DOI:
10.1039/C2RA01171A
(Paper)
RSC Adv., 2012,
2, 3340-3346
Photo-responsive release of ascorbic acid and catalase in CDBA-liposome for commercial application as a sunscreen cosmetic
Received
23rd November 2011
, Accepted 11th January 2012
First published on 24th February 2012
Abstract
Long-term exposure of the skin to UV light causes degenerative effects, which can be minimized by using antioxidant formulations. A remedy is that L-ascorbic acid (Vc) can be applied in commercial sunscreen cosmetics. This study was designed to determine whether 4-cholesterocarbonyl-4′-(N,N'-diethylaminobutyloxy)azobenzene (CDBA) liposome can undergo photoisomerization, and lead to release of ascorbic acid in CDBA-liposome under UV irradiation. Using the standard sonication method, Vc or catalase loaded liposomes containing CDBA were prepared. Size distribution of the liposome, photoisomerization of CDBA either in chloroform solution or in CDBA-liposome, stability of Vc or catalase, the release of Vc from Vc-loaded CDBA-liposome, and the protecting efficacy of CDBA-liposome upon UV irradiation were studied. The results showed that the average diameter of CDBA-liposome was 140 nm. CDBA both in solution and in liposome can undergo trans-to-cis isomerization upon UV irradiation and cis-to-trans isomerization by incubation at 37 °C. Furthermore, both Vc and catalase in CDBA-liposome were much more stable than in their free states, and the release of L-ascorbic acid from CDBA-liposome was photoresponsive. Meanwhile, it was confirmed by in vivo tests that the CDBA-liposome showed a good protecting efficacy from UV irradiation, which implies that this formulation could be used in commercial sunscreen cosmetics.
1. Introduction
UV radiation can be absorbed by different chromophores in the skin, and absorption of UV photons by these chromophores results in different photochemical reactions and secondary interactions involving reactive oxygen species (ROS), which result in harmful effects.1 UV radiation involves both ultraviolet UVB (290–320 nm) and UVA (320–400 nm). UVB radiation is mainly responsible for the most severe damage: acute damage such as sunburn, and long-term damage including cancer. It has a direct impact on cell DNA and proteins. Unlike UVB, UVA radiation is not directly absorbed by biological targets, but can still dramatically impair cell and tissue functions.2,3 As a result, a major concern has been raised that most available sunscreen products are incapable of preventing the harmful effects of UVA, the most abundant and persistent element of UV daily sunlight. As such, they could leave the skin overexposed for hours to intense doses of UVA radiation while users believe they are safe, as UVA does not cause any immediate perceptible clinical damage. That is why having efficient UVA filters to cover the entire range of harmful radiation and developing more effective sunscreen products have become crucial needs.
Ascorbic acid, also known as vitamin C, has been widely employed in commercial cosmetic preparations, including semisolid topical dosage forms. Ascorbic acid has important physiological effects on the skin, including promotion of collagen biosynthesis, inhibition of melanogenesis and prevention of free radical formation, all closely relating to the well-known antioxidant properties of this compound.4–8 Ascorbic acid therefore plays an important role in skin aging and may be considered a useful ingredient of cosmetic skincare products. Unfortunately, while ascorbic acid is safe it is very unstable.9,10 Ascorbic acid presents reasonable stability however when the storage conditions protect it from humidity, heat and luminosity. In aqueous solutions, it is decomposed by environmental oxygen and oxidising agents, particularly in the presence of alkalis and heavy metals, leading to dehydroascorbic acid and then diketogluconic acid. Oxalic acid is formed as the final degradation product of ascorbic acid.11,12 Chen et al. investigated that liposomal curcumin had higher stability than free curcumin in PBS13 while Ioele et al. showed that retinoic acids incorporated in liposome complex showed an increased stability in comparison to ethanol solution.14
Catalase (Cat) is a common enzyme found in nearly all living organisms that are exposed to oxygen, where it functions to catalyze the decomposition of hydrogen peroxide to water and oxygen.15 Catalase is essential to protect aerobic organisms from the toxic effects of H2O2. High amounts of this enzyme in the skin can impart antioxidative activity. Recently, catalase has begun to be used in the aesthetic industry. Several mask treatments combine the enzyme with hydrogen peroxide on the face with the intent of increasing cellular oxygenation in the upper layers of the epidermis. The enzyme reactions occurring in liposomes have received considerable attention because both the stability and reactivity of the liposomal enzyme are potentially regulated by keeping the molecular structure of the enzyme intact.
