DOI:
10.1039/C2RA21632A
(Paper)
RSC Adv., 2012,
2, 8789-8792
Sustainable H2O/ethyl lactate system for ligand-free Suzuki–Miyaura reaction†
Received
1st August 2012
, Accepted 1st August 2012
First published on 3rd August 2012
Abstract
A sustainable catalyst system consisted of H2O/ethyl lactate (EL), Pd(OAc)2 and K2CO3 has been developed as a generally applicable protocol for Suzuki–Miyaura reactions using various aryl bromides and iodides to incorporate arylboronic acids under ligand-free conditions. This protocol is advantageous owing to the employment of water and non-toxic, biomass available ethyl lactate as green media. In addition, as a co-solvent with water, ethyl lactate displayed evident superiority over conventional polar organic solvents such as DMF, DMSO and dioxane etc., which implied the additional potential function of EL as a ligand in these reactions.
Introduction
The discovery of the Suzuki–Miyaura reaction has brought revolutionary progress to synthetic organic chemistry by providing a universally applicable method of constructing sp2 C–C bonds via the coupling of aryl/vinyl halides and boranes.1 The past several decades have witnessed spectacular advances on the research of the Suzuki–Miyaura reaction, and the application of this classical transformation has nowadays permeated to the synthesis of natural products, agrochemicals, clinical medicines, intermediates of the chemical industry as well as functionalized materials.2 Among the current extensive research interests into the field of the Suzuki–Miyaura reaction, developing green and sustainable catalyst protocols represents one of the most widely concerned directions. In order to attain ideal results with eco-friendly processes, numerous efforts have been made to establishing green methodologies for the Suzuki–Miyaura reaction.
Currently, routes of designing eco-friendly protocols for the Suzuki–Miyaura reaction include alternating traditional phosphine ligands with environmentally benign ligands or ligand-free catalysis,3 reactions under mild conditions,4 reactions at low catalyst loading, transition metal-free conditions or employing reusable supported catalysts.5 However, most efforts on green Suzuki–Miyaura methodology have focused on the search of green and sustainable solvent mediated catalyst systems. A lot of different green media have been employed for developing the Suzuki–Miyaura reaction in eco-friendly conditions. Typical examples in this area include water-poly(ethylene glycol) (PEG),6 water–acetone,7 water–ionic liquid,8 water–MeCN,9 water–DMF,3d water–alcohol (EtOH, i-PrOH, BuOH) etc.,10 to name but a few. On the other hand, using only water as solvent for the Suzuki–Miyaura reactions had also been achieved with elegant results, the limit for these reactions was the need for prior decorated palladium catalysts with functional organic auxiliaries, harsh heating, special ligands or other external assistance in most cases.11 For those reactions performed under conventional heating with simple palladium catalysts such as PdCl2, Pd(OAc)2etc., it seems that the presence of organic solvent is still currently inevitable to guarantee satisfactory results.12 In this regard, the design of a catalyst system employing authentically nontoxic and biodegradable organic medium is a promising direction for establishing greener Suzuki–Miyaura reactions.
In recent years, nontoxic biomass available solvents have been regarded as highly potential sustainable media for organic synthesis.13 Solvents of this type such as glycerol,14 glycerol ethers15etc. have already shown broad applicability as media in organic synthesis. Ethyl lactate is another biomass-derivable solvent that possesses unique advantages of low production cost, nontoxicity (used as additive in food industry), low environmental impact (completely biodegradable), good compatibility with water, high boiling point (154 °C) and excellent solubility to organic compounds.16 Therefore, ethyl lactate is theoretically an ideal solvent as medium for sustainable organic synthesis. However, the research on EL mediated organic synthesis is presently in its infancy,17 to our best knowledge, the Suzuki–Miyaura reaction using EL or similar biomass derived solvents is not yet available. Herein, we report the first water–EL mixed green solvent mediated Suzuki–Miyaura reaction using a simple commercially available palladium catalyst under ligand free conditions.
