DOI:
10.1039/C2PY20656K
(Paper)
Polym. Chem., 2013,
4, 166-173
Synthesis of block polymer miktobrushes†
Received 16th August 2012, Accepted 12th September 2012
First published on 13th September 2012
Abstract
Well defined μ-A(BC)n ‘miktobrush’ terpolymers were synthesized utilizing the alternating radical copolymerization of two hydrophobic and incompatible macromonomer (MM) building blocks; a maleimide (MI) end functionalized poly(methyl-caprolactone) block (MI-PMCL) or ‘C’ and a styrene (Sty) end functionalized poly(perfluoro propylene oxide) block (Sty-PFPO) or ‘F’. Polymerizations were mediated by a poly(ethylene oxide) (PEO) functionalized reversible addition–fragmentation chain transfer (RAFT) agent (PEO–CTA) or ‘O’ to control the chain growth of the MMs from the O block to form O(CF)n “miktobrush” terpolymers. The synthesis of a range of well defined μ-O(CF)n terpolymers with various compositions was achieved by changing the feed of MMs. All building blocks and brush polymers were characterized by nuclear magnetic resonance (NMR) spectroscopy, size exclusion chromatography (SEC) and elemental analyses. This new strategy offers a powerful route towards a block polymer architecture that can enable the formation of multi-domain hierarchical nanostructures with features on multiple length scales due to the incompatibility and unique connectivity of the building blocks incorporated.
Block polymers can spontaneously self-assemble into well-defined nanostructures in block selective solvents and in the bulk, offering a powerful ‘bottom up’ approach towards precisely engineered materials for many diverse applications including; microelectronics,1 tissue engineering,2 drug delivery and water purification.3 The increased understanding of self-assembled block polymers with varied architectures is of both fundamental and technological importance.4 Towards this goal, AB diblock copolymers have been studied in depth.5–7 Adding more blocks and functional groups to AB diblock copolymers increases architectural and functional complexity and can lead to more exotic self-assembled morphologies.8,9 For instance, in a solvent selective for the A block, an ABC triblock terpolymer will form ‘multicompartment micelles’ whereby the two solvophobic (and incompatible) B and C blocks will produce two separate nanoscopic core domains.10,11If the chemistry of the microphase separated domains (B and C) is sufficiently different, multicompartment micelles can facilitate the storage of distinct small molecules within their separate core domains, thus enabling simultaneous storage and delivery of multiple incompatible payloads.12 Linear ABC terpolymers,13–19 linear BAC or ACB terpolymers,20 side chain grafted ABC terpolymers or ‘polysoaps’,21–23miktoarm star μ-ABC terpolymers,24–30 and blends of block polymers31–36 have been pursued as multicompartment micelle systems by various research groups. In each case, the connection point of the solvophobic B and C chains relative to the solvophilic corona forming block, A influences the micelle morphology to a great extent, although factors such as the solvophobicity and overall terpolymer composition play important roles.5,11
In μ-ABC terpolymers, three mutually immiscible blocks are joined at one common junction point. This architecture results in the suppression of concentric ABC or core–shell–corona micelles and the formation of two distinct B and C core domains.12,24–26,31,37–41 A rich variety of non-concentric micellar morphologies have been observed for μ-[poly(ethyl ethylene)]-[poly(ethylene oxide)]-[poly(perfluoro propylene oxide)] (μ-EOF) miktoarm stars, whereby the resultant multicompartment micelle morphology was dictated by the relative lengths of each block. These include; hamburger-like micelles,31 segmented worm-like micelles,26 compartmentalized vesicles,26,37 and polygonal bilayer sheets.26,37
Generally, the synthesis of μ-ABC stars can be more challenging than linear ABC polymers.24 Nonetheless, we recently prepared a range of μ-ABC block compositions by functionalizing the midpoint of an EO diblock copolymer with a hydroxyl initiator, from which ring opening polymerization (ROP) was performed to give poly(methyl-caprolactone) (PMCL) or a ‘C’ block, thus giving μ-EOC miktoarm star terpolymers with tuneable ‘C’ block lengths and thus different μ-EOC compositions. We subsequently studied the influence of the C block length on the solution morphology of μ-EOC stars in water with several different morphologies observable by cryo-TEM.39,41 Other research groups have also pioneered the synthesis of μ-ABC stars by facile and efficient ‘click chemistry’ methods,30,36,42–44 representing promising advancements towards fast and efficient syntheses of μ-ABC terpolymers.
