high thermal and chemical stability, and excellent solvent power, ionic liquids (ILs) are regarded as a kind of novel materials for CO2 capture. Until now, many kinds of ILs have been synthesized and used for CO2 capture. CO2 can physically be absorbed in normal ILs.4–8 For example, [Bmim][PF6] can physically absorb CO2 at high pressures.4 The physical absorption of CO2 in normal ILs depends on the pressure of CO2. The mole ratio of CO2 in ILs decreases when the partial pressure of CO2 decreases. At ambient pressure, normal ILs can hardly absorb CO2. As a result, these ILs cannot be used to capture CO2 in flue gas. Due to these reasons, Bates et al.9 synthesized a task-specific IL, 1-n-propylamine-3-butylimidazolium tetrafluoroborate, which can capture CO2 at ambient pressure. And after that many kinds of task-specific ILs were synthesized, such as tetraalkylammonium amino acids,10 tetrabutylphosphonium amino acids.11–14 These ILs are functionalized with an amido group at the anion and/or the cation. And they can capture a large amount of CO2 at ambient pressure.
As we know, the temperatures of flue gas are very high, for example, 110 °C. But all of the previous studies on ILs absorbing CO2 were carried out at low temperatures less than 60 °C and even at room temperature. The increase in temperature can decrease the absorption capacities of these ILs. As a result, when temperatures reach the real flue gas ones, these ILs might hardly absorb CO2. On the other hand, a decrease of flue gas temperatures in order to capture CO2 by ILs costs much energy. Therefore, it is necessary to design new task specific ILs to capture CO2 at flue gas temperatures with high absorption capacity. As we know, polyamine has a strong molecular interaction with CO2 and can absorb a large amount of CO2, but it is volatile especially at high temperatures. So it is possible to functionalize polyamines onto ILs to combine the extremely low volatility of ILs and the high CO2 absorption capacity of polyamines.
In this work, a new type of polyamine based ILs were developed to absorb CO2 from simulated flue gas at high temperatures and ambient pressure with large absorption capacities. The polyamine based ILs could be easily synthesized by neutralization of polyamines and organic acids.
Experimental
Materials
CO2 (99.995%) and N2 (99.99%) were supplied from Beijing Haipu Gases. Diethylene triamine, triethylene tetramine, lactic acid and trifluoroacetic acid were purchased from Shanghai Jingchun Reagent Plant (Shanghai, China). All the reagents and solvents were analytical reagents. Before the use of reagents, the water contents of them were determined by Karl Fischer titration (ZDJ-400S, Multifunctional Titrator, Beijing Xianqu Weifeng Company, Beijing, China).
Synthesis of ILs
The ILs used in this work were prepared by neutralization of polyamines in water with different acids. Taking triethylene tetramine lactate ([TETA]L) for example, the synthesis procedure is as follows: 0.5 mol of triethylene tetramine and 100 mL of water were added into a 500 mL three-necked flask. The flask was placed in an ice-water bath at 0 °C and equipped with a reflux condenser under vigorous stirring with a magnetic stirrer. 0.5 mol of lactic acid was added into a pressure funnel and was added dropwise to the flask over about 1 h. The reaction lasted for 4 h at 25 °C. The solvent was removed by evaporation under vacuum. After the drying process, the water fractions determined by Karl Fischer titration were less than 0.005. Using a similar method, we synthesized triethylene tetramine lactate ([TETA]L), triethylene tetramine trifluoroacetate ([TETA][Tfa]), diethylene triamine lactate ([DEA]L), triethylene tetramine bis(lactate) ([TETA]L2) and diethylene triamine bis(lactate) ([DEA]L2) for CO2 capture.
Apparatus and procedures
The CO2/N2 gas mixtures, with different CO2 contents by volume, were prepared by mixing CO2 and N2 at ambient pressure. The apparatus consisted mainly of a cylinder containing the pure CO2 gas, a cylinder containing the pure N2 gas, a test tube with an inner diameter of 12 mm and a length of 200 mm, two rotameters (Beijing Forth Automation Meter Factory, China) and a constant temperature oil bath. This absorption experiment was carried out at ambient pressure.
