DOI:
10.1039/C2RA20974H
(Communication)
RSC Adv., 2012,
2, 6170-6173
A synergetic whole-cell biocatalyst for biodiesel production
Received
17th May 2012
, Accepted 21st May 2012
First published on 25th June 2012
Abstract
As an alternative, biodegradable and nonpolluting transportation fuel, biodiesel has recently attracted considerable attention. Comparing with conventional chemical catalysis and supercritical methanol, enzymatic transesterification for biodiesel is one of the most promising strategies due to its gentle reaction conditions, environmentally friendly nature, oil feedstock permission and easy product separation. However, the two major obstacles of high cost and easy inactivation of lipases remain to retard their wide commercialization. To overcome these bottlenecks of enzymatic catalysis biodiesel production, we herein report a novel economical biocatalyst by co-displaying two synergistic lipases, Candida antarctica lipase B and Thermomyces lanuginosus lipase, on the surface of Phichia pastoris cell to markedly cut down the cost of biodiesel production. Results showed that the co-displayed whole-cells were promising biocatalysts in biodiesel production with high biodiesel conversion (ca. 95.4%) and good operational stability, which suggests biodiesel catalyzed by the co-displayed whole-cell catalysts should be a promising alternative technology for biodiesel future development.
1. Introduction
With the depletion of fossil fuels and deterioration of environmental pollution, renewable and clean biofuels are very imperative for the sustainable development of mankind.1 As one of the most ideal renewable and clean energies, biodiesel has been recently attracted more and more attention.2 Several conventional methods for biodiesel production are available in the literature, such as alkaline/acid catalysts, supercritical fluid, and biocatalysts. The alkaline/acid- catalyzed method, though widely employed, has so many drawbacks that purification of the product is complex and laborious.3 The supercritical fluid method, using supercritical alcohol and other solvents,4,5 is an energy consuming procedure with high temperature and pressure. In contrast, the lipase-catalyzed method is one of the most promising strategies due to its gentle reaction conditions, environmental friendly nature, oil feedstock permission and easy product separation.6 Biodiesel production catalyzed by lipases has been extensively studied from all kinds of oil feedstocks.7–9 In our previous work, we developed a novel alternative procedure of biodiesel production using the combined lipases of 1.96% Novozym435 and 2.04% Lipozyme TLIM as a biocatalyst, which could obtain a biodiesel yield of more than 97% after 8 h reaction, and the activity of the combined lipases remained at 95% after 300 cycles (ca. 2400 h).10 However, the cost of the lipase associated with its laborious preparation processes including purification from the culture broth, finding a suitable supporting carrier and immobilization with an effective method, as well as biodiesel’s poor market competitiveness to fossil diesel, seem to be the major obstacles to the industrialization of lipase-catalyzed transesterification for biodiesel production.
Compared with traditional immobilization, surface display of enzymes as whole cell catalysts may be a better solution because it is a kind of self-immobilization and needs no purification or complex immobilization procedure. So far, many functional enzymes have been genetically immobilized on the cell surface of Saccharomyces cerevisiae and Pichia pastroris due to their safety, simplicity of genetic manipulation and rigidity of the cell-wall structure.11 Therefore, to avoid the complex and laborious preparation of immobilization lipase, a novel whole-cell biocatalyst co-displaying two synergetic lipases, C. antarctica lipase B (CLAB, commercial name is Novozyme 435) and T. lanuginosus lipase (TLL, commercial name is Lipozyme TLIM), on the surface of P. pastoris cell was developed for the first time for biodiesel production. The enzymatic properties were characterized, and the catalyzed transesterification reaction conditions were further optimized with Plackett–Burman (PB) design and response surface methodology (RSM). Furthermore, its catalysis effect was compared with the commercial immobilized lipases Novozyme 435, Lipozyme TLIM and their combined form under their optimum conditions.10 In addition, to evaluate its potentiality for large-scale application, the operational stability of the co-displayed lipases was also explored.
2. Experimental
The procedure for developing the whole-cell biocatalyst co-displaying on the surface of P. pastoris cells was modified from our former study.12 Firstly, we evaluated the displaying results of several anchor proteins (Cwp2p, Sed1p, α-lectin and Flo1p) fused with green fluorescent protein EGFP or red fluorescent protein RFP in pPIC9k and pPICZαA, respectively. Then, a proper system was chosen to make a construction of single displaying system of CALB or TLL. Consequently, the displaying vector pPICZαA-TLL was then electro-transformed into GS115/pPIC9k-CALB. The recombinants were screened and a recombinant containing two lipases with the highest activity of 623 U/g-dry cells was obtained, which was 7.3 and 2.4 times that of the single displayed CALB and TLL (Fig. 1).