We now present a synthetic strategy towards a wide compositional range of structurally well defined “miktobrush” block terpolymers, μ-A(BC)n obtained using rather mild and facile reaction conditions with three separate polymer building blocks. This new μ-A(BC)nmiktobrush architecture extends beyond the μ-ABC approach, and should also promote the formation of non-concentric solution morphologies due to the mandatory convergence of all three chains. In this approach we utilize the alternating copolymerization of two incompatible and hydrophobic end-functionalized polymers ‘B’ and ‘C’ (referred to as macromonomers or MMs) in the presence of an initiator species functionalized with a single hydrophilic chain ‘A’ to give μ-A(BC)n terpolymers with controlled composition.
As the number of repeat units of alternating BC units increases, the μ-A(BC)n terpolymers will enter a so called ‘brush-like’ regime of behaviour, whereby the backbone of the (BC)n copolymer will become increasingly rigid due to the steric constraints imparted by the grafted chains. Such ‘brush-like’ polymers45–48 have been studied in detail by various research groups, including Sheiko and Matyjaszewski,49–63 and by the groups of Grubbs,64–70 Rzayev,71–80 Bowden,81–85 Wooley,86–91 and others.92–96 The ‘grafting through’ approach to brush polymers involves the polymerization of MMs. We employ this strategy using alternating controlled radical copolymerization of two distinct MMs. We were inspired by the work of Chen and co-workers who investigated the alternating copolymerization of two different MMs bearing N-2-hydroxylethyl maleimide (MI) and styrene (Sty) functionalities; MI-PCL and Sty-PEO to form alternating copolymer brushes.97,98 The alternating copolymerizations of maleic anhydrides/N-substituted maleimides with styrene have been well studied,99–103 and even utilized in grafting from approaches towards brush copolymers or codendrimers.104–107 In Chen's study, the alternation of MI-PCL and Sty-PEO was inferred by determining the reactivity ratios of the MMs to be equivalent to each-other and close to the values of Sty and MI (ca. 0.1). Our approach to μ-A(BC)n materials exploits this alternating tendency of MI and Sty MMs and is depicted in Fig. 1 for MI-PMCL and Sty-PFPO MMs to give PEO-b-P((Sty-g-PFPO)-alt-(MI-g-PMCL)) or μ-O(CF)n terpolymers.