The photostability of commercial sunscreen products is considered a key parameter for effective in vitro evaluation of their effectiveness.16 A sunscreen product which loses its capacity to block UV radiation during exposure to the sun provides a clear risk of damage to the user. Therefore, photochromism has been attracting intense interest for potential use in commercial cosmetics.17–20 Some new photo-controlled azo compounds have been designed and synthesized by our group and they can be readily combined with bilayers of liposomes; their cholesterol group can minimize perturbation of membranes and spontaneous release of drugs encapsulated in the liposomes. The compounds can absorb UV light and photoisomerize from trans to cis forms under ultraviolet radiation.21,22 In this paper, we synthesized a photosensitive azobenzene compound, 4-cholesterocarbonyl-4′-(N,N'-diethylaminobutyloxy)azobenzene (CDBA), and included it in a liposomal membrane. The stability of encapsulated L-ascorbic acid (Vc) or catalase was studied within such a system. Moreover, the photo-controlled release of Vc, an in vivo test for UV protecting efficacy of this liposomal formulation were also assessed. Possible application in commercial cosmetics is of great interest.
2. Materials and methods
Egg phosphatidylcholine (PC), cholesterol, catalase from bovine and Triton X-100 were purchased from Sigma (USA). L-Ascorbic acid was purchased from Sino-American Biotechnology Co. (China). Sephadex G-50 was purchased from Amersham Biosciences (Sweden). 4-tert-Butyl-4′-methoxydibenzoylmethane (also known as avobenzone and Parsol 1789, C20H22O3), a commercial sunscreen filter was from King Jack Int., Hong Kong. The buffer used was phosphate type at pH 7.4 (25 °C). Other reagents were the best grade commercially available, which were distilled, recrystallized or used with further purification as appropriate. All reactions were conducted under dry N2 unless otherwise stated.
2.1. Lipid synthesis
The synthetic routes of the cholesterol derivatives containing an azobenzene moiety are given in Scheme 1.23
2.2. Liposome preparation
The preparation of small unilamellar liposomes (SUV) was carried out using a standard sonication method under nitrogen. Briefly, stock lipids in CHCl3 were transferred from their stock solutions into a flask. The solutions were subsequently rotary evaporated under low vacuum to produce a thin film of lipid. The samples were then stored under vacuum for 1 h at room temperature. The thin lipid film formed on the wall of flask was hydrated with a 0.15 M phosphate buffered saline solution (PBS, pH 7.4) and sonicated under nitrogen for 14 min (2 min on and 5 s off for each cycle) with a VC 130 Probe Sonicator at maximum power output (Sonics & Materials, Inc.) with the temperature controlled using an ice–water bath. The total lipid concentration was 0.1 mM. Subsequent centrifugation at 100
000g was carried out to remove untrapped lipid and titanium. For release measurements, the thin lipid film was hydrated with 10 mg mL−1L-ascorbic acid or 1 mg mL−1 catalase at pH 7.4 before sonication.
2.3. Size distribution of CDBA-liposome
Liposomal solution was diluted with PBS (pH 7.4) and the size distribution (based on the intensity of particles) was determined using an MALVERN Nano-s (UK), equipped with a computer-controlled image analysis system.
2.4. Photoisomerization of CDBA lipid in chloroform
Diluted CDBA lipid in CHCl3 was irradiated with a 400 W high-pressure Hg lamp (LCE-9, Zhengzhou, China). A bandpass filter (λT = 275–400 nm) was used for UV light. After a certain period, the UV spectrum of the liposome dispersion was measured using a UV-Vis spectrophotometer (U3010, HITACHI). Similarly, the UV spectra of the CDBA lipid was measured in the same way after incubation at 37 °C for given times.
