DOI:
10.1039/C2RA20302B
(Paper)
RSC Adv., 2012,
2, 4110-4119
Vitamin C-reinforcing silk fibroin nanofibrous matrices for skin care application
Received
17th February 2012
, Accepted 20th February 2012
First published on 16th March 2012
Abstract
In this work, we reported on the preparation and skin benefits of L-ascorbic acid 2-phosphate (VC-2-p)-loaded silk fibroin (SF) nanofibrous matrices for the first time. The matrices was fabricated using a facile eco-friendly electrospinning processing. With a post treatment of 75 v/v% ethanol vapor, the structure of the matrices transformed from unstable silk I form into water-stable silk II. In vitro release studies confirmed VC-2-p disassociated from SF nanofibrous matrices easily. Both neat and VC-2-p-loaded SF nanofibrous matrices were beneficial to mouse fibroblast L929 cells (L929 cells) adhering, spreading and proliferating against cover slips. Whereas compared with neat SF nanofibrous matrices, VC-2-p-loaded SF nanofibrous matrices significantly promoted the expression of collagen type I alpha 1 (Col1a1), as evidenced by real time PCR. Subsequently, the oxidative injury model further verified both matrices aided L929 cells through antioxidation to survive from tert-butyl hydroperoxide-induced oxidative stress (OS). Importantly, under severe OS, L929 cells on VC-2-p-loaded SF nanofibrous matrices maintained a higher mRNA level of Col1a1 as well as another two important functional genes, glutathione peroxidase 1 and catalase, than neat SF nanofibrous matrices. Our findings clearly indicated that the impressive skin benefits of SF nanofibrous matrices were further reinforced with the incorporation of VC-2-p, which implies the promising application of VC-2-p-loaded SF nanofibrous matrices in personal skin care and skin regeneration, including serving as wound dressings and anti-aging materials.
Introduction
Skin is an essential natural barrier protecting the organism from multifarious injuries or damage, however, its functions are continuously disturbed by a host of evitable stress factors such as UV radiation and environmental pollutants, which lead to varied skin disorders or diseases including skin aging and skin cancer.1–3 To restore those functions of the skin barrier, an extensive diversity of skin care products has already been developed and caring for skin has already became routine for a large population.
For a long time L-ascorbic acid (vitamin C, VC), with remarkable skin benefits, has been reassessed with repeated references in the literature.4–11 VC has numerous impressive skin care functions such as enhancing the expression of collagens, promoting wound healing, decreasing melanin and so forth.4–9 Furthermore, as a pivotal physiological antioxidant, it can effectively quench or react with diverse oxygen radical species, and aid cells to survive in oxidative stress (OS).4,5,10,11 Nonetheless, humans have failed to synthesize VC, which captures growing attention of researchers, rendering it the target of various skin care formulations.9,12,13
As well as VC, silk fibroin (SF) from Bombyx mori is another beneficial factor of human health and especially, it is favored by skin cells or tissues.14–18 There exists a number of reports on its skin benefits: it's able to stimulate the biosynthesis of collagen protein, promote re-epithelializing, accelerate wound healing, and helps to alleviate atopic dermatitis and eliminate scarring;16–18 it is also capable of maintaining an aqueous environment for skin with a mechanism similar to natural moisturizing factors of the skin’s own moisturizing system, which is important for improving skin texture and promoting nutrient ingredient assimilation.5,19 Furthermore, SF is also a promising biomaterial for skin regeneration and possesses some unique properties such as impressive biocompatibility and biodegradability, favorable oxygen and water vapor permeability, low immunogenicity etc.14,15 Recently, advantages of SF nanofibrous matrices in biomedical field have been widely reported, whereas perhaps less obvious is its tremendous potential in personal skin care.14,15,20,21
Therefore, we hypothesize that VC-loaded SF nanofibrous matrices could be powerful in personal skin care or skin regeneration, as it not only integrates SF with VC skillfully, but also provides a specifically high level of surface area for cell attachment and growth. To test the hypothesis, L-ascorbic acid 2-phosphate (VC-2-p)-loaded SF nanofibrous matrices (we used VC-2-p instead of VC in this work, since VC-2-p was reported to function much better as a bioactive factor due to its excellent stability22) were developed using a facile eco-friendly electrospinning processing reported in our previous studies.21 The morphology and structure of nanofibrous matrices were investigated using scanning electronic microscopy (SEM) and 13C nuclear magnetic resonance (13C-NMR), and the release profile of VC-2-p from SF nanofibrous matrices was determined with high performance liquid chromatography (HPLC). On the top of them, the skin benefits of VC-2-p-loaded SF nanofibrous matrices were confirmed using SEM, 3-[4,5-dimethylthiazol-2-yl]-2,5-diphenyl tetrazolium bromide (MTT) assay, real time PCR and the oxidative injury model in vitro. The present work could provide a basis for further studies or practical applications in skin care or skin regeneration.
Experimental
Materials
The cocoon of Bombyx mori silkworm was a generous gift from Jiaxing Silk Co. (China). VC-2-p in the form of L-ascorbic acid 2-phosphate trisodium salt: C6H6Na3O9P·xH2O (purity ≥ 95.0%, HPLC), and tert-butyl hydroperoxide (t-BHP) were purchased from Sigma-Aldrich China Inc. Acetonitrile was from J&K Scientific Ltd (China). Mouse fibroblast L929 cells (L929 cells) were provided by the Institute of Biochemistry and Cell Biology (Chinese Academy of Sciences, China). All other reagents involved in this study were of analysis grade or higher. Ultrapure water (Rephile Shanghai Bioscience & Technology Co., Ltd., China) was used throughout the whole study.