Results and discussion
We started to explore the method on the model reaction of iodobenzene 1a (0.5 mol) and phenylboronic acid 2a (0.75 mol) with palladium catalysis. In the presence of K2CO3, 1 mol% Pd(OAc), reactions performed at 60 °C in 3 mL of EL solvent, pure water and EL
:
water (2
:
1) and (1
:
1) mixture suggested that 1
:
1 mixed EL and water was better than other media systems (entries 1–4, Table 1), further modification of the solvent proved that 2 mL of 1
:
1 mixed EL
:
water gave even better result (entry 5, Table 1). With this established mixed solvent system, extensive investigation was conducted to evaluate the effect of temperature (entry 6, Table 1), base species (entries 7–9, Table 1) and catalyst loading (entries 10–11, Table 1). Through comparing the results from these experiments, optimal conditions turned out to be 1 mol% Pd(OAc)2, 2 eq of K2CO3 in 2 mL mixed EL
:
water (1
:
1) and 60 °C (entry 5, Table 1). Further experiments performed with PdCl2 as catalyst or with DMF–water, DMSO–water, ethyl acetate–water, dioxane–water as mixed media all gave inferior yield of the product 3a (entries 12–16, Table 1).
Table 1 Optimization of reaction conditionsa
Entry |
Catalyst (mol %) |
Base |
Solvent (mL) |
Yield (%)b |
Unless specified, the general conditions: 1a (0.5 mmol), 2a (0.75 mmol) and base (2 eq) at 60 °C for 2 h. EL = ethyl lactate, EA = ethyl acetate.
Isolated yield based on 1a.
This reaction was performed at 45 °C.
|
1 |
Pd(OAc)2 (1) |
K2CO3 |
EL : H2O (2 : 1) |
26 |
2 |
Pd(OAc)2 (1) |
K2CO3 |
EL (3) |
62 |
3 |
Pd(OAc)2 (1) |
K2CO3 |
H2O (3) |
60 |
4 |
Pd(OAc)2 (1) |
K2CO3 |
EL : H2O (1.5 : 1.5) |
56 |
5 |
Pd(OAc)2 (1) |
K2CO3 |
EL : H2O (1 : 1) |
93 |
6c |
Pd(OAc)2 (1) |
K2CO3 |
EL : H2O (1 : 1) |
47 |
7 |
Pd(OAc)2 (1) |
Na2CO3 |
EL : H2O (1 : 1) |
62 |
8 |
Pd(OAc)2 (1) |
Et3N |
EL : H2O (1 : 1) |
53 |
9 |
Pd(OAc)2 (1) |
NaHCO3 |
EL : H2O (1 : 1) |
44 |
10 |
Pd(OAc)2 (0.5) |
K2CO3 |
EL : H2O (1 : 1) |
52 |
11 |
Pd(OAc)2 (0.25) |
K2CO3 |
EL : H2O (1 : 1) |
44 |
12 |
PdCl2 (1) |
K2CO3 |
EL : H2O (1 : 1) |
23 |
13 |
Pd(OAc)2 (1) |
K2CO3 |
DMF : H2O (1 : 1) |
70 |
14 |
Pd(OAc)2 (1) |
K2CO3 |
DMSO : H2O (1 : 1) |
57 |
15 |
Pd(OAc)2 (1) |
K2CO3 |
Dioxane : H2O (1 : 1) |
58 |
16 |
Pd(OAc)2 (1) |
K2CO3 |
EA : H2O (1 : 1) |
15 |
Based on the optimized results, we first examined the applicable scope by using different iodobenzenes and arylboronic acid derivatives. The results from this section were shown in Table 2. It was found that most aryl iodides reacted with phenylboronic acids to give corresponding biaryls with excellent yields since aryl iodides are known as active electrophiles in various cross coupling reactions. The heteroaryl substrate thiophen-2-boronic acid provided relatively lower yield of the corresponding product when reacted with 1a (entry 11, Table 2). Based on these typical examples, it was undoubted that the palladium-catalyzed green protocol consisted of EL–water was well suited for the Suzuki–Miyaura cross coupling of aryl iodide substrates with boronic acids. With these acquired results, we believed that this method should be also applicable for the reactions of aryl bromides. Therefore, we turned to examine the Suzuki–Miyaura reactions using aryl bromides as these reactions represented more practical application of a catalytic system for the Suzuki–Miyaura reaction. The reaction results acquired from a broad array of aryl/heteroaryl bromides and aryl boronic acids were outlined in Table 3. As expected, the reactions of aryl bromides have also shown excellent tolerance to this protocol albeit the reaction temperature was enhanced because of lower reactivity of these bromide substrates. Generally, aryl bromides with electron withdrawing as well donating groups in the ortho-, meta- and para-positions, and heteroaryl bromides were able to smoothly incorporate a class of different aryl boronic acids to give corresponding biaryls 3 in moderate to excellent yields. Based on the results from these present entries, the properties of the functional groups in both reactants didn't show any regular effects on the reaction efficiency. On the other hand, an attempt on employing functional aryl chlorides such as p-tolyl chloride as a reaction partner showed only trace transformation under present conditions.