2.5. Photoisomerization of CDBA combined liposome
A diluted CDBA/egg PC mixed liposomal dispersion was irradiated as described above and the UV spectrum was measured.
Accurately 1 mg of standard ascorbic acid (99.9% purity) was weighed and dissolved in 100 ml of 0.15 M phosphate buffered saline solution (pH 7.4) to obtain a stock concentration of 100 μg mL−1. Aliquots of standard ascorbic acid were diluted to required concentrations ranging from 1.0 to 40.0 μg mL−1. Working standard solutions were prepared in triplicate. Ascorbic acid was measured by oxidation with activated carbon to form dehydroascorbic acid type (D-ascorbic acid), then o-phenylenediamine (OPDA) was added to form a fluorescent quinoxaline, its fluorescence intensity was directly proportional to the total amount of ascorbate. The standard curve was constructed by plotting the mean of the fluorescence intensity of quinoxaline (GB 5413.18—2010, National Food Safety Standard of China: Determination of vitamin C in foods for infants and young children, milk and milk products).
2.7. Determination of liposome encapsulated ascorbic acid
Liposome encapsulated ascorbic acid was calculated by the equation:
where W1 is the amount of unentrapped L-ascorbic acid which was separated by gel filtration on Sephadex G-50 column using above PBS buffer as the eluent and W2 is the original weight of 10 mg mL−1 ascorbic acid when preparing liposomes.
2.8. Stability of L-ascorbic acid in CDBA-liposome
L-Ascorbic acid is susceptible to oxidation to form D-ascorbic acid under UV irradiation. The stability of ascorbic acid was monitored by quantification of D-ascorbic acid which was contained in the liposomal solution. Stability of free ascorbic acid and that in liposomes was compared after UV irradiation for 2, 4, 6, 8 h.
2.9. Ascorbic acid released from CDBA-liposome
The release behavior of ascorbic acid was observed by monitoring the release of D-ascorbic acid from CDBA-liposome. The liposomal dispersion was added to PBS buffer. After UV irradiation for 1 h and then placing in incubator at 37 °C for 2 h, the D-ascorbic acid released from liposomes was measured by the fluorescence method as described in section 2.6.
2.10.
In vivo test for assessing UV protection
A number of methods have been proposed to evaluate the UV protection level of sunscreens in vivo.24 Since highly invasive end points such as those requiring biopsy clearly were not suitable for assessing photoprotection provided by commercial sunscreen products, in vivo test methods were primarily based on less invasive end point measurements, namely erythema and ambustion. The UV source for in vivo testing consisted of a 400 W high-pressure mercury lamp (LCE-9, Zhengzhou, China) attached a filter cutting out the wavelength above 400 nm. Four test sites (4 cm2) were selected on the back of rats (Scheme 2). The sites were located between breast and scapulae and lateral to the midline. The test samples were applied at an amount of 20 μl cm−2 (0.06 mg CDBA) and spread uniformly over each test site using a finger cot and then allowed to dry for 15 min before starting the UV exposure. On each test site, four squares (1 cm × 1 cm) were defined, on an unprotected skin area of the rat dorsal and on a sunscreen-protected area. At a distance of 15 cm from the light source, the time of illumination was 5 h on the test sites.
2.11. Enzyme activities of free and liposomal catalase
Catalase activity was determined as an enzyme kinetic assay by the disappearance of H2O2. For catalase-containing liposomes (CAL), aliquots of the CAL suspension were added to the PBS solution containing 15 mM H2O2 to give overall catalase concentrations of 0.1–0.2 μg ml−1. Then the time course of the decomposition of H2O2 was followed at 25 °C based on the absorbance of H2O2 at 240 nm using a UV-Vis spectrophotometer (U3010, HITACHI). The initial decomposition rate was taken as the apparent activity of the liposomal catalase. The intrinsic catalase activity of the CAL was measured in the same way as described above except that the reaction solution contained Triton X-100 for complete solubilization of catalase from liposomes. PC, cholesterol, CDBA and Triton X-100 had negligible effects on the above activity measurement. For free catalase, its activity was measured in the same way as above at catalase concentrations of 0.1–0.2 μg mL−1.