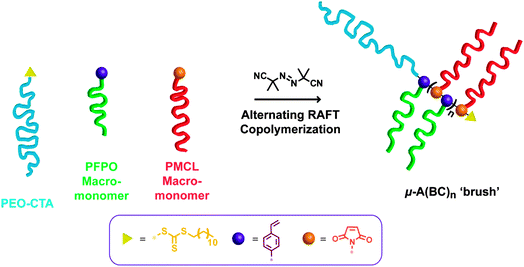 |
| Fig. 1 Alternating RAFT copolymerization strategy to synthesize μ-A(BC)nmiktobrush terpolymers. | |
Results and discussion
We selected a hydrophilic PEO block or ‘O’; a degradable polyester, PMCL or ‘C’; and a fluorocarbon block, PFPO or ‘F’ as the three building blocks for the μ-A(BC)n terpolymers based upon their anticipated incompatibility. To facilitate the alternating copolymerization strategy, the C block was end functionalized with a N-2-hydroxylethyl maleimide (MI) unit and the F block was end functionalized with a styrene (Sty) unit giving two MMs; MI-PMCL and Sty-PFPO (Fig. 2).107–110 To control the degree of polymerization of MI-PMCL and Sty-PFPO MMs (and molar mass/composition of μ-O(CF)n terpolymers), we performed the copolymerizations in the presence of PEO–CTA, a reversible addition–fragmentation chain transfer agent (RAFT CTA) functionalized with PEO.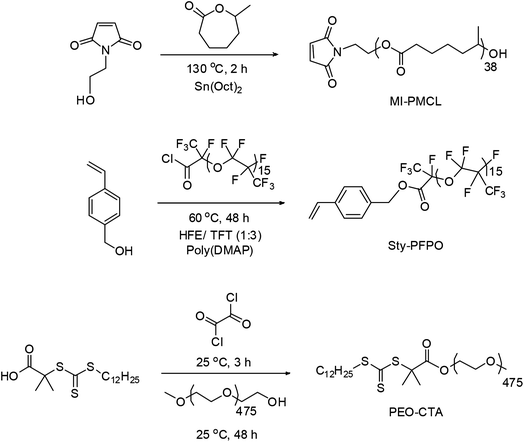 |
| Fig. 2 Synthesis of the three polymer building blocks used in this study; MI-PMCL and Sty-PFPO macromonomers (MMs) and PEO chain transfer agent (PEO–CTA). | |
Synthesis of MI-PMCL
MI-PMCL was synthesized using N-2-hydroxylethyl maleimide (MI) as an initiator for the ring-opening transesterification polymerization of MCL.111,112 The molar mass of MI-PMCL was determined to be 5.3 kDa by 1H NMR spectroscopy by comparing the MI end group signal intensities to the backbone PMCL signal intensities assuming one MI end group per chain (Fig. S1†). Consistent with this assumption, the MI end group signals shifted downfield from their original positions in the MI initiator. The molar mass dispersity (Đ) of MI-PMCL, was 1.18 by SEC (Fig. 6a) and the elemental analysis data was consistent with the structure depicted in Fig. 2 (see Table 1).
Table 1 MI-PMCL, Sty-PFPO and PEO–CTA molecular parameters
Sample | Na | MNMRna (kDa) | MMALSnb (kDa) | Đc | Elemental analysis calculated (elemental analysis found) |
---|
C% | H% | F% | N% | S% |
---|
As determined by 1H NMR spectroscopy in CDCl3, 1,1,2-trichlorofluoroethane (Freon-113) or CD2Cl2 respectively. Molar masses were determined by size exclusion chromatography (SEC) using a multi angle light scattering (MALS) detection system using literature dn/dc values for each block; PEO (0.135 mL g−1) and PMCL (0.12 mL g−1).113,114 Dispersities (Mw/Mn) were determined by size exclusion chromatography (SEC) using a refractive index detection system vs. polystyrene standards. Estimated from MALDI-ToF MS analysis.115 |
---|
MI-PMCL | 38 | 5.3 | 5.3 | 1.18 | 65.2 (65.0) | 9.3 (9.2) | | 0.3 (0.2) | |
Sty-PFPO | 15 | 2.6 | | 1.10d | 24.9 (24.7) | 0.4 (0.2) | 64.9 (64.9) | | |
PEO–CTA | 475 | 21.0 | 21.5 | 1.12 | 54.6 (54.4) | 9.2 (9.0) | | | 0.5 (0.2) |
Synthesis of Sty-PFPO