![Screening of co-displayed recombinant yeast strains. [The recombinants were primary screened in a plate with Rhodamine B as indicator, and the size of halos around the strains was observed. Then, those exhibiting large-size halos were selected for flask fermentation, and checked their hydrolysis activity. There are several strains showing highest activities.]](/image/article/2012/RA/c2ra20974h/c2ra20974h-f1.gif) |
| Fig. 1 Screening of co-displayed recombinant yeast strains. [The recombinants were primary screened in a plate with Rhodamine B as indicator, and the size of halos around the strains was observed. Then, those exhibiting large-size halos were selected for flask fermentation, and checked their hydrolysis activity. There are several strains showing highest activities.] | |
Using the co-displayed whole-cell catalysts, the methanolysis of soybean oil was conducted in a screw-capped bottle with incubation at 40 °C in a reciprocal shaker with the speed rate of 200 rpm. The composition of the reaction mixture was as follows: soybean oil 2.19 g, methanol 0.4 mL (methanol/oil molar ratio 4
:
1), and tert-butanol 1.11 mL. These components were mixed with 5 wt% (based on oil weight, w/w) of the dried whole-cell biocatalyst in a 50 mL screw-capped bottle. Samples were periodically (interval 12 h) removed from the reaction mixtures and analyzed by gas chromatography, which was detailed in our previous work.10 Based on this, the transesterification reaction parameters were further optimized via PB design and RSM,13 and the catalytic effect was compared respectively with the commercial immobilized lipases Novozyme 435, Lipozyme TLIM and their combined form under their optimal transesterification conditions so as to assess the superiority of the co-displayed lipases. Furthermore, to evaluate the potentiality for large scale application of the co-displaying lipases, the operational stability was also explored. The co-displayed lipases were centrifuged from the reaction system and regenerated by washing with organic solvent tert-butanol after each reaction cycle.
3. Results and discussion
3.1. Whole-cell biocatalysts of CALB and TLL co-displayed in P. pastoris
First, a display vector pPICZαA-TLL was constructed on the base of Sed1p and then transformed into GS115. TLL was successfully displayed on the surface of GS115, and exhibited a hydrolysis activity of 257.8 U/g dry cells towards olive oil. Then, the displaying vector pPICZαA-TLL was transformed into GS115/pPIC9k-CALB. The recombinants were screened and a recombinant containing two lipases with the highest activity of 623 U/g dry cells (Fig. 1) was obtained, which was 7.3 and 2.4 times as the single displayed CALB and TLL. The treated cells containing two lipases under the fluorescence microscope emitted bright red under 495 nm and green fluorescence under 545 nm at the same time, which indicated CALB and TLL were both successfully co-displayed on the yeast cell (Fig. 2). Flow cytometer analysis showed that the displaying rates of CALB and TLL were 94.9% and 74.5%, respectively (Fig. 3). The co-displayed CALB and TLL showed excellent thermostability and good organic solvents tolerance. The whole-cell biocatalyst had an optimal temperature and pH at 30 °C and 8.5, respectively, and it can keep more than 80% of original activity within a wide temperature (20–60 °C) and pH (6.0–9.0) range. The biocatalyst was weakly inactivated by K2+, Ca2+, Mg2+, EDTA, DMSO, TritonX-100, SDS, while Cu2+ showed strong inhibition effect on the whole-cell biocatalyst.