2.12. Catalase released from CDBA-liposome
The release behavior was observed by monitoring the release of catalase from CDBA-liposome. The liposomal dispersion was added to PBS buffer. After UV irradiation, catalase released from liposomes was measured by the method described above.
2.13. Stability of catalase in CDBA-liposome
The stability behavior was observed by monitoring catalase activity in the liposomal solution. Stability of free catalase and that in liposomes was compared under UV irradiation for 0, 1, 3, 5 h.
3. Results and discussion
3.1. Size distribution of CDBA-liposome
Particle size analysis showed that the CDBA-liposome, with an average diameter of 143.4 ± 2.0 nm (n = 3, PDI = 0.248 ± 0.010), was rather homogeneous in size, indicating that most of them were in the form of SUV (Fig. 1).
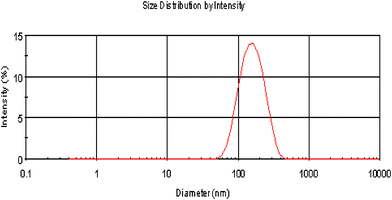 |
| Fig. 1 Particle distribution of the CDBA-liposome. | |
3.2. Photoisomerization of CDBA in chloroform
It is known that azobenzene derivatives in CHCl3 solution undergo trans-to-cis isomerization by UV irradiation and cis-to-trans isomerization by visible irradiation. CDBA lipid were irradiated with UV light and the UV-Vis spectra are shown in Fig. 2 (left). Data for samples placed in an incubator at 37 °C are shown in Fig. 2 (right). This trans–cis isomerization could be repeated at least three times.
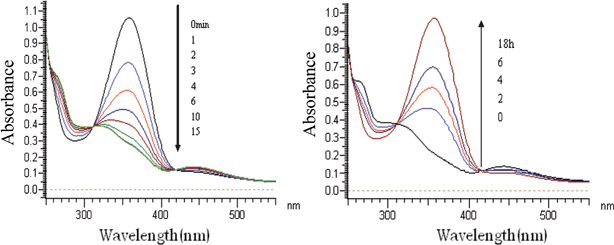 |
| Fig. 2 UV-Vis spectral change of CDBA lipids in chloroform following UV irradiation (left) or incubation at 37 °C (right) for different times. | |
3.3. Photoisomerization of CDBA lipids in liposomes
Liposomes incorporated with CDBA lipid were irradiated with UV light and the UV-Vis spectra are shown in Fig. 3 (left) while data for samples placed in an incubator at 37 °C are shown in Fig. 3 (right). trans-CDBA lipid in liposomes converted to cis-CDBA lipid as evidenced from the decrease of a strong peak at 360 nm and the concurrent increase of a weak peak at 450 nm. It was estimated from the spectral change that 65% of trans-CDBA lipid in liposomes was converted into cis-CDBA lipid and reached a photostationary state after 30 min of UV irradiation; while in CHCl3 solution, it only took 15 min of UV irradiation to convert 90% of trans-CDBA lipid into cis-CDBA lipid (Fig. 2 and 3). Thus the rate and ratio of trans-to-cis isomerization by UV irradiation was slower and lower in liposomes than in CHCl3 solution, implying that to retard photoisomerization, CDBA lipid should be incorporated into liposomes. It was likely that trans-to-cis isomerization, along with enlargement of bulk structure of the CDBA lipid molecule, was considerably suppressed in the lipid membrane. When incubated at 37 °C, the rate of cis-to-trans isomerization of CDBA lipid in liposomes was very slow. We confirmed that this trans–cis isomerization could be repeated for at least three times.