Sty-PFPO was synthesized by an esterfication reaction of PFPO–COCl24 and 4-vinyl benzyl alcohol116 with Poly(DMAP)® resin to uptake the liberated HCl. This procedure (see ESI†) routinely produced pure Sty-PFPO, as indicated by 1H, 19F and 13C NMR spectroscopy and elemental analyses (see Table 1). In the 1H NMR spectrum of Sty-PFPO (see Fig. S2†) the signal for the methylene group adjacent to the ester linkage to PFPO (CH2–CO–O) shifted downfield from the methylene signal in the 4-vinyl benzyl alcohol and showed no trace of the original peak. In the 13C NMR spectrum of Sty-PFPO, the peak corresponding to the methylene carbon atom (–CH2–) shifted downfield from its original position in the alcohol providing further evidence for exclusively ester formation (see Fig. S3†). The 19F NMR spectrum of Sty-PFPO is shown in Fig. S4.† The fluorine atom adjacent to the carbonyl group in PFPO–COCl exhibits a signal at δ = −126 ppm, and upon conversion to Sty-PFPO, this resonance shifts to δ = −135 ppm, which is expected for ester formation and consistent with 19F NMR analyses for similar coupling reactions between PFPO–COCl and alcohols performed by us previously.117–119 This signal integrated well with respect to backbone fluoro-ether group signals indicating quantitative end group conversion to styrene (Sty).Synthesis of PEO–CTA
PEO–CTA was synthesized in good yield and on multi gram scales as reported previously by our research groups (see ESI†).120,121 Briefly, S-1-dodecyl-S′-(α,α′-dimethyl-α′′-acetic acid)trithiocarbonate chain transfer agent (CTA) was reacted with oxalyl chloride followed by PEO-mono methyl ether. End group conversion of mono-methyl ether PEO to PEO–CTA was indicated by 1H NMR spectroscopy (Fig. S5†). Quantitative end group conversions were found in all syntheses of PEO–CTA, consistent with reports by our research groups previously.120,121 The molar mass distribution of PEO–CTA was compared to the original PEO mono-methyl ether by SEC. As can be seen from Fig. S6,† the peaks for both PEO blocks overlap, with little change between them indicating no change in distribution upon coupling of the CTA end-group.Synthesis of μ-O(CF)n terpolymers
To enable a controlled alternating copolymerization of Sty-PFPO and MI-PMCL MMs and incorporate a single PEO chain onto the terpolymer, the reaction mixture was required to be homogeneous throughout the course of the polymerization. We found MI-PMCL to be soluble in α,α,α-trifluorotoluene (TFT), Sty-PFPO soluble in methoxy-nonafluorobutane (HFE-7100®) and PEO–CTA soluble in chloroform (CHCl3). Furthermore, 1
:
1
:
1 ratios of TFT
:
HFE-7100®
:
CHCl3 (v/v/v) of solvents formed a homogeneous solution (by eye) at room temperature which could enable dissolution of a range of feed ratios of MMs and gave a homogeneous reaction mixture throughout the course of the copolymerization (Fig. 3). Organic solvents (like CHCl3) can inhibit the radical polymerization of monomers due to chain transfer between the growing polymer chain and solvent.122 However, the chain transfer constant, Cs for CHCl3 in the polymerization of styrene123 at 60 °C is 3.4 × 10−5 and given that high concentrations of PEO–CTA are utilized with a high chain-transfer constant, Ctr ≈ 140 (ref. 124) the vast majority of μ-O(CF)n polymer chains should contain a PEO ‘R group’ and a trithiocarbonate ‘Z group’ from the PEO–CTA. We note that steric hindrance can play a significant role in determining the fidelity of this approach if either large MMs or high degrees of polymerization for the alternating copolymer are targeted.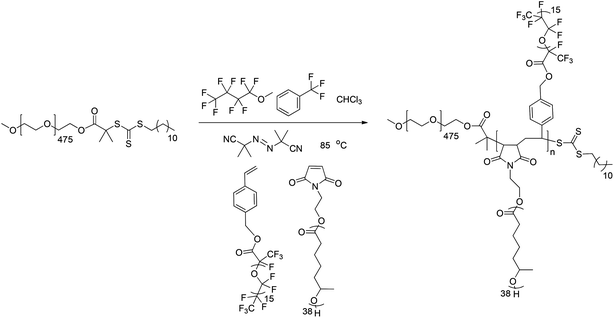 |