Preparation of regenerated SF (RSF)
RSF sponge was fabricated as stated in our previous studies.21 Briefly, the cocoon was boiled three times in a 0.5% (w/w) Na2CO3 aqueous solution (20–30 min each time, based on the quality of the cocoon) and then extensively rinsed with warm ultrapure water to prepare sericin protein-free silk. Following drying at 40 °C, degummed silk was dissolved in a ternary solvent system of CaCl2/H2O/CH3CH2OH solution (molar ratio 1
:
8
:
2) at 65 °C to a clear solution. Then RSF sponge was obtained by lyophilizing, after dialyzing in ultrapure water with cellulose tube (molecular weight cutoff 14
000 Da, Jing Ke Hong Da Biotechnology Co., Ltd., China) at ambient temperature for 3 days.
Fabrication of nanofibrous matrices
RSF sponge and VC-2-p were dissolved in ultrapure water, and constantly incubated into clear solutions under slight agitation with a magnetic stirrer. The homogeneous VC-2-p-containing SF solution was obtained by adding SF solution to VC-2-p solution under constant stirring. The final concentration of SF ranged from 20 to 35 wt%, and VC-2-p was in the range of 1 and 3 wt% based on the weight of SF, according to the previous studies.7,8,22 Then, the VC-2-p/SF solution was filled into a 2.5 mL plastic syringe capped with a 27-gauge blunt needle (ID = 0.21 mm). The syringe was located in a syringe pump (Model 789100C, Cole-Parmer Instrument Co., USA) with a rate of 0.3 mL h−1. A voltage of 20 kV provided by a high voltage power supply unit (BGG6-358, BMEI CO., LTD., China) was applied to the needle, with a ground aluminum foil as the collector. The distance between spinneret and collector was 20 cm. After vacuum-drying, the obtained VC-2-p-loaded SF nanofibrous matrices were treated under an atmosphere of 75 v/v% ethanol vapor and allowed to dry in a fume hood for further studies. (Briefly, 75 v/v% ethanol vapor-treated samples were prepared by placing nanofibrous matrices in a sealed desiccator saturated with 75 v/v% ethanol vapor at ambient temperature. The treatment time ranged from 24 h to 96 h, which depended on the thickness of nanofibrous matrices.21) Meanwhile, a series of neat SF nanofibrous matrices were also prepared as controls under the same conditions.
Determining the actual amount of VC-2-p loaded in the samples
Different samples with treatment of 75 v/v% ethanol vapor were decomposed with proteinase K solution (0.5 mg mL−1 in 12.5 mM Tris-HCL buffer solution, pH 8.0) at 37 ± 0.5 °C, respectively. Each of resulting solutions was assayed using HPLC. The actual amount of VC-2-p in each sample was determined from the obtained data against a predetermined calibration curve for VC-2-p.
HPLC analysis was performed using Varian 920-LC series HPLC system (Varian, USA) with a C18 column (particle size = 5 μm; column dimension = 250 × 4.6 mm). The mobile phase was 55% acetonitrile with 10 mM phosphilic acid (flow rate 0.8 mL min−1) and it was detected at 245 nm, based on the previous studies.23
Characterization of nanofibrous matrices
The morphology of nanofibers was inspected using SEM (JSM-5600LV, Japan Electron Optical Laboratory). The sample was sputter-coated with a thick gold film prior to the observation. The average fiber width was determined from 60 random measurements on a typical SEM image using Image-J 1.34 software (National Institutes of Health, USA). 13C-NMR analysis was carried out at room temperature using NMR spectrometry (Bruker AV400) with a 13C resonance frequency of 100 MHz, a contact time of 1.0 ms and a pulse delay time of 4.0 s.
Release of VC-2-p from VC-2-p-loaded SF nanofibrous matrices
To investigate the cumulative release profile of VC-2-p from VC-2-p-loaded SF nanofibrous matrices in vitro, samples were incubated in ultrapure water (pH 7.0) with a continuous swing of 80 rpm at 32 ± 0.5 °C respectively. After specified time-intervals, 1 mL of the release solution was withdrawn and an equal volume of fresh ultrapure water was refilled to maintain a constant volume. The amount of VC-2-p in the release solution was measured using HPLC (as described above) against a predetermined calibration curve for VC-2-p. The obtained data were used to calculate the cumulative amount of VC-2-p released from samples into ultrapure water at each specific time point.
Cell culture and seeding
As described in our previous studies,21 L929 cells were cultured in RPMI 1640 medium (Gibco) supplemented with 10% fetal bovine serum (Gibco) and incubated in a humidified incubator at 37 °C, with 5% CO2. To assess the effects of nanofibrous matrices on L929 cells, neat and VC-2-p-loaded SF nanofibrous matrices were collected on circular cover slips (14 mm in diameter) for 12 h. After treatment with 75 v/v% ethanol vapor, nanofiber-deposited cover slips were placed into 24-well cell culture plate and fixed with autoclaved steel rings, herein, nanofiber-free cover slips as controls. L929 cells were directly plated on nanofibrous matrices and cover slips in the 24-well cell culture plate at the same density of 1.2 × 104 cells/well. Initially, the volume of cells and medium was 400 μL for each well, and then 200 μL of fresh medium was added to each well every 3 days.
Cell proliferation and morphology
L929 cells were allowed to proliferate on nanofibrous matrices and cover slips for 1, 3, 5 and 7 days. At these specific time point, cell-seeded or unseeded nanofibrous matrices and cover slips were rinsed with phosphate-buffered saline (PBS) three times following the removal of the medium. Thereafter, each well was supplemented with 400 μL of serum-free Dulbecco's modified Eagle's medium (DMEM) (Gibco) containing 0.5 mg ml−1 MTT (Sigma-Aldrich). After being cultured for 4 h, the medium was completely aspirated and the formazan formed was dissolved in 400 μl of dimethylsulfoxide. Then 100 μL of the solution was withdrawn to measure the absorption at 492 nm with a microplate reader (Multiskan MK3; Thermo Labsystems Co., China).
Following incubation for 5 days, the cultures were harvested, washed with PBS and then fixed with 4% paraformaldehyde for 20 min at 4 °C. After three rinses with PBS, fixed samples were consecutively exposed to 30, 50, 70, 80, 90, 95 and 100% ethanol solutions, and then dried in a fume hood. Dry samples were observed using SEM following being sputter-coated with a gold film.