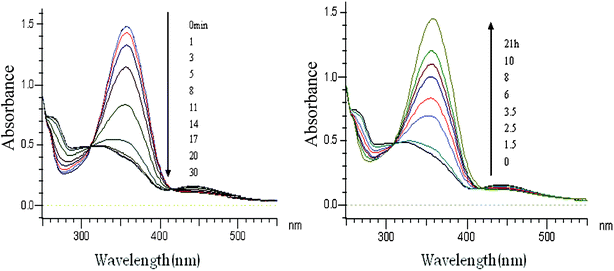 |
| Fig. 3 UV-Vis spectral change of CDBA-liposome following UV irradiation (left) or incubation at 37 °C for different times. | |
3.4. Determination of liposome encapsulated ascorbic acid
The linearity of the fluorescence method towards ascorbic acid concentration was studied over a concentration range of 1.0–40.0 μg mL−1. A standard curve was constructed according to the fluorescence intensity of ascorbic acid and OPDA to form quinoxaline. The result was satisfactory. Least-squares regression analysis was used to evaluate the concentration range that showed excellent linearity over the interval studied with r2 ≥ 0.99. Linear regression was y = 0.006x − 0.467, where y was the concentration of ascorbic acid (μg mL−1) and x was the fluorescence intensity of quinoxaline.
As determined by D-ascorbic acid activity assay, an average encapsulation efficiency of ascorbic acid of ca. 25.0%, was obtained with liposomes prepared by the standard sonication method under nitrogen. With the same method, Zhao et al. reported that the highest encapsulation rate of Vc-loaded liposome was 18.1%.25 Liang et al. reported that the highest encapsulation rate of Vc-loaded liposome was 10.0%.26 Therefore, the encapsulation rate of Vc-loaded liposome with CDBA was higher than those reported previously.
3.5. Stability of L-ascorbic acid in CDBA-liposome
In aqueous solution L-ascorbic acid is decomposed by environmental oxygen and other oxidants leading to dehydroascorbic acid. This last stage of oxidation is irreversible and biological activities are already compromised.27 Therefore, for protection by L-ascorbic acid, it must not be oxidized. L-Ascorbic acid in CDBA-liposome was obviously much more stable than its free state, as shown in Fig. 4. As a result, CDBA-liposome could protect L-ascorbic acid from decomposition and oxidation.
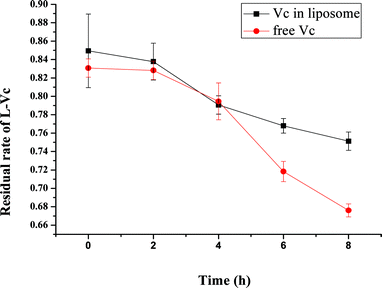 |
| Fig. 4 Stability of L-ascorbic acid in CDBA-liposome and its free state after UV irradiation for various periods. Each point represents the mean ± SD (n = 3). | |
3.6. Photo-controlled release from CDBA-liposome
An important factor for controlling on–off release system is to provide a perfect off state. Although there was a very small amount of ascorbic acid released from liposomes spontaneously, the amount was dependent on the lipid used in the liposomes. The release rate could be suppressed by placing the liposome in an incubator at 37 °C. As shown in Fig. 5, ascorbic acid was released upon UV irradiation and this photo-triggered release could be suppressed by incubation at 37 °C. This is thus an intelligent on–off release system. The decrease of ascorbic acid in the third cycle might be due to that most of the ascorbic acid that was encapsulated was released upon previous UV irradiation. Another possible reason may be that D-ascorbic acid could degrade at 37 °C,28 so decreasing the fluorescence intensity.
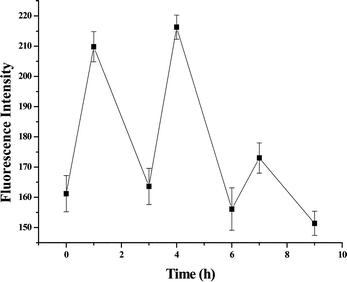 |
| Fig. 5 Release of ascorbic acid from CDBA-liposome upon UV irradiation for 1 h and then incubation at 37 °C for 2 h. Each point represents the mean ± SD (n = 3). | |
3.7. Spontaneous release from liposomes
Liposome (PC+Chol) and CDBA-liposome were placed in an incubator at 37 °C, and ascorbic acid concentrations were measured at periodic intervals. Fig. 6 shows that the spontaneous release rate in PC liposome was faster than CDBA-liposome. Thereby, CDBA lipid in liposome had a function that could prohibit the spontaneous release, probably due to its cholesterol structure.