In a typical experiment, the pure CO2 gas and the pure N2 mixed together, and then went through the [TETA]L loaded in the test tube. The weight of the IL was about 3.2 g. The flow rate of each gas was monitored by rotameters and calibrated by a soap film fluid meter. The total flow rate of gas was 100 mL min−1. The test tube was partly immersed into the oil bath, the temperature of which was maintained within ±1 °C. After a given time for absorption, the mass ratio of CO2 to IL was determined by the mass difference of the test tube via an electrical balance (BS224S Sartorius) with an accuracy of ±0.0001 g. And then the mole ratio of CO2 to IL was calculated. The expanded uncertainty of CO2 mass ratio to IL is ±4.2%.
The desorption of CO2 was carried at 140 °C with N2 as the sweeping gas. The desorbing time was about 0.5 h.
In this work, we also have measured the solubility of pure N2 in [TETA]L at 110 °C, and we found the mass ratio of N2 to IL is less than 0.001, which can be neglected compared with the absorption amounts of CO2.
Results and discussion
Effect of IL on the absorption of CO2
Fig. 1 shows the absorption of pure CO2 in the five ILs at 110 °C, indicating that all the ILs can absorb CO2 from simulated gas, but the mole ratios of CO2 to ILs are significantly different. At a high temperature of 110 °C, [TETA]L, [TETA][Tfa] and [DEA]L show high capacities of CO2 absorption, from 0.54 to 0.94 mole ratios of CO2 to IL, and from 0.12 to 0.17 mass ratios of CO2 to IL, which are higher than that reported in the literature.13,14 The most efficient IL is [TETA]L, which shows that the mole ratio of CO2 to IL reaches 0.944, and the mass ratio 0.176. To the best of our knowledge, this is the highest mass ratio of CO2 to task-specific IL. [TETA][Tfa] can also get a very high value of CO2 absorption, which shows a mole ratio of CO2 to IL of 0.901. When [DEA]L2 or [TETA]L2 was used to absorb CO2, the mole ratio of CO2 to IL decreased. For example, a mole ratio of CO2 to [DEA]L in equilibrium is 0.541, and that to [DEA]L2 is just 0.075.
![Absorption of CO2 in different kinds of ILs with pure CO2 at 110 °C: ■, [TETA]L; ●, [TETA][Tfa]; ▲, [DEA]L; ◀, [TETA]L2; ▼, [DEA]L2.](/image/article/2012/RA/c2ra00996j/c2ra00996j-f1.gif) |
| Fig. 1 Absorption of CO2 in different kinds of ILs with pure CO2 at 110 °C: ■, [TETA]L; ●, [TETA][Tfa]; ▲, [DEA]L; ◀, [TETA]L2; ▼, [DEA]L2. | |
The key reason for the different CO2 absorption capacities in these ILs may be the number of amido groups in the cation. With a decrease in free amido groups that are not neutralized by the acid, the mole ratio of CO2 to IL decreases. Both [TETA]L and [TETA][Tfa] have three free amido groups, and the mole ratios of these two ILs can reach about 0.9. Both [DEA]L and [TETA]L2 have two free amido groups, and the mole ratios of these two ILs can reach about 0.5. As compared to [TETA]L, the absorption of CO2 in [TETA]L2 decreased obviously. When there is only one free amido group left in the IL, [DEA]L2, the IL has a very low absorption of CO2 at a high temperature of 110 °C.
Effect of temperature on the absorption of CO2
As we know, the temperature has a great effect on CO2 absorption by ILs. In this paper, [TETA]L was employed to investigate the effect of temperature on the absorption of CO2. Fig. 2 shows the absorption of CO2 in [TETA]L with pure CO2 at different temperatures. The mole ratio of CO2 to [TETA]L increases with the decrease in temperature. For instance, when the temperature is 130 °C, the mole ratio of CO2 to [TETA]L can reach 0.660 in equilibrium. However, when the temperature decreases to 110 °C, the mole ratio of CO2 to [TETA]L increases to 0.944. Although the increase in temperature can decrease the absorption of CO2, the capacity of CO2 in [TETA]L can still reach a high value of 0.660 mol per mol IL. This is similar to the absorption of acid gases in task-specific ILs, such as SO2.15 Due to the influence of temperature on CO2 absorption, we can desorb CO2 at higher temperatures to reuse the ILs.