Detection of the mRNA level of some functional genes in the cells on the matrices
Total RNA was extracted from the cells after 7 day culture on the matrices, using RNAprep pure Cell/Bacteria Kit (TIANGEN BIOTECH CO., LTD., Beijing, China) and the reverse transcription reactions were conducted using RevertAid™ First Strand cDNA Synthesis Kit (Fermentas) according to the manufacturer's protocol. Real time PCR was then carried out using Maxima® SYBR Green/ROX qPCR Master Mix (Fermentas) on a ROTOR-GEN3000A analysis system (Corbett Research). The primer sequences for collagen type I alpha 1 (Col1a1), glutathione peroxidase 1 (Gpx1) and catalase (Cat) genes are listed in Table 1. Reactions were performed in triplicate under the following conditions: 95 °C for 10 min followed by 40 cycles of 95 °C for 15 s, 60 °C for 30 s, 72 °C for 30 s. Relative changes in gene expression were calculated in relation to beta-actin (Actb) expression levels using the 2−ΔCt method.24
Table 1 The sequences of the primers used in real-time PCR
Gene name (Accession No.) |
Primer sequencea (5′→3′) |
Intron-Exon boundry |
Product size (bps) |
The intron-exon boundry is indicated with bold in the primer sequence.
|
Actb
|
ATCGTGCGTGACATCAAAGAGAAGC |
NO |
200 |
(NM_007393.3) |
ATGCCACAGGATTCCATACCCAAGA |
YES |
Col1a1
|
GGACACCGAGGCTTCAG |
YES |
195 |
(NM_007742.3) |
AGCACCAACAGCACCAT |
NO |
Cat
|
AGTCTCTCCATCAGGTTTCTTTCT |
YES |
186 |
(NM_009804.2) |
GCCTTCCTGCCTCTCCAA |
NO |
Gpx1
|
GCAATCAGTTCGGACACCAGGAG |
YES |
128 |
(NM_008160.5) |
CTCACCATTCACTTCGCACTTCTCAA |
NO |
Antioxidation assay
To further assess the skin benefit of nanofibrous matrices, the viability assay of cells cultured on the matrices under OS was conducted as follows. After cells were cultured for 6 days on the matrices, t-BHP was added with final concentrations of 0, 50, 100 and 200 μM respectively. Following 24-hour treatment of t-BHP, the cell viability was determined using the MTT method and the mRNA level of the functional genes was detected using the same protocol described above.
Statistical analysis
All experiments were conducted at least three times and data were reported as mean ± standard deviation (SD). Statistical analysis was conducted on an Origin 7.5 (OriginLab, USA) using one-way ANOVA. In all statistical comparisons, a p-value of less than 0.05 was considered statistically significant. The error bars in the figures are the SD of the data.
Results and discussion
Morphology of nanofibrous matrices
To optimize the concentration of electrospinning solution, VC-2-p-loaded SF nanofibers (concentration of VC-2-p was 3 wt% based on the weight of SF) were fabricated with different concentrations (20, 25, 30 and 35 wt%) of SF. Along with the increasing SF concentration, the average width (AW) of as-spun fibers (n = 60) increased gradually, as shown in the width distribution histograms (Fig. 1), whereas fibers cannot be obtained via electrospinning at a low (≤15 wt%) or high (≥40 wt%) concentration of SF, due to the low or high viscosity of solution. At a SF concentration of 20 wt%, the as-prepared nanofibers displayed bead-fiber morphology (Fig. 1a). Obviously, when the concentration of SF was up to 25 wt%, the resulting ribbon-like fibers had a relatively homogeneous morphology with a smooth surface (Fig. 1b). Therefore, the 25 wt% aqueous SF solution was selected as the basic solution, into which different amounts of VC-2-p were individually added. Hereinafter, neat and VC-2-p-loaded SF nanofibers (concentration of VC-2-p was 1 and 3 wt% based on the weight of SF respectively) were denoted as 25%SF, 1%VC-2-p-25%SF and 3%VC-2-p-25%SF respectively.
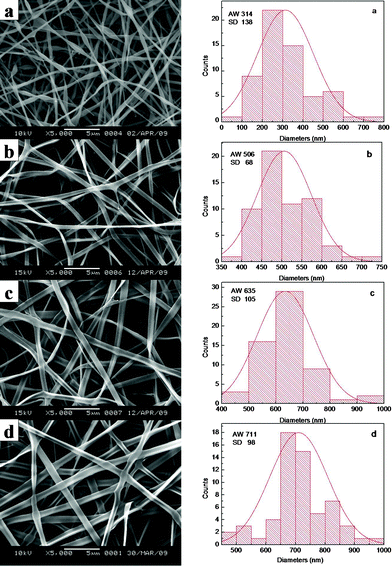 |
| Fig. 1 SEM micrographs and width distribution histograms of electrospun VC-2-p-loaded SF nanofibers (concentration of VC-2-p was 3 wt% based on the weight of SF) with different concentrations (a. 20 wt%, b. 25 wt%, c. 30 wt% and d. 35 wt%) of SF. | |
When SF was at the concentration of 25 wt%, the average width of VC-2-p-loaded SF nanofibers (n = 60) ranged from 362 ± 121 nm to 506 ± 68 nm with different amounts of VC-2-p. With the increasing concentration of VC-2-p, the morphology of fibers became relatively homogeneous and the average width of as-spun nanofibers increased gradually (Fig. 2).