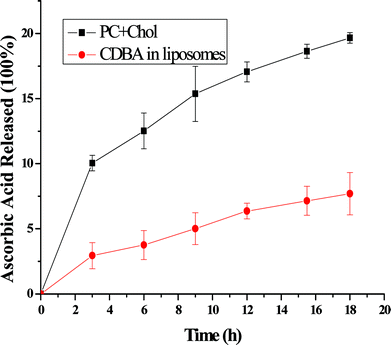 |
| Fig. 6 Spontaneous release rates from liposomes at 37 °C. Each point represents the mean ± SD (n = 3). | |
3.8.
In vivo test for assessing UV protection
According to the absorbency spectrum and Lambert–Beer law, the apparent molar absorptivity of CDBA lipid in chloroform was 19
913 L mol−1 cm−1 at the maximum absorption of 357 nm, and apparent molar absorptivity of avobenzone in chloroform was 44
280 L mol−1 cm−1 at the maximum absorption of 358 nm. To compare their effects of UV protection, samples with the same absorption were applied for in vivo testing. The four test sites (Scheme 2) were coated separately with liposome (PC+Chol), CDBA lipid in liposome, avobenzone lipid in liposome and avobenzone only, on the backs of rats. In vivo test methods were primarily based on erythema and ambustion, so the UV protection was assessed after UV irradiation for periodic intervals; results are shown in Fig. 7.
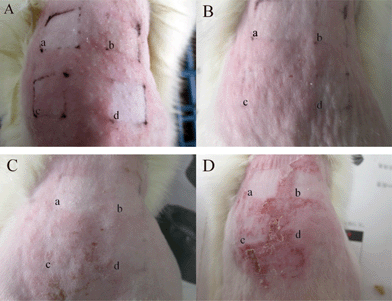 |
| Fig. 7 The skin states of erythema and ambustion after UV irradiation for 5 h and then studied after 0 (A), 1 (B), 2 (C) and 3 days (D): a: avobenzone-liposome; b: CDBA-liposome; c: PC-liposome (PC+Chol); d: avobenzone. | |
As seen from Fig. 7A, after UV irradiation for 5 h, the surrounding area for PC-liposome showed much more obvious erythema than that of coating with CDBA-liposome, avobenzone-liposome and avobenzone. One day after the UV irradiation, erythema was not so obvious as seen from Fig. 7B. From Fig. 7C, two days after the UV irradiation, erythema continued to become even more inconspicuous than before, but festering became apparent. From Fig. 7D, three days after the UV illumination, festering was not observed with CDBA-liposome and avobenzone-liposome, while skin coated with PC-liposome and avobenzone showed very serious festering. Therefore both CDBA and avobenzone displayed sun protection.
3.9. Catalase release from CDBA-liposome upon UV irradiation
Catalase can be encapsulated in the aqueous phase of the CDBA-liposome as a biomacromolecule, and the mean entrapment efficiency was 14%. As shown in Fig. 8 (left), upon continuous UV irradiation, entrapment efficiency decreased gradually, which indicated that CDBA-liposome can encapsulate the biomacromolecule which can be released from the CDBA-liposome with a photo-triggered event.
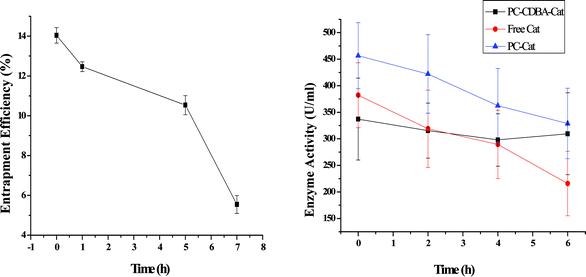 |
| Fig. 8 Release of catalase from CDBA-liposome (left); stability of free catalase and catalase in liposomes under UV irradiation for various times (right). Each point represents the mean ± SD (n = 3). | |
3.10. Stability of catalase in CDBA-liposome
Fig. 8 (right) shows the remaining catalase activities of PC-liposome, CDBA-liposome and free catalase. After continuous UV irradiation for 6 h, catalase activities of PC-liposome, CDBA-liposome and free catalase decreased by 23.5, 11.1 and 44.3%, respectively. Accordingly, catalase in liposomes was much stable than its free state towards UV irradiation, and catalase in CDBA-liposome was the most stable in all.