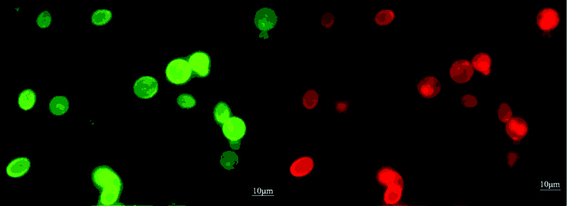 |
| Fig. 2 Fluorescence microscope image of co-displayed recombinants. The figure shows the same view under the fluorescence microscope. Right: CALB lipase cells under 495 nm exciting light. Left: TLIM lipase cells under 545 nm exciting light. | |
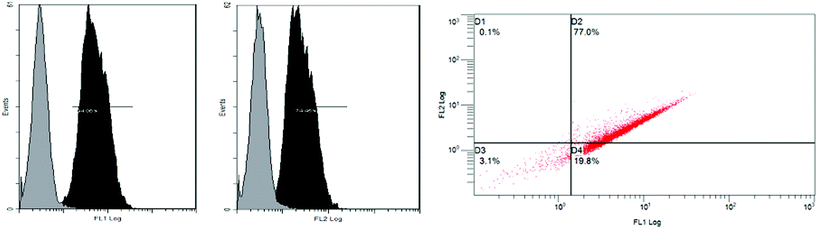 |
| Fig. 3 Flow cytometer analysis of co-displayed recombinants. Compared with controls cells GS115 which was indicated by grey peaks, FL1 (green fluorescence) and FL2 (red fluorescence) channels could be tested with strong positive signals and showing significant floating, the floating rates were 94.86% for GS115/pPICZαA-TLS and 74.45% for GS115/pPIC9k-CAS, which were indicated by black peaks. | |
3.2. Confirmation of the synergy of the whole-cell biocatalyst
As a whole-cell catalyst, the fermentation cells co-displayed of CALB and TLL were used to prepare biodiesel from soybean oil and methanol. The transesterification reaction conditions were preliminarily set at: methanol/oil ratio 1
:
4, soy oil 2.19 g, tert-butanol 1.111 mL, methanol 0.404 mL, lipase loading 80 U, reaction temperature 40 °C, rotating speed 200 r min−1, in a 50 mL stoppered Erlenmeyer flask.10 After 96 h reaction, the biodiesel yield was 72.62%, which is 11.04% and 12.62% more than the single displayed CALB and TLL, respectively (Fig. 4). The reason is probably that the co-displayed lipases of CALB and TLL present positive synergistic effect resulting in high conversion rate of biodiesel. As known, the enzymatic transesterification process for biodiesel production involves three steps, i.e., from triglyceride (TG) to diglyceride (DG), from diglyceride to monoglyceride (MG) and from monoglyceride to glycerol (GL).14 During temporal reaction dynamics, for transesterification process of single displayed CALB, the reaction step from MG to GL was faster than the steps from TG to DG and DG to MG and the slowest step from DG to MG was the rate-limiting step. While for the single displayed TLL, the rate-limiting step was the step from TG to DG. Therefore, co-displayed lipases of CALB and TLL could eliminate the rate-limiting step resulting in biodiesel conversion enhancement. In conclusion, co-displayed CALB and TLL exhibits a significantly synergetic effect and thus is quite effective to reduce the cost of lipase because it provides biocatalyst with multi-functionality without complex preparation procedures.
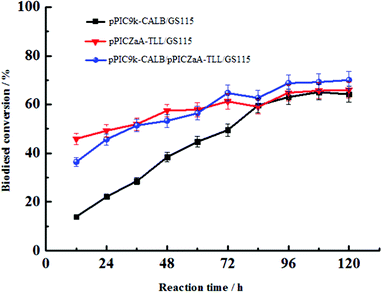 |
| Fig. 4 Time course of biodiesel production by whole-cell catalyst in tert-butanol. Conditions: methanol/oil ratio 1 : 4, soy oil 2.19 g, tert-butanol 1.111 mL, methanol 0.404 mL, lipase loading 80 U, reaction temperature 40 °C, rotating speed 200 r min−1, reaction 96 h, in a 50 mL stoppered Erlenmeyer flask. The ME yields for single displayed CALB, TLL and co-displayed CALB-TLL were 61.58%, 60.00%, and72.62%, respectively. | |
3.3. Optimization of biodiesel preparation using the whole-cell catalyst
The optimal conditions of ME yields catalyzed with the co-displayed lipases were obtained through PB design and RSM, which were an methanol/oil ratio 1
:
4.2, tert-butanol quantity (v/v) 0.56, methanol quantity (mol/mol) 5.36, enzyme loading (g g−1) 0.12, reaction temperature 40.5 °C, reaction time 12.6 h. Under the conditions, the predicted value of ME yield was 96.5% and the actual experimental value was 95.4 ± 0.6%, which coincided with the predicted one. However, the ME yields catalyzed by the same quantity of enzymatic activity of Lipozyme TLIM, Novozym435 and the combination of two were 51.2%, 87.6%, 97.3%, respectively. Therefore, the results indicate that the co-displayed synergetic lipases exhibit similar function as the combination of the two immobilized lipases, but they omit the complex and laborious procedure of preparation for the immobilized lipases, which suggests a promising prospect in application.