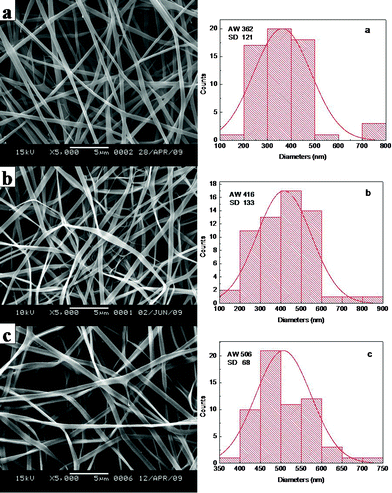 |
| Fig. 2 SEM micrographs and width distribution histograms of electrospun VC-2-p-loaded SF nanofibers (a. 25%SF, b. 1%VC-2-p-25%SF and c. 3%VC-2-p-25%SF). | |
Secondary structure of nanofibrous matrices
The potential skin benefits of VC-2-p-loaded SF nanofibrous matrices are determined by various factors, including the morphology and the structure of nanofibrous matrices. The previous studies have revealed that SF from Bombyx mori typically fostered two dimorphic structures, i.e., silk I and silk II.20,25 SF nanofibrous matrices in the silk I form are unstable, in which a random coil/helical conformation prevails. However, when the structure of SF nanofibrous matrices undergoes a conversion into the silk II type (which mainly adopts a β-sheet conformation), SF nanofibrous matrices become water-stable and this conversion depends on various conditions.20,21,26 Therefore, to discover the effects of VC-2-p and 75 v/v% ethanol vapor treatment on neat or VC-2-p-loaded SF nanofibrous matrices, the structure of VC-2-p-loaded SF nanofibrous matrices was determined using solid-state 13C-NMR analysis, in which the chemical shifts of Cα and Cβ in the alanine (Ala) residue are indicative of the SF conformation. It has been reported that the chemical shifts of Cα and Cβ in the Ala residue within the range 50.0–53.0 ppm and 14.5–17.5 ppm, respectively, indicated the silk I form and within the region of 48.0–50.0 ppm and 18.5–20.5 ppm, respectively, were for the silk II form.26
In this study, the chemical shifts of Ala Cβ in electrospun neat and VC-2-p-loaded SF nanofibrous matrices were 16.9, 16.4 and 16.4 ppm respectively (Fig. 3); in the case of Ala Cα, the chemical shifts were 50.5, 50.0 and 50.1 ppm for 25%SF, 1%VC-2-p-25%SF and 3%VC-2-p-25%SF, respectively (Fig. 3). After treatment of 75 v/v% ethanol vapor, the chemical shifts of Ala Cβ and Ala Cα in the both SF nanofibrous matrices (neat and VC-2-p-loaded SF nanofibrous matrices) changed into 18.5–20.5 ppm and 48.0–50.0 ppm, respectively (Fig. 4). These results suggested that electrospun neat and VC-2-p-loaded SF nanofibrous matrices typically took the silk I form, and thereby VC-2-p itself had no significant effect on the structure of SF nanofibrous matrices. With 75 v/v% ethanol vapor treatment, the structure of both SF nanofibrous matrices was successfully induced from the silk I form into silk II.
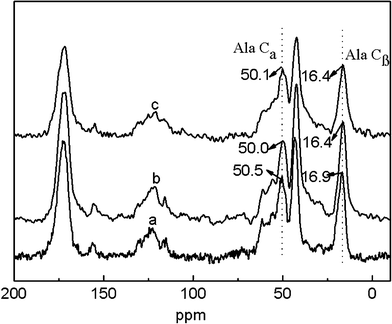 |
| Fig. 3
13C-NMR spectra of electrospun neat and VC-2-p-loaded SF nanofibrous matrices (a. 25%SF, b. 1%VC-2-p-25%SF and c. 3%VC-2-p-25%SF). | |
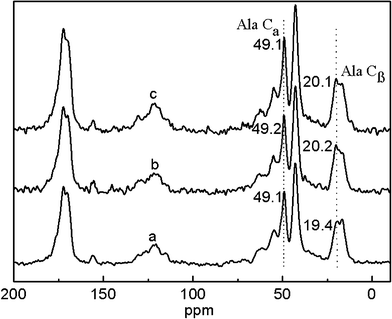 |
| Fig. 4
13C-NMR spectra of neat and VC-2-p-loaded SF nanofibrous matrices with 75 v/v% ethanol vapor treatment (a. 25%SF, b. 1%VC-2-p-25%SF and c. 3%VC-2-p-25%SF). | |
Release of VC-2-p from VC-2-p-loaded SF nanofibrous matrices in vitro
Although the formulation or preparation of VC-containing skin care products has been continuously optimized, many of them are still challenged by the poor disassociation of VC from vehicles.4,5,9
Here, the release profile of VC-2-p from SF nanofibrous matrices was determined by incubating nanofibrous matrices in ultrapure water (pH 7.0) with a continuous swing of 80 rpm at 32 ± 0.5 °C (ultrapure water was selected as the test medium based on the mobile phase of HPLC analysis). The cumulative amount of VC-2-p released was reported as the percentage of the actual amount of VC-2-p present in the sample (data not shown). It can be clearly seen that VC-2-p released from SF nanofibrous matrices exhibited a burst release behavior during the initial 20 min and then was gradually released from samples to reach a plateau (approximately 60% for 1%VC-2-p-25%SF and 70% for 3%VC-2-p-25%SF) with extending release time (Fig. 5). It is probably because the electrospinning process easily caused ions, namely VC-2-p, to locate close to the surface or on the surface of SF nanofibers and ribbon-like fibers facilitated this process, hence accelerating the diffusion of water-soluble VC-2-p into ultrapure water.27 Meanwhile the increased initial content of VC-2-p in nanofibers could enhance the driving force for diffusion, which aided VC-2-p to disassociate from 3%VC-2-p-25%SF more rapidly as compared to 1%VC-2-p-25%SF (Fig. 5).28 Therefore, the maximum skin benefits of VC-2-p-loaded SF nanofibrous matrices could be obtained by optimizing the amount of VC-2-p loaded into SF nanofibrous matrices, which will be further investigated in our future study.
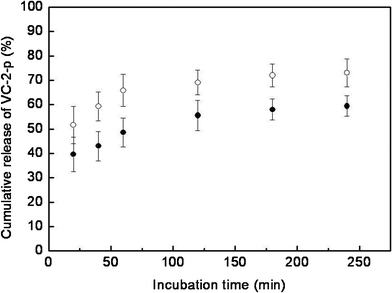 |
| Fig. 5 Release profiles of VC-2-p from SF nanofibrous matrices (● 1%VC-2-p-25%SF and ○ 3%VC-2-p-25%SF) in ultrapure water. | |
In addition, Fig. 2 and Fig. 6 showed that after 75 v/v% ethanol vapor treatment, SF nanofibers had a good water-resistance property (which is consistent with the result of 13C-NMR) while the incorporation and the release of VC-2-p had no significant effect on the fiber surface.