4. Conclusion
It has been shown that Vc-loaded CDBA-liposome could undergo trans-to-cis isomerization by UV irradiation and cis-to-trans isomerization by incubation at 37 °C. Furthermore, this trans-cis isomerization could be repeated several times. Both L-ascorbic acid and catalase in CDBA-liposome were obviously much stable than their free states. An intelligent release of ascorbic acid from CDBA-liposome could be achieved by external stimuli such as UV irradiation and temperature. Moreover, CDBA-liposome was a good sunscreen product due to the absorption ability in the UV range of CDBA, which implies possible commercial application as a sunscreen cosmetic.
Acknowledgements
The authors gratefully acknowledge the financial support provided by Shanghai Qin-Lin Investment Co., Ltd; the National Natural Science Foundation of China (30870635, 31070868); the Chinese Academy of Sciences (KGCX2-SW-602-5); and the Medical-Engineering Cross Project of Shanghai Jiaotong University (YG2010MS69).
References
- P. Kullavanijaya and H. W. Lim, Photoprotection, J. Am. Acad. Dermatol., 2005, 52, 937–958 CrossRef.
- D. Moyal, A. Chardon and N. Kollias, UVA protection efficacy of sunscreens can be determined by the persistent pigment darkening (PPD) method, Photodermatol. Photoimmunol. Photomed., 2000, 16, 250–255 CAS.
- R. M. Lavker, D. Veres, C. J. Irwin and K. H. Kaidbey, Cumulative effects from repeated exposures to suberythemal doses of UVB and UVA in human skin, J. Am. Acad. Dermatol., 1995, 32, 53–62 CrossRef CAS.
- N. P. Mahesh and B. P. Varsha, Development of vitamin loaded topical liposomal formulation using factorial design approach: Drug deposition and stability, Int. J. Pharm., 2006, 320, 37–44 CrossRef.
- R. Kohen, Skin antioxidants: Their role in aging and in oxidative stress -New approaches for their evaluation, Biomed. Pharmacother., 1999, 53, 181–192 CrossRef CAS.
- M. Podda, M. G. Traber, C. Weber, L. J. Yan and L. Packer, Free radicals, UV-irradiation depletes antioxidants and causes oxidative damage in a model of human skin, Free Radic. Biol. Med., 1998, 24, 55–65 CrossRef CAS.
- H. Freiberger, D. Grove, A. Sivarajah and S. R. Pinnel, Procollagen I synthesis in human skin fibroblasts: effect of culture conditions on biosynthesis, J. Invest. Dermatol., 1980, 75, 425–430 CrossRef CAS.
- S. Murad, D. Grove, K. A. Lindberg, G. Reynolds, A. Sivarajah and S. R. Pinnell, Regulation of collagen synthesis by ascorbic acid, Proc. Natl. Acad. Sci. U. S. A., 1981, 78, 2879–2882 CrossRef CAS.
- R. Austria, A. Semenzato and A. Bettero, Stability of vitamin C derivatives in solution and topical formulations, J. Pharm. Biomed. Anal., 1997, 15, 795–801 CrossRef CAS.
- M. Gallarate, M. E. Carlotti, M. Trotta and S. Bovo, On the stability of ascorbic acid in emulsified systems for topical and cosmetic use, Int. J. Pharm., 1999, 188, 233–241 CrossRef CAS.
- M. R. Lowik, J. Schrijver and M. Wedel, Vitamin C analysis in whole blood, plasma and cells using reduced glutathione as preservative (stabilizer): losses and redistribution, Int. J. Vitam. Nutr. Res., 1990, 61, 43–45 Search PubMed.
- E. De Ritter, Vitamins in pharmaceutical formulations, J. Pharm. Sci., 1982, 71, 1073–1096 CrossRef CAS.