| Fig. 3 Alternating RAFT copolymerization of MI-PMCL and Sty-PFPO MMs using PEO–CTA to give μ-O(CF)n terpolymers. | |
To assess the alternating characteristics of the copolymerization of MMs, and prove the alternating microstructure of the (CF)n component of the μ-O(CF)n terpolymer, the reactivity ratios of the MMs were investigated. This was achieved by varying the feed ratio of the MMs during the copolymerizations and investigating the composition (by 1H NMR spectroscopy) of the resulting μ-O(CF)n terpolymer at a range of low conversions of MMs. We determined the reactivity ratios of each MM to be r1 = 0.063 and r2 = 0.070) (where M1 = MI-PMCL and M2 = Sty-PFPO) using the copolymerization equation and a non-linear regression fit, see Fig. 4.125 The values for MI-PMCL and Sty-PFPO are close to the values for MI-PCL and Sty-PEO MMs (r1 = r2 = 0.08) as reported by Chen and co-workers using the Fineman and Ross linear regression model.97
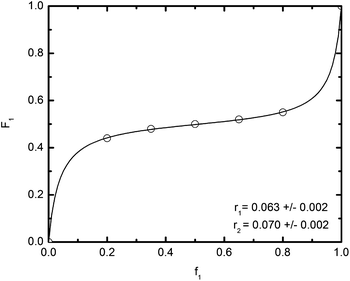 |
| Fig. 4 A nonlinear fit, F1 = (r1f12 + f1f2)/(r1f12 + 2f1f2 + r2f22), of the copolymerization data of MI-PMCL (monomer 1) and Sty-PFPO (monomer 2) to determine their reactivity ratios in the RAFT polymerization at 85 °C. The solvent utilized was a 1 : 1 : 1 (v/v/v) mixture of TFT : HFE-7100® : CHCl3. | |
Alternating copolymerizations of MMs were performed at 85 °C using AIBN as an initiator. These conditions enabled copolymerizations to be performed on practical timescales.97 We fixed the feed ratio of the two MMs to be 1
:
1 and varied the number of MM molar equivalents with respect to the PEO–CTA. By varying the equivalents of MMs from 5 to 80 we could access different degrees of polymerization of alternating MI-PMCL and Sty-PFPO units over the same time frame and for the same conversion, thus producing μ-O(CF)n block terpolymers with increasing numbers of repeating units, n (Table 2).
Table 2 Molecular parameters of μ-O(CF)n terpolymers synthesized. [PMCL]0/[PFPO]0 = 1
Formulaa | fPMClb | fPEOb | fPFPOb | MNMRna (kDa) | MMALSnc (kDa) | Đd |
---|
As determined by 1H NMR spectroscopy in (9 : 2) 1,1,2-trichlorofluoroethane (Freon-113)–CDCl3 and elemental analyses of the final purified terpolymer. Volume fractions of each block were calculated using the amorphous densities of each homopolymer at room temperature; ρ(PMCL) = 1.09 g cm−3, ρ(PFPO) = 1.90 g cm−3, ρ(PEO) = 1.12 g cm−3 (amorphous) and their molar masses as determined by 1H NMR spectroscopy. Molar masses were determined by size exclusion chromatography (SEC) using a multi angle light scattering (MALS) detection system using calculated dn/dc values for each terpolymer assuming that the total RI increment of the terpolymer is the sum of the weight average of the refractive index of its three constituent blocks. Literature values for each constituent homopolymer were used for the RI contributions; 0.135, 0.12 and 0.03 mL g−1 for PEO, PMCL and PFPO respectively.113–115 The insolubility of PFPO in THF prohibits determination of a dn/dc value for this polymer in this solvent. However, the low dn/dc value estimate (since all perfluoroethers have low dn/dc values in a range of solvents) and the small fraction of PFPO within the μ-O(CF)n terpolymers (≤0.26) means that the calculated dn/dc values/Mn by MALS for the terpolymers is insignificantly affected by the value for PFPO.126 Dispersities (Mw/Mn) of the terpolymers were determined by size exclusion chromatography (SEC) using a RI detection system vs. polystyrene standards. |