Entry |
R1 |
Ar |
Product |
Yield (%)b |
General reaction conditions: 1 (0.5 mmol), 2 (0.75 mmol) mixed in 1 : 1 EL : H2O, stirred at 60 °C for 2 h in the presence of 0.005 mmol Pd(OAc)2 and 1 mmol K2CO3.
Isolated yield based on 1.
|
1 |
H |
Ph |
3a
|
93 |
2 |
4-MeO |
Ph |
3b
|
99 |
3 |
3-NO2 |
Ph |
3c
|
91 |
4 |
2-MeO |
Ph |
3d
|
81 |
5 |
2-OH |
Ph |
3e
|
80 |
6 |
H |
4-FC6H4 |
3f
|
98 |
7 |
H |
4-ClC6H4 |
3g
|
97 |
8 |
H |
4-MeOC6H4 |
3b
|
82 |
9 |
H |
4-MeC6H4 |
3h
|
70 |
10 |
H |
2-MeC6H4 |
3i
|
97 |
11 |
H |
Thiophene-2-yl |
3j
|
60 |
12 |
H |
Naphth-1-yl |
3k
|
92 |
Entry |
Ar1 |
Ar2 |
Product |
Yield (%)b |
General reaction conditions: 4 (0.5 mmol), 2 (0.75 mmol) mixed in 1 : 1 EL : H2O, stirred at 80 °C for 2 h in the presence of 0.005 mmol Pd(OAc)2 and 1 mmol K2CO3.
Isolated yield based on 4.
|
1 |
Ph |
Ph |
3a
|
81 |
2 |
4-OHC6H4 |
Ph |
3l
|
93 |
3 |
3-MeOC6H4 |
Ph |
3m
|
76 |
4 |
4-AcC6H4 |
Ph |
3n
|
65 |
5 |
2-OHCC6H4 |
Ph |
3o
|
60 |
6 |
Ph |
4-MeOC6H4 |
3b
|
43 |
7 |
Ph |
4-FC6H4 |
3f
|
56 |
8 |
Ph |
Naphtha-1-yl |
3k
|
62 |
9 |
4-AcC6H4 |
4-MeC6H4 |
3p
|
81 |
10 |
4-AcC6H4 |
4-FC6H4 |
3q
|
88 |
11 |
2-OHCC6H4 |
4-ClC6H4 |
3r
|
96 |
12 |
2-OHCC6H4 |
4-FC6H4 |
3s
|
62 |
13 |
4-MeC6H4 |
4-ClC6H4 |
3t
|
43 |
14 |
3-MeOC6H4 |
Napth-1-yl |
3u
|
50 |
15 |
Naphtha-2-yl |
Ph |
3v
|
48 |
16 |
Pyridine-2-yl |
Ph |
3w
|
45 |
The practical reaction results of aryl boronic acids to both aryl iodides and bromides encouraged us to further explore the reactivity of different bromo- and iodoaryl dihalides to investigate the chemoselectivity of these bihaloaryls in the Suzuki–Miyaura coupling. Interestingly, when subjected to 80 °C heating in the presence of 2 equiv of phenylboronic acid, the mono-coupled biaryl products 3x–3z were obtained as single or major products and the doubly coupled triaryl product was not observed or isolated as a minor product (Scheme 1). The chemoselectivity was different from most previously reported catalyst protocols wherein triaryls were usually provided as the main or sole products.5k,7,18 The excellent chemoselectivity of our approach on the production of bromo-substituted biaryls was advantageous for the synthesis of unsymmetrical triaryls via stepwise cross coupling using different aryl boronic acids.