- C. Chen, T. D. Johnstona and H. Jeon, An in vitro study of liposomal curcumin: Stability, toxicity and biological activity in human lymphocytes and Epstein-Barr virus-transformed human B-cells, Int. J. Pharm., 2009, 366, 133–139 CrossRef CAS.
- G. Ioele, E. Cione and A. Risoli, Accelerated photostability study of tretinoin and isotretinoin in liposome formulations, Int. J. Pharm., 2005, 293, 251–260 CrossRef CAS.
- P. Chelikani, I. Fita and P. C. Loewen, Diversity of structures and properties among catalases, Cell. Mol. Life Sci., 2004, 61, 192–208 CrossRef CAS.
- B. L. Diffey, R. P. Stokes, S. Forestier, C. Mazilier and A. Rougier, Suncare product photostability: a key parameter for a more realistic in vitro efficacy evaluation, Eur. J. Dermatol, 1997, 7, 226–228 Search PubMed.
- S. P. Huong, V. Andrieu, J. P. Reynier, E. Rocher and J. D. Fourneron, The photoisomerization of the sunscreen ethylhexyl p-methoxy cinnamate and its influence on the sun protection factor, J. Photochem. Photobiol. A, 2007, 186, 65–70 CrossRef CAS.
- G. Ioele, E. Cione, A. Risoli, G. Genchi and G. Ragno, Accelerated photostability study of tretinoin and isotretinoin in liposome formulations, Int. J. Pharm., 2005, 293, 251–260 CrossRef CAS.
- B. M. Baughman, E. Stennett, R. E. Lipner, A. C. Rudawsky and S. J. Schmidtke, Structural and spectroscopic studies of the photophysical properties of benzophenone derivatives, J. Phys. Chem. A, 2009, 113, 8011–8019 CrossRef CAS.
- P. Supason, M. Thitinun, H. Piyawan and L. Pamornwan, Photoisomerization of octyl methoxycinnamate, J. Photochem. Photobiol. A, 2004, 161, 269–274 CrossRef.
- X. M. Liu, B. Yang, Y. L. Wang and J. Y. Wang, Photoisomerisable cholesterol derivatives as photo-trigger of liposomes: Effect of lipid polarity, temperature, incorporation ratio, and cholesterol, Biochim. Biophys. Acta, Biomembr., 2005, 1720, 28–34 CrossRef CAS.
- X. M. Liu, B. Yang, Y. L. Wang and J. Y. Wang, New nanoscale pulsatile drug delivery system, Chem. Mater., 2005, 17, 2792–2795 CrossRef CAS.
- B. Yang, S. Y. Geng, X. M. Liu, J. T. Wang, Y. K. Chen, Y. L. Wang and J. Y. Wang, Positive cholesterol derivative combined liposome as an efficient drug delivery system, in vitro and in vivo study, Soft Matter, 2012, 8, 518–525 RSC.
- D. Moyal, How to measure UVA protection afforded by sunscreen products, Expert Rev. Dermatol., 2008, 3, 307–313 CrossRef CAS.
- H. Zhao, Y. m. Dong and C. F. He, The research of Vc liposome manufacture, Flavour Frag. Cosmet., 2006, 3, 17–20 Search PubMed.
- Y. Z. Liang and L. Bao, Synthesis of embedded Ve and Vc of lecithin liposomes, Daily Chem. Ind., 1994, 3, 172–175 Search PubMed.
- M. R. Lowik, J. Schrijver and M. Wedel, Vitamin C analysis in whole blood, plasma and cells using reduced glutathione as preservative (stabilizer): losses and redistribution, Int. J. Vitam. Nutr. Res., 1990, 61(1), 43–45 Search PubMed.
- A. M. Maia, A. R. Baby and W. J. Yasaka, Validation of HPLC stability-indicating method for Vitamin C in semisolid pharmaceutical/cosmetic preparations with glutathione and sodium metabisulfite, as antioxidants, Talanta, 2007, 71(2), 639–643 CrossRef CAS.
Footnote |
† These authors contributed equally to this work. |
|
This journal is © The Royal Society of Chemistry 2012 |
Click here to see how this site uses Cookies. View our privacy policy here.