![Absorption of CO2 in [TETA]L with pure CO2 at different temperatures: ■, 110 °C; ●, 120 °C; ▲, 130 °C.](/image/article/2012/RA/c2ra00996j/c2ra00996j-f2.gif) |
| Fig. 2 Absorption of CO2 in [TETA]L with pure CO2 at different temperatures: ■, 110 °C; ●, 120 °C; ▲, 130 °C. | |
Effect of the volume fraction of CO2 in simulated flue gas on CO2 absorption
Fig. 3 shows the absorption of CO2 in [TETA]L with different volume fractions of CO2 at 110 °C. CO2 absorption capacity decreases with the decrease in CO2 volume fraction in simulated flue gas. For example, when the CO2 volume fraction in the simulated flue gas is 0.50, the mole ratio of CO2 to [TETA]L is 0.804. When the CO2 volume fraction is 0.15, which is close to the CO2 concentration in real flue gas, the mole ratio of CO2 to [TETA]L still reaches 0.547.
![Absorption of CO2 in [TETA]L with different volume fractions of CO2 at 110 °C: ■, 100%; ●, 50%; ▲, 25%; ▼, 15%.](/image/article/2012/RA/c2ra00996j/c2ra00996j-f3.gif) |
| Fig. 3 Absorption of CO2 in [TETA]L with different volume fractions of CO2 at 110 °C: ■, 100%; ●, 50%; ▲, 25%; ▼, 15%. | |
The desorption of CO2 in [TETA]L
In this work, the absorbed CO2 was released from [TETA]L upon sweeping by nitrogen at 140 °C for about 0.5 h. Fig. 4 shows the absorption and desorption of CO2 by [TETA]L for three cycles. The mole ratio of CO2 to [TETA]L in these three cycles were 0.944, 0.922 and 0.931 after the absorption at 110 °C. Experimentally, the IL can be reused to absorb CO2 with no obvious change in the absorption capacity and absorption rate.
![Absorption and desorption cycles of CO2 in [TETA]L.](/image/article/2012/RA/c2ra00996j/c2ra00996j-f4.gif) |
| Fig. 4 Absorption and desorption cycles of CO2 in [TETA]L. | |
The mechanism of CO2 absorbed in [TETA]L
To obtain some information about the chemical interaction between CO2 and the ILs used in this work, FTIR was used to characterize the fresh IL and CO2 absorbed IL, which is shown in Fig. 5. After absorption of CO2, a broad peak clearly appears at 2167 cm−1, which can be assigned to the C
O stretch of CO2. Compared with the pure CO2 FTIR spectrum (from NIST web), the wave number of the C
O stretch of CO2 absorbed by the IL decreases from 2350 cm−1. This suggests that CO2 is chemically interacts with the IL. The peak at 1661 cm−1 appears stronger after absorption, demonstrating the characteristic absorption peak of a carbamate C
O stretch.9 It is noted that the IL was spread on potassium bromide pellets and CO2 from air was absorbed in the thin film of the spread IL to a small extent. Therefore, the FTIR spectrum of fresh IL also shows a little CO2 information.
![FT-IR spectrum of [TETA]L before and after the absorption of CO2: solid line, before the absorption; dashed line, after the absorption.](/image/article/2012/RA/c2ra00996j/c2ra00996j-f5.gif) |
| Fig. 5
FT-IR spectrum of [TETA]L before and after the absorption of CO2: solid line, before the absorption; dashed line, after the absorption. | |
All the above results indicate that the new ILs can absorb CO2 at high temperatures, which hints that the molecular interaction between the IL and CO2 is stronger than in normal ILs that can be used only at near room temperature. But the absorbed CO2 can be recovered by increasing the temperature, suggesting that the chemical interaction is not very strong and is reversible. The absorption capacity is greatly influenced by the volume fraction of CO2 in flue gas, further demonstrating that a reversible chemical reaction happens for the CO2 absorption.
Inspired by the absorption mechanism reported by Bates et al.,9 we propose a mechanism for the new polyamine based ILs absorbing CO2 which is shown in Scheme 1.
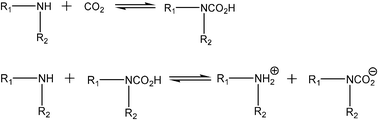 |
| Scheme 1 Proposed mechanism of the absorption of CO2. R1 stands for ionic liquids, R2 stands for alkyl chains. | |
One CO2 molecule interacts with two amino groups on the IL molecules to form a complex. Based on the above analysis, these ILs absorb CO2 following the chemical equilibrium in eqn (1).
| CO2 + 2 amino-IL = CO2(amino-IL)2 | (1) |
In
eqn (1), amino-IL stands for the free amino group on the IL, and CO
2(amino-IL)
2 for the complex. The chemical reaction equation has an equilibrium constant. Obviously, at a given temperature, such as 110 °C, the equilibrium constant is fixed, and CO
2 absorption capacity in the IL increases with the increase in CO
2 partial pressure. The equilibrium constant is a function of temperature. From
Fig. 2, a decrease in temperature favors CO
2 absorption, which means the equilibrium constant increases with a decrease in absorption temperature. An increase in the number of amino groups in the IL can increase the amino group concentration resulting in the increase of CO
2 absorption amount. However, during desorption of CO
2via vacuum and heat treatment, the increase in temperature decreases the equilibrium constant, and the decrease in CO
2 partial pressure decreases the concentration of CO
2(amino-IL)
2, both resulting in CO
2 desorption from the IL quickly.