3.4. Study on operational stability and reusability
The operational stability of the co-displayed lipases was further explored so as to evaluate its potential for large-scale application. Fig. 5 shows that there is less than 16% loss in activity after being repeatedly used for 15 cycles, which indicates that the derivative lipase has fairly good operational stability. The probable reasons are due to the co-displaying enhances lipase's stability, and in the transesterification reaction system, tert-butanol can increase the solubility of methanol and glycerol in oil, resulting in a great decrease of their negative effects on co-displayed lipase. As can been seen, the study provided a potential alternative technology for biodiesel production in the future.
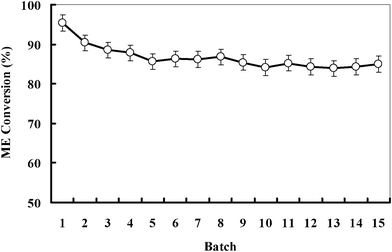 |
| Fig. 5 Operational stability of co-displayed lipase in the tert-butanol system. Conditions: oil, 2 g, methanol/oil ratio 1 : 4.2, tert-butanol quantity (v/v) 0.56, methanol quantity (mol/mol) 5.36, enzyme loading (g g−1) 0.12, reaction temperature 40 °C, stirring rate 200 rpm, reaction time 12 h. | |
4. Conclusions
The objective of this communication is to explore a high effective whole-cell biocatalyst through coexpressing CLAB and TLL in P. pastoris cell for biodiesel production. From the industrialization view of point, biodiesel by synergetic whole-cell bio-catalyzed transesterification should be a promising alternative technology for enhancing biodiesel benefits. In conclusions, it can be applied to further develop economical-viable enzymatic production of biodiesel in industrial scale.
Acknowledgements
We acknowledge the financial support by the National Natural Science Foundation of P. R. China (NSFC) (Nos. 31070089 and 31170078), the National High Technology Research and Development Program of P. R. China (863 Program) (No. 2011AA02A204).
References
- J. C. Serrano-Ruiz, E. V. Ramos-Fernández and A. Sepúlveda-Escribano, Energy Environ. Sci., 2012, 5, 5638–5652 CAS.
- R. Luque, J. C. Lovett, B. Datta, J. Clancy, J. M. Campelo and A. A. Romero, Energy Environ. Sci., 2010, 3, 1706–1721 CAS.
- Y. Liu, L. Wang and Y. Yan, Fuel Process. Technol., 2009, 9, 857–862 CrossRef.
- K. T. Tan, M. M. Gui, K. T. Lee and A. R. Mohamed, J. Supercrit. Fluids, 2010, 53, 82–87 CrossRef CAS.
- C. H. Chen, W. H. Chen, C. M. J. Chang, S. M. Lai and C. H. Tu, J. Supercrit. Fluids, 2010, 52, 228–234 CrossRef CAS.
- Y. Liu, T. Liu, X. Wang, L. Xu and Y. Yan, Energy Fuels, 2011, 25, 1206–1212 CrossRef CAS.
- X. Li, X. Y. He, Z. L. Li, Y. D. Wang, C. Y. Wang, H. Shi and F. Wang, Fuel, 2012, 92, 89–93 CrossRef CAS.
- Y. Wang and X. Cao, Bioresour. Technol., 2011, 102, 10173–10179 CrossRef CAS.
- I. C. Veras, F. A. L. Silva, A. D. Ferrao-Gonzales and V. H. Moreau, Bioresour. Technol., 2011, 102, 9653–9658 CrossRef CAS.
- Y. Huang, H. Zheng and Y. Yan, Appl. Biochem. Biotechnol., 2010, 160, 504–515 CrossRef CAS.
- P. N. Lipke and R. Ovalle, J. Bacteriol., 1998, 180, 3735–3740 CAS.
- W. Liu, B. Jia, H. Zhao, L. Xu and Y. Yan, J. Agric. Food Chem., 2010, 58(19), 10426–10430 CrossRef CAS.
- S. F. A. Halim and A. H. Kamaruddin, Process Biochem., 2008, 43, 1436–1439 CrossRef CAS.
- Q. Li, J. Zheng and Y. Yan, Fuel Process. Technol., 2010, 91(10), 1229–1234 CrossRef CAS.
|
This journal is © The Royal Society of Chemistry 2012 |
Click here to see how this site uses Cookies. View our privacy policy here.