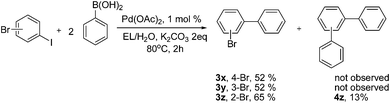 |
| Scheme 1 Chemoselective Suzuki–Miyaura reactions of bromoaryl iodides. | |
Conclusions
In conclusion, we developed a sustainable system consisting of EL–water media in the presence of 1 mol% Pd(OAc)2. This protocol tolerated the reactions of a broad array of aryl halides and aryl boronic acids under mild reaction conditions. Unique advantages of this methodology were the nontoxicity, no environmental impact, low cost and easy availability of the reaction media as well as ligand-free conditions. More importantly, since the biomass available EL was rarely employed in organic synthesis as an eco-friendly solvent, successful employment of EL as solvent for Suzuki–Miyaura reactions marks a notable extension on biomass organic solvent-based green synthesis. The present methodology also provides a useful complement to known catalyst systems for Suzuki–Miyaura reactions.
Experimental
General procedure for Suzuki–Miyaura reactions using aryl iodides
To a 25 mL round bottom flask was charged aryl iodide (0.5 mmol), aryl boronic acid (0.75 mmol), Pd(OAc)2 (0.005 mmol) and K2CO3 (1 mmol). EL (1 mL) and water (1 mL) were added and the mixture was heated at 60 °C for 2 h (TLC). After cooling down to room temperature, the mixture was extracted with ethyl acetate (3 × 10 mL). The combined organic phases were dried over anhydrous Na2SO4. The solvent was then removed under reduced pressure and the residue was subjected to silica gel chromatography to give pure product.
General procedure for Suzuki–Miyaura reactions using aryl bromides and bromophenyl iodides.
To a 25 mL round bottom flask was charged aryl bromide/bromophenyl iodide (0.5 mmol), aryl boronic acid (0.75 mmol), Pd(OAc)2 (0.005 mmol) and K2CO3 (1 mmol), EL (1 mL) and water (1 mL) were added and the mixture was heated at 80 °C for 2 h (TLC). After cooling down to room temperature, the mixture was extracted with ethyl acetate (3 × 10 mL). The combined organic phases were dried over anhydrous Na2SO4. The solvent was then removed under reduced pressure and the residue was subjected to silica gel chromatography to give pure product.
Acknowledgements
Financial support from NSFC of China (No. 21102059), NSF of Jiangxi Province (No. 20114BAB213005), a Sponsored Program for Cultivating Youths of Outstanding Ability in Jiangxi Normal University and a grant from the Open Project Program of Key Laboratory of Functional Small Organic Molecule, Ministry of Education, Jiangxi Normal University (No. KLFS-KF-201220) are gratefully acknowledged.
References
-
(a) N. Miyaura and A. Suzuki, J. Chem. Soc., Chem. Commun., 1979, 866–867 RSC;
(b) N. Miyaura, K. Yamada and A. Suzuki, Tetrahedron Lett., 1979, 20, 3437–3440 CrossRef.
-
(a) N. Miyaura and A. Suzuki, Chem. Rev., 1995, 95, 2457–2483 CrossRef CAS;
(b) A. Suzuki, Angew. Chem., Int. Ed., 2011, 50, 6722–6737 CrossRef CAS;
(c) J. Dupont, C. S. Consorti and J. Spencer, Chem. Rev., 2005, 105, 2527–2572 CrossRef CAS;
(d) L. Yin and J. Liebscher, Chem. Rev., 2007, 107, 133–173 CrossRef CAS;
(e) J. D. Sellars and P. G. Steel, Chem. Soc. Rev., 2011, 40, 5170–5180 RSC;
(f) A. Fihri, M. Bouhrara, B. Nekoueishahraki, J.-M. Basset and V. Polshettiwar, Chem. Soc. Rev., 2011, 40, 5181–5203 RSC;
(g) F. A. Littke and G. C. Fu, Angew. Chem., Int. Ed., 2002, 41, 4176–4211 CrossRef;
(h) S. Kotha and K. Mandal, Chem.–Asian J., 2009, 4, 354–362 CrossRef CAS.