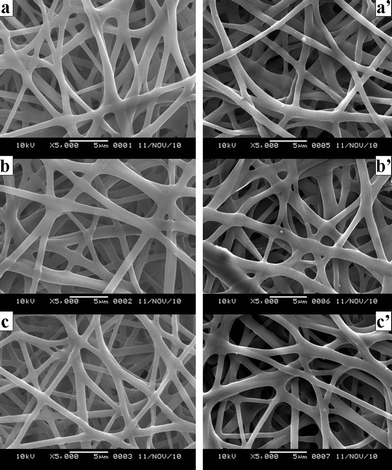 |
| Fig. 6 SEM micrographs of neat and VC-2-p-loaded SF nanofibers treated with 75 v/v% ethanol vapor (a. 25%SF, b. 1%VC-2-p-25%SF and c. 3%VC-2-p-25%SF); SEM micrographs of neat and VC-2-p-loaded SF nanofibers treated with 75 v/v% ethanol vapor after the release of VC-2-p (a'. 25%SF, b'. 1%VC-2-p-25%SF and c'. 3%VC-2-p-25%SF). | |
Phenotype and viability of L929 cells cultured on nanofibrous matrices
It is quite interesting to investigate the effect of VC-2-p-loaded SF nanofibrous matrices on the phenotype and viability of skin cells for possible use as skin care materials or skin scaffolds. In this work, such properties were studied by assessing cell adhesion, spreading and proliferation. L929 cells at the same amount as described before (i.e. 1.2 × 104 cells per matrix) were seeded onto neat or VC-2-p-loaded SF nanofibrous matrices (with cover slips as controls) and were incubated for different times (i.e., 1, 3, 5 and 7 days). Subsequently, cell viability was determined using an MTT assay. The results show an obvious enhancement on the cell population from day 1 to day 7 (Fig. 7). Against cover slips, both neat and VC-2-p-loaded SF nanofibrous matrices stimulated L929 cell adhesion and proliferation significantly on any day. In contrast, with the extended culture time, L929 cells on VC-2-p-loaded SF nanofibrous matrices proliferated faster than those on neat ones from day 5 to day 7, which is consistent with the stimulating effect of VC-2-p on skin fibroblast proliferation as reported in a previous study.22 The results above could also be caused by the capacity of coverslips and different nanofibrous matrices for decreasing accumulation of peroxides, which were formed in the process of cell metabolism and are harmful to the living cells.6,10 Therefore, our data reveal that the incorporation of VC-2-p had no adverse effect on the excellent cytocompatibility of SF nanofibrous matrices, and in turn tended to encourage L929 cells to proliferate.
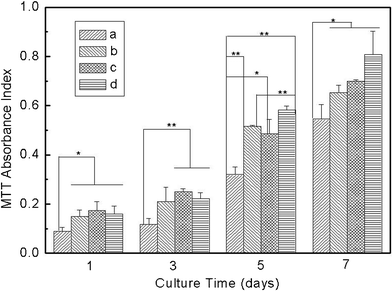 |
| Fig. 7 Viability assay of L929 cells cultured on neat and VC-2-p-loaded SF nanofibrous matrices (a. cover slips, b. 25%SF, c. 1%VC-2-p-25%SF and d. 3%VC-2-p-25%SF) for 1, 3, 5 and 7 days respectively (*P < 0.05, **P < 0.01). | |
On day 5 after seeding, a cell phenotype on different matrices was disclosed using SEM (Fig. 8). Cells on both cover slips and nanofibrous matrices typically adopted a fusiform or polygonal morphology and had spread over a large area (Fig. 8 a–d). Interestingly, cells on nanofibrous matrices started bridging each other to form cell sheets (Fig. 8 b–d). At a high magnification, it can be clearly seen that cells had started infiltrating through the pores and growing under layers of nanofibers (Fig. 8 b'–d'). Cells and nanofibrous matrices appeared to assemble cell–matrix composite structures and the compact interactions could be beneficial to the cell–cell and cell–matrix signal conduction and in turn further highlight the skin benefits of nanofibrous matrices in that the biomaterial matrix in essence is a translator transducing environmental signals to cells seeded on it.15
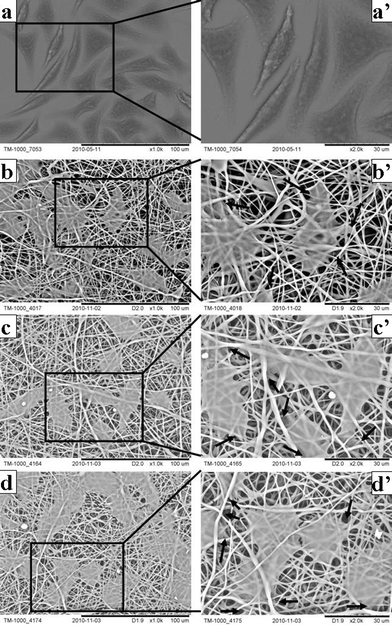 |
| Fig. 8 Cell phenotype on neat and VC-2-p-loaded SF nanofibrous matrices (a, a'. cover slips; b, b'. 25%SF; c, c'. 1%VC-2-p-25%SF; d, d'. 3%VC-2-p-25%SF) for 5 days at the magnification of 1000× and 2000× respectively. Arrows signify cells infiltrating through the pores and growing under layers of nanofibers. | |
The expression levels of some functional genes in the L929 cells cultured on the matrices
To assess skin benefits of the matrices, it is necessary to investigate their influence on the expression of important functional genes in the skin cells. In this work, we detected the mRNA levels of Col1a1, Gpx1 and Cat due to their contribution to the skin barrier (Type I collagen, the major collagen in skin tissue, dominates the structure, function and texture features of skin to a large extent; Gpx1 and Cat are two pivotal antioxidative enzymes in skin cells, protecting skin tissue from oxidative damage).29,30 As shown in Fig. 9, the cells cultured on SF nanofibrous matrices expressed Col1a1 mRNA with a significantly higher level than those on cover slips. Importantly, the VC-2-p-loaded SF nanofibrous matrices exhibited more powerful capacity on improving the expression level of Col1a1 compared with the neat ones and cells on 3%VC-2-p-25%SF had the highest expression level of Col1a1, which is also consistent with the previous study on VC-2-p promoting collagen accumulation.22 As to the other 2 functional genes detected, no significant difference was observed among the tested groups, which indicated that the as-prepared SF nanofibrous matrices have no adverse influence on the expression of these functional genes. Thus, our data demonstrate the remarkable property of SF nanofibrous matrices on skin benefit, and the incorporation of VC-2-p significantly reinforced such virtue of SF nanofibrous matrices.