Conclusion
A new type of ILs has been easily synthesized by neutralization of polyamines and organic acids and they can capture a large amount of CO2 from simulated flue gas at high temperatures and ambient pressure with low contents of CO2. The CO2 absorption is influenced by temperature, CO2 volume fraction in flue gas, and IL. The CO2 absorption capacity of the ILs is increased by the number of amido groups on the ILs, which give an IL absorption function and can be adjusted by the mole ratio of polyamines to acids during synthesis. A possible mechanism of the CO2 absorption has been proposed.
Acknowledgements
The authors thank Prof. Chengyue Li, Prof. Zhenyu Liu and Dr Qingya Liu for their help. The project is financially supported by the Natural Science Foundation of China (21176020) and Program for New Century Excellent Talents in University (NSET-08-0710).
References
- N. MacDowell, N. Florin, A. Buchard, J. Hallett, A. Galindo, G. Jackson, C. S. Adjinman, C. K. Williams, N. Shah and P. Fennell, Energy Environ. Sci., 2010, 3, 1645–1669 CAS.
- J. M. Navaza, D. Gomez-Diaz and M. D. L. Rubia, Chem. Eng. J., 2009, 146, 184–188 CrossRef CAS.
- M. Kanniche and C. Bouallou, Appl. Therm. Eng., 2007, 27, 2693–2702 CrossRef.
- L. A. Blanchard, D. Hancu, E. J. Beckman and J. F. Brennecke, Nature, 1999, 399, 28–29 CrossRef.
- L. A. Blanchard, Z. Y. Gu and J. F. Brennecke, J. Phys. Chem. B, 2001, 105, 2437–2444 CrossRef CAS.
- J. L. Anthony, E. J. Maginn and J. F. Brennecke, J. Phys. Chem. B, 2002, 106, 7315–7320 CrossRef CAS.
- M. B. Shiflett and A. Yokozeki, Ind. Eng. Chem. Res., 2005, 44, 4453–4464 CrossRef CAS.
- M. B. Shiflett and A. Yokozeki, J. Phys. Chem. B, 2007, 111, 2070–2074 CrossRef CAS.
- E. D. Bates, R. D. Mayton, L. Ntai and J. H. Davis Jr., J. Am. Chem. Soc., 2002, 124, 926–927 CrossRef CAS.
- Y. Y. Jiang, G. N. Wang, Z. Zhou, Y. T. Wu, J. Geng and Z. B. Zhang, Chem. Commun., 2008, 505–507 RSC.
- J. M. Zhang, S. J. Zhang, K. Dong, Y. Q. Zhang, Y. Q. Shen and X. M. Lv, Chem.–Eur. J., 2006, 12, 4021–4026 CrossRef CAS.
- G. R. Yu, S. J. Zhang, X. Q. Yao, J. M. Zhang, K. Dong, W. B. Dai and R. Mori, Ind. Eng. Chem. Res., 2006, 45, 2875–2880 CrossRef CAS.
- B. E. Gurkan, J. C. Fuente, E. M. Mindrup, L. E. Ficke, B. F. Goodrich, E. A. Price, W. F. Schneider and J. F. Brennecke, J. Am. Chem. Soc., 2010, 132, 2116–2117 CrossRef CAS.
- Y. Q. Zhang, S. J. Zhang, X. M. Lu, Q. Zhou, W. Fan and X. P. Zhang, Chem.–Eur. J., 2009, 15, 3003–3011 CrossRef CAS.
- W. Z. Wu, B. X. Han, H. X. Gao, Z. M. Liu, T. Jiang and J. Huang, Angew. Chem., Int. Ed., 2004, 43, 2415–2417 CrossRef CAS.
|
This journal is © The Royal Society of Chemistry 2012 |
Click here to see how this site uses Cookies. View our privacy policy here.