-
(a) C. Zhou, J. Wang, L. Li, R. Wang and M. Hong, Green Chem., 2011, 13, 2100–2106 RSC;
(b) S.-S. Zhang, Z.-Q. Wang, M.-H. Xu and G.-Q. Lin, Org. Lett., 2010, 12, 5540–5549 Search PubMed;
(c) L.-W. Xu, X.-H. Chen, H. Shen, Y. Deng, J.-X. Jiang, K.-Z. Jiang, G.-Q. Lai and C.-Q. Shen, Eur. J. Org. Chem., 2012, 290–297 CrossRef CAS;
(d) C. Liu, Q. Ni, F. Bao and J. Qiu, Green Chem., 2011, 13, 1260–1266 RSC;
(e) C. Liu, Q. Ni, P. Hu and J. Qiu, Org. Biomol. Chem., 2011, 9, 1054–1060 RSC;
(f) Y. Kitamura, S. Sako, A. Tsutsui, Y. Monguchi, T. Maegawa, Y. Kitade and H. Sajiki, Adv. Synth. Catal., 2010, 351, 718–730 CrossRef;
(g) B. Basu, K. Biswas, S. Kundu and S. Ghosh, Green Chem., 2010, 12, 1734–1738 RSC.
-
(a) L. Wu, E. Drinkel, F. Gaggia, S. Capolicchio, A. Linden, L. Falivene, L. Cavallo and R. Dorta, Chem.–Eur. J., 2011, 17, 12886–12890 CrossRef CAS;
(b) B. H. Lipshutz, T. B. Petersen and A. R. Abela, Org. Lett., 2008, 7, 1333–1336 CrossRef;
(c) B. Karimi and P. F. Akhavan, Chem. Commun., 2011, 47, 4686–4688 Search PubMed;
(d) P. Das, C. Sarmah, A. Tairai and U. Bora, Appl. Organomet. Chem., 2011, 25, 283–288 CrossRef CAS;
(e) Y.-Y. Peng, J. Liu, X. Lei and Z. Yin, Green Chem., 2010, 12, 1072–1075 RSC.
-
(a) A. Fihri, D. Luart, C. Len, A. Solhy, C. Chevrin and V. Polshettiwar, Dalton Trans., 2011, 40, 3116–3121 RSC;
(b) M. Cui, J. Li, A. Yu, J. Zhang and Y. Wu, J. Mol. Catal. A: Chem., 2008, 290, 67–71 CrossRef CAS;
(c) O. Navarro, N. Marion, J. Mei and S. P. Nolan, Chem.–Eur. J., 2006, 19, 5142–5148 CrossRef;
(d) N. E. Leadbeater and M. Marco, Angew. Chem., Int. Ed., 2003, 12, 1407–1409 CrossRef;
(e) R. K. Arvela, N. E. Leadbeater and M. J. Collins, Tetrahedron, 2005, 61, 9349–9355 CrossRef CAS;
(f) K. Inamoto, C. Hasegawa, K. Hiroya, Y. Kondo, T. Osako, Y. Uozumi and T. Doi, Chem. Commun., 2012, 48, 2912–2914 RSC;
(g) R. K. Arvela, N. E. Leadbeater, M. S. Sangi, V. A. Williams, P. Granados and R. D. Singer, J. Org. Chem., 2005, 70, 161–168 CrossRef CAS;
(h) B. Karimi, D. Elhamifar, J. H. Clark and J. Hunt, Chem.–Eur. J., 2010, 16, 8047–8053 CAS;
(i) L. Wu, B.-L. Li, Y.-Y. Huang, H.-F. Zhou, Y.-M. He and Q.-H. Fan, Org. Lett., 2006, 8, 3605–3608 CrossRef CAS;
(j) D.-H. Lee, M. Choi, B.-W. Yu, R. Ryoo, A. Taher, S. Hossain and M. J. Jin, Adv. Synth. Catal., 2009, 351, 2912–2920 CrossRef CAS;
(k) J.-F. Wei, J. Jiao, J.-J. Feng, J. Lv, X.-R. Zhang, X.-Y. Shi and Z.-G. Chen, J. Org. Chem., 2009, 74, 6283–6286 CrossRef CAS.
-
(a) L. Liu, Y. Zhang and Y. Wang, J. Org. Chem., 2005, 70, 6122–6125 CrossRef CAS;
(b) S. Shi and Y. Zhang, Green Chem., 2008, 10, 868–872 RSC;
(c) A. L. F. de Souza, A. D. Silva and O. A. C. Autunes, Appl. Organomet. Chem., 2009, 23, 5–8 CrossRef CAS.
- L. Liu, Y. Zhang and B. Xin, J. Org. Chem., 2006, 71, 3994–3997 CrossRef CAS.