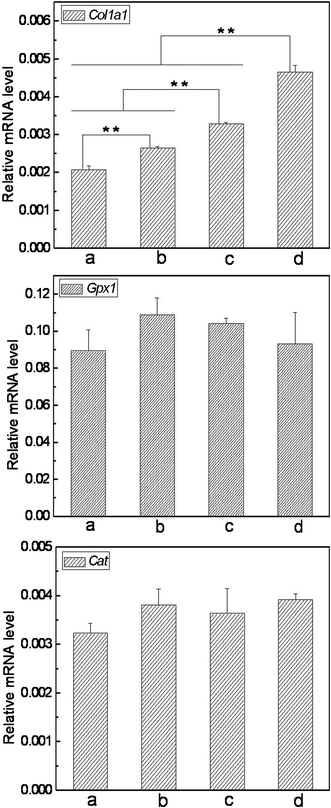 |
| Fig. 9 Expression of Col1a1, Gpx1 and Cat in L929 cells cultured on different nanofibrous matrices (a. cover slips, b. 25%SF, c. 1%VC-2-p-25%SF and d. 3%VC-2-p-25%SF) (**P < 0.01). | |
The assessment of viability and gene expression of L929 cells on nanofibrous matrices under exposure to oxidative stress (OS)
To further explore the skin benefits of nanofibrous matrices, we hypothesized that nanofibrous matrices could protect skin cells against OS through antioxidation. This was tested using an oxidative injury model where L929 cells cultured on nanofibrous matrices were challenged by t-BHP-induced OS (T-BHP, an OS-inducing agent, has been reported as a model compound to have insight into cell damage in relation to OS31,32). Consequently, the effect of t-BHP (at a concentration range 50∼200 μM) on cell viability was revealed using an MTT assay (Fig. 10). Our data show that when the concentration of t-BHP is 100 μM or more, the number of viable cells on cover slips reduced gradually against the control group without t-BHP. While for any of the three types of nanofibrous matrices, the significant difference between the tested group and the control group appeared only under severe OS resulted from 200 μM t-BHP. These findings indicated that cells on SF nanofibrous matrices can stand more severe oxidative conditions than on cover slips. Obviously, SF nanofibrous matrices contributed positively to the survival of the cells against t-BHP-induced oxidative insult and the incorporation of VC-2-p further highlighted this contribution, which is consistent with the result of cell proliferation under the studied conditions.
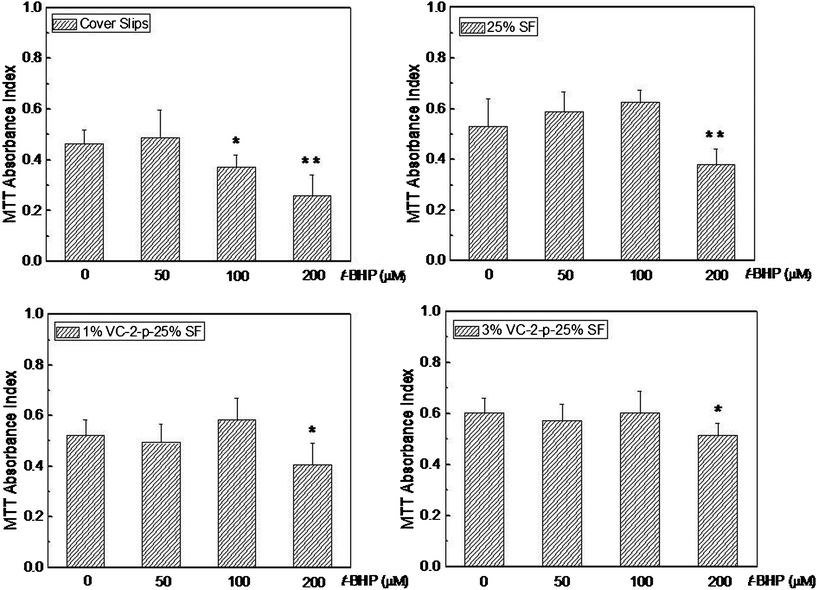 |
| Fig. 10 Viability assay of L929 cells cultured on cover slips, 25%SF, 1%VC-2-p-25%SF and 3%VC-2-p-25%SF after exposure to different concentrations of t-BHP (i.e., 50 μM, 100 μM and 200 μM). *P < 0.05 and **P < 0.01 versus the control group without t-BHP (0 μM t-BHP). | |
To disclose how the nanofibrous matrices protected cells from oxidative insult, it is meaningful to explore what happened on the expression of functional genes under severe OS. In this work, the mRNA levels of Col1a1, Gpx1 and Cat were detected by real time PCR following treatment with 200 μM of t-BHP for 24 h. Our data reveal that the expression level of these genes decreased significantly under severe OS compared with that of none t-BHP treatment (Fig. 11vs.Fig. 9). Meanwhile, the trend of Col1a1 expression among the detected groups was still similar to that prior to t-BHP treatment (Fig. 11vs.Fig. 9). For Gpx1 and Cat, although their expression exhibited no difference among the detected groups without t-BHP treatment (Fig. 9), the trend varied considerably under severe OS: both GPx1 and Cat mRNA levels in the cells on nanofibrous matrices were significantly higher than those on cover slips; importantly, the cells on VC-2-p-loaded SF nanofibrous matrices expressed remarkably higher levels of GPx1 and Cat mRNA than those on neat SF nanofibrous matrices (Fig. 11). Our result indicated that SF nanofibrous matrices can aid the skin cells to maintain a higher expression level of the functional genes such as Col1a1, Gpx1 and Cat under severe OS, and such capacity of SF nanofibrous matrices can be significantly reinforced with the incorporation of VC-2-p.