-
(a) B. Xin, Y. Zhang, L. Liu and Y. Wang, Synlett, 2005, 3083–3086 CAS;
(b) N. Liu, C. Liu and Z. Jin, Green Chem., 2012, 14, 592–597 RSC.
- I. Cobo, M. I. Matheu, S. Castillón, O. Boutureira and B. G. Davis, Org. Lett., 2012, 14, 1728–1731 CrossRef CAS.
-
(a) M. Gruttadauria, L. F. Liotta, A. M. P. Salvo, F. Giacalone, V. L. Parola, C. Aprile and R. Noto, Adv. Synth. Catal., 2011, 253, 2119–2130 CrossRef;
(b) T. Maegawa, Y. Kitamura, S. Sako, T. Udzu, A. Sakurai, A. Tanaka, Y. Kobayashi, K. Endo, U. Bora, T. Kurita, A. Kozaki, Y. Monguchi and H. Sajiki, Chem.–Eur. J., 2007, 13, 5937–5943 CrossRef CAS;
(c) C. Liu and W. Yang, Chem. Commun., 2009, 6267–6269 RSC;
(d) S. Li, Y. Lin, J. Cao and S. Zhang, J. Org. Chem., 2007, 72, 4067–4072 CrossRef CAS;
(e) C. A. Fleckenstein and H. Plenio, J. Org. Chem., 2008, 73, 3236–3244 CrossRef CAS.
-
(a) L. Botella and C. Nájera, Angew. Chem., Int. Ed., 2002, 41, 179–182 CrossRef CAS;
(b) J. M. Chalker, C. S. C. Wood and B. G. Davis, J. Am. Chem. Soc., 2009, 131, 16346–16347 CrossRef CAS;
(c) B. Yuan, Y. Pan, Y. Li, B. Yin and H. Jiang, Angew. Chem., Int. Ed., 2010, 49, 4054–4058 CrossRef CAS;
(d) J. Zhi, D. Song, Z. Li, X. Lei and A. Hu, Chem. Commun., 2011, 47, 10707–10709 RSC;
(e) A. N. Marziale, D. Jantke, S. H. Faul, T. Reiner, E. Herdtweck and J. Eppinger, Green Chem., 2011, 13, 169–177 RSC;
(f) N. E. Leadbeater, Chem. Commun., 2005, 2881–2092 RSC;
(g) N. E. Leadbeater and M. Marco, J. Org. Chem., 2003, 68, 888–892 CrossRef CAS;
(h) C. Baleizão, A. Corma, H. García and A. Leyva, Chem. Commun., 2003, 606–607 RSC.
- For a review on aqueous media-based Suzuki–Miyaura reaction, see: V. Polshettiwar, A. Decottignies, C. Len and A. Fihri, ChemSusChem, 2010, 3, 502–522 CrossRef CAS.
- P. G. Jessop, Green Chem., 2011, 13, 1391–1398 RSC.
- Y. Gu and F. Jérôme, Green Chem., 2010, 12, 1127–1138 RSC.
- J. I. García, H. García-Marín, J. A. Mayoral and P. Pérez, Green Chem., 2010, 12, 426–434 RSC.
-
(a) S. Aparicio and R. Alcalde, Green Chem., 2009, 11, 65–78 RSC;
(b) C. S. M. Pereira, V. M. T. M. Silva and R. E. Rodrigues, Green Chem., 2011, 13, 2658–2671 RSC.
- J. S. Bennett, K. L. Charles, M. R. Miner, C. F. Heuberger, E. J. Spina, M. F. Bartels and T. Foreman, Green Chem., 2009, 11, 166–168 RSC.
-
(a) S. R. Borhade and S. B. Waghmode, Beilstein J. Org. Chem., 2011, 7, 310–319 CrossRef CAS;
(b) N. Mejías, R. Pleixats, A. Shafir, M. Medio-Simón and G. Asensio, Eur. J. Org. Chem., 2010, 5090–5099 CrossRef;
(c) X.-H. Fan and L.-M. Yang, Eur. J. Org. Chem., 2011, 1467–1471 CrossRef CAS.
Footnote |
† Electronic Supplementary Information (ESI) available: General experimental information, characterization data as well as copies of 1H and 13C NMR spectra of all products. See DOI: 10.1039/c2ra21632a/ |
|
This journal is © The Royal Society of Chemistry 2012 |
Click here to see how this site uses Cookies. View our privacy policy here.