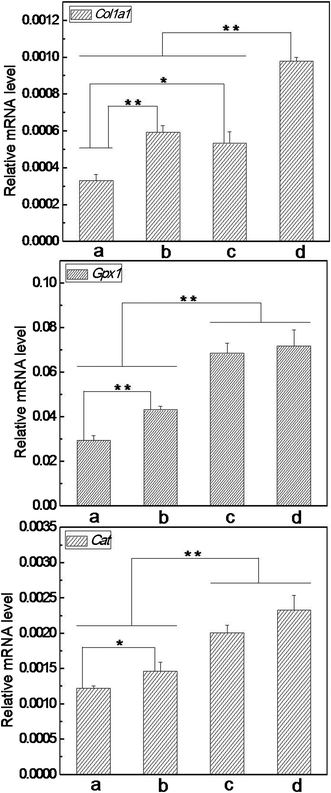 |
| Fig. 11 Expression of Col1a1, Gpx1 and Cat in L929 cells cultured on different nanofibrous matrices under exposure to 200 μM of t-BHP (a. cover slips, b. 25%SF, c. 1%VC-2-p-25%SF and d. 3%VC-2-p-25%SF) (*P < 0.05, **P < 0.01). | |
Accumulating evidence indicates that many skin disorders or diseases are mainly attributed to the overwhelmed generation of reactive oxygen species (ROS) like hydrogen peroxide, singlet oxygen and peroxides.2,3,33 An excess of ROS can oxidatively damage cell constituents including cell proteins, DNA, RNA and lipids by releasing OS,2,3,33–35 which is consistent with our results for cell viability and gene expression in the presence of severe OS. Responses of the organism to ROS or OS mainly rely on a variety of antioxidant enzymes (such as catalase and glutathione peroxidase) and physiological antioxidants (such as L-ascorbic acid and D-alpha-tocopherol) available for living cells.5,10,29,32–34,36 Here, we demonstrated that the VC-2-p loaded SF nanofibrous matrices can help the cells through antioxidation to maintain a higher expression level of Gpx1 and Cat, which means a better ability for cells to scavenge ROS or OS, and may in turn make the cells survive better from oxidative insult.
Conclusions
We successfully fabricated VC-2-p-loaded SF nanofibrous matrices using an eco-friendly electrospinning processing for the first time. After 75 v/v% ethanol vapor treatment, the structure of SF nanofibrous matrices with or without VC-2-p was converted from unstable silk I into water-stable silk II, as verified by SEM and 13C-NMR. Results from the HPLC assay confirmed that VC-2-p disassociated from SF nanofibrous matrices easily under the studied conditions. As-prepared SF nanofibrous matrices significantly promoted fibroblast proliferation and Col1a1 expression in the cells against cover slips. Importantly, the incorporation of VC-2-p further reinforced such capacity of SF nanofibrous matrices. Furthermore, under severe oxidative stress, VC-2-p-loaded SF nanofibrous matrices maintained the higher fibroblast viability and mRNA level of some key functional genes (Col1a1, Gpx1 and Cat) compared with neat SF nanofibrous matrices. Taken together, the present work confirmed that in combination with VC, the excellent skin benefits of SF nanofibrous matrices were further reinforced, which implies the promising application of VC-2-p-loaded SF nanofibrous matrices in personal skin care and skin regeneration, including serving as wound dressings and anti-aging materials.
Acknowledgements
This work was supported by the Shanghai-Unilever Research and Development Fund (08520750100), National Nature Science Foundation of China (31070871), the Natural Science Foundation of Shanghai (11ZR1400100), Fundamental Research Funds for the Central Universities, Open Foundation of State Key Laboratory for Modification of Chemical Fibers and Polymer Materials (LK1111, LK0804 and LZ0906) and the Program of Introducing Talents of Discipline to Universities (B07024).
References
- C. Baudouin, M. Charveron, R. Tarroux and Y. Gall, Environmental pollutants and skin cancer., Cell Biol. Toxicol., 2002, 18, 341–348 CrossRef CAS.
- Y. Saitoh, A. Miyanishi, H. Mizuno, S. Kato, H. Aoshima, K. Kokubo and N. Miwa, Super-highly hydroxylated fullerene derivative protects human keratinocytes from UV-induced cell injuries together with the decreases in intracellular ROS generation and DNA damages., J. Photochem. Photobiol., B, 2011, 102, 69–76 CrossRef CAS.
- P. Kovacic and R. Somanathan, Dermal Toxicity and Environmental Contamination: Electron Transfer, Reactive Oxygen Species, Oxidative Stress, Cell Signaling, and Protection by Antioxidants., Rev. Environ. Contam. Toxicol., 2010, 203, 119–138 CAS.
- Z. D. Draelos, Topical Agents Used in Association With Cosmetic Surgery., Semin. Cutaneous Med. Surg., 1999, 18, 112–118 CrossRef CAS.
- Z. D. Draelos, Novel topical therapies in cosmetic dermatology., Current Problems in Dermatology, 2000, 12, 235–239 CrossRef.
- S. R. Pinnell, Cutaneous photodamage, oxidative stress, and topical antioxidant protection., J. Am. Acad. Dermatol., 2003, 48, 1–19 CrossRef.
- B. V. Nusgens, Topically Applied Vitamin C Enhances the mRNA Level of Collagens I and III, Their Processing Enzymes and Tissue Inhibitor of Matrix Metalloproteinase 1 in the Human Dermis., J. Invest. Dermatol., 2001, 116, 853–859 CrossRef CAS.
- K. Kameyama, Inhibitory effect of magnesium L-ascorbyl-2-phosphate (VC-PMG) on melanogenesis in vitro and in vivo., J. Am. Acad. Dermatol., 1996, 34, 29–33 CrossRef CAS.
- S. Farahmand, H. Tajerzadeh and E. S. Farboud, Formulation and Evaluation of a Vitamin C Multiple Emulsion., Pharm. Dev. Technol., 2006, 11, 255–261 CrossRef CAS.
- R. E. Beyer, The role of ascorbate in antioxidant protection of biomembranes: interaction with vitamin E and coenzyme Q., J. Bioenerg. Biomembr., 1994, 26, 349–358 CrossRef CAS.
- T. Nakamura, S. R. Pinnell, D. Darr, I. Kurimoto, S. Itami, K. Yoshikawa and J. W. Streilein, Vitamin C abrogates the deleterious effects of UVB radiation on cutaneous immunity by a mechanism that does not depend on TNF-α., J. Invest. Dermatol., 1997, 109, 20–24 CAS.
- M Nishikimi, R Fukuyama, S Minoshima, N Shimizu and K. Yagi, Cloning and Chromosomal Mapping of the Human Nonfunctional Gene for L-Gulono-y-lactone Oxidase, the Enzyme for L-Ascorbic Acid Biosynthesis Missing in Man., J Biol Chem, 1994, 269, 13685–13688 CAS.
- X. M. Wu, C. J. Branford-White, D. G. Yu, N. P. Chatterton and L. M. Zhu, Preparation of core-shell PAN nanofibers encapsulated α-tocopherol acetate and ascorbic acid 2-phosphate for photoprotection., Colloids Surf., B, 2011, 82, 247–252 CrossRef CAS.
- C. Vepari and D. L. Kaplan, Silk as a biomaterial., Prog. Polym. Sci., 2007, 32, 991–1007 CrossRef CAS.
- C. Sobajo, F. Behzad, X. F. Yuan and A. Bayat, Silk: A Potential Medium for Tissue Engineering., Eplasty, 2008, 8, 438–446 Search PubMed.
- A. Sugihara, Promotive effects of a silk film on epidermal recovery from full-thickness skin wounds., Proc. Soc. Exp. Biol. Med., 2000, 225, 58–64 CrossRef CAS.
- D. H. Roh, Wound healing effect of silk fibroin/alginate-blended sponge in full thickness skin defect of rat., J. Mater. Sci.: Mater. Med., 2006, 17, 547–552 CrossRef CAS.
- G. Ricci, A. Patrizi, B. Bendandi, G. Menna, E. Varotti and M. Masi, Clinical effectiveness of a silk fabric in the treatment of atopic dermatitis., Br. J. Dermatol., 2004, 150, 127–131 CrossRef CAS.
- A. V. Daithankar, M. N. Padamwar, S. S. Pisal, A. R. Paradkar and K. R. Mahadik, Moisturizing efficiency of silk protein hydrolysate: Silk fibroin., Indian J Biotechnol, 2005, 4, 115–121 CAS.
- B. M. Min, L. Jeong, Y. S. Nam, J. M. Kim, J. Y. Kim and W. H. Park, Formation of silk fibroin matrices with different texture and its cellular response to normal human keratinocytes., Int. J. Biol. Macromol., 2004, 34, 223–230 CrossRef.
- L. P. Fan, H. S. Wang, K. H. Zhang, C. L. He, Z. X. Cai and X. M. Mo, Regenerated silk fibroin nanofibrous matrices treated with 75% ethanol vapor for tissue engineering applications., J. Biomater. Sci., Polym. Ed., 2012, 23, 497–508 CrossRef CAS.
- R. I. Hata and H. Senoo, L-Ascorbic Acid 2-Phosphate Stimulates Collagen Accumulation, Cell Proliferation, and Formation of a Three-Dimensional Tissuelike Substance by Skin Fibroblasts., J. Cell. Physiol., 1989, 138, 8–16 CrossRef CAS.
- H. Shibayama, M. Hisama, S. Matsuda and M. Ohtsuki, Permeation and Metabolism of a Novel Ascorbic Acid Derivative, Disodium Isostearyl 2-O-L-Ascorbyl Phosphate, in Human Living Skin Equivalent Models., Skin Pharmacol. Physiol., 2008, 21, 235–243 CrossRef CAS.
- K. J. Livak and T. D. Schmittgen, Analysis of relative gene expression data using real-time quantitative PCR and the 2(-Delta Delta C(T)) method. Methods, 2001, 25, 402–408 CAS.
- T. Asakura, J. M. Yao, T. Yamane, K. Umemura and A. S. Ulrich, Heterogeneous Structure of Silk Fibers from Bombyx mori Resolved by 13C Solid-State NMR Spectroscopy., J. Am. Chem. Soc., 2002, 124, 8794–8795 CrossRef CAS.
- P. Zhou, G. Y. Li, Z. Z. Shao, X. Y. Pan and T. Y. Yu, Structure of Bombyx mori Silk Fibroin Based on the DFT Chemical Shift Calculation., J. Phys. Chem. B, 2001, 105, 12469–12476 CrossRef CAS.
- Y. Su, X. Q. Li, L. J. Tan, C. Huang and X. M. Mo, Poly(L-lactide-co-ε-caprolactone) electrospun nanofibers for encapsulating and sustained releasing proteins., Polymer, 2009, 50, 4212–4219 CrossRef CAS.
- P. Taepaiboon, U. Rungsardthong and P. Supaphol, Vitamin-loaded electrospun cellulose acetate nanofiber mats as transdermal and dermal therapeutic agents of vitamin A acid and vitamin E., Eur. J. Pharm. Biopharm., 2007, 67, 387–397 CrossRef CAS.
- H. Masaki, Y. Okano and H. Sakurai, Differential role of catalase and glutathione peroxidase in cultured human fibroblasts under exposure of H2O2 or ultraviolet B light., Arch. Dermatol. Res., 1998, 290, 113–118 CrossRef CAS.
- K. Gelse, E. Pöschl and T. Aigner, Collagens—structure, function, and biosynthesis., Adv. Drug Delivery Rev., 2003, 55, 1531–1546 CrossRef CAS.
- S. J. Bae, 5-Hydroxytrytophan Inhibits tert-Butylhydroperoxide (t-BHP)-Induced Oxidative Damage via the Suppression of Reactive Species (RS) and Nuclear Factor-KB (NF-KB) Activation on Human Fibroblast., J. Agric. Food Chem., 2010, 58, 6387–6394 CrossRef CAS.
- W. S. Kim, Evidence supporting antioxidant action of adipose-derived stem cells: Protection of human dermal fibroblasts from oxidative stress., J. Dermatol. Sci., 2008, 49, 133–142 CrossRef CAS.
- M. H. Shin, H2O2 Accumulation by Catalase Reduction Changes MAP Kinase Signaling in Aged Human Skin In Vivo., J Invest Dermatol, 2005, 125, 221–229 CAS.
- L. Declercq, I. Sente, L. Hellemans, H. Corstjens and D. Maes, Use of the synthetic superoxide dismutase/catalase mimetic EUK-134 to compensate for seasonal antioxidant deficiency by reducing pre-existing lipid peroxides at the human skin surface., Int. J. Cosmet. Sci., 2004, 26, 255–263 CrossRef CAS.
- W. Martinet, G. R. Y. De Meyer, A. G. Herman and M. M. Kockx, Reactive oxygen species induce RNA damage in human atherosclerosis., Eur. J. Clin. Invest., 2004, 34, 323–327 CrossRef CAS.
- J. Fuchs and H. Kern, Modulation of UV-light-induced skin inflammation by D-alpha-tocopherol and L-ascorbic acid: a clinical study using solar simulated radiation., Free Radical Biol. Med., 1998, 25, 1006–1012 CrossRef CAS.
|
This journal is © The Royal Society of Chemistry 2012 |
Click here to see how this site uses Cookies. View our privacy policy here.