---|
μ-O(C2F3) | 0.28 | 0.59 | 0.13 | 39 | 35 | 1.20 |
μ-O(C3F2) | 0.39 | 0.54 | 0.08 | 42 | 38 | 1.18 |
μ-O(C2F4) | 0.27 | 0.56 | 0.16 | 42 | 49 | 1.10 |
μ-O(C3F4) | 0.36 | 0.50 | 0.15 | 47 | 60 | 1.32 |
μ-O(C5F8) | 0.43 | 0.36 | 0.21 | 68 | 70 | 1.10 |
μ-O(C7F13) | 0.46 | 0.28 | 0.26 | 92 | 98 | 1.14 |
μ-O(C15F20) | 0.59 | 0.17 | 0.24 | 153 | 146 | 1.11 |
μ-O(C33F38) | 0.68 | 0.09 | 0.24 | 295 | 287 | 1.14 |
To provide further evidence for alternation we developed a model based upon the copolymerization of MI and Sty small molecules MI-acetate (MI-Ac) and Sty-acetate (Sty-Ac) with near identical electronics to those in the MMs (as indicated by 1H NMR spectra comparisons of the monomers and the MMs, see Scheme S1 and Fig. S7 and S8†). We recognize that differing steric factors and solubility profiles of the MMs may change their copolymerization behaviour. We studied the copolymerization of MI-Ac and Sty-Ac in varying feed ratios and in the presence of a PEO–CTA. We then analyzed the microstructure of the resultant alternating copolymers by NMR spectroscopy. The 1H NMR spectrum of a copolymer isolated from a 50
:
50 feed ratio shows that the proton environments according to both MI-Ac and Sty-Ac are in near equal proportion, thus indicating a 1
:
1 ratio of MI-Ac/Sty-Ac (Fig. S9†). 13C NMR spectroscopy was also utilized (Fig. S10†) to compare poly(Sty-AC) and poly(MI-Ac) homopolymers and the poly((Sty-Ac)-alt-(MI-Ac)) copolymers. The chemical shift of the quaternary aryl carbon within Sty-Ac provides useful information about the microstructure of the copolymer since it is very sensitive to the electronic structure of adjacent monomers. When adjacent to the MI-Ac unit, this signal is characteristically shifted to δ = 138 ppm. These findings are very consistent with values found by Wang and co-workers who conducted an in-depth study of the alternating microstructure of dendritic poly(Sty-Ac)-alt-(MI-Ac) copolymers.106
In the synthesis of brush polymers using MMs unreacted MMs can be difficult to separate.46,48 As such, we developed an efficient method of purifying μ-O(CF)n polymers from MM impurities. Post synthesis, the crude polymer mixture was dissolved in chloroform to produce a concentrated and homogeneous solution that was precipitated into a (1
:
9 v/v) toluene
:
n-hexanes mixture at room temperature. This solvent mixture ensured efficient precipitation of μ-O(CF)n terpolymers and dissolution of MM impurities. The solvent mixture was decanted from the precipitated μ-O(CF)n terpolymer and the dissolution–precipitation–decanting process was repeated until all MM impurities were removed (as detectable by 1H NMR and SEC analyses). To synthesize terpolymers with high purity (<2% MM impurity), three precipitations were typically required.
Fig. 5 shows a representative 1H NMR spectrum of a μ-O(CF)n terpolymer. 1H NMR spectra for μ-O(CF)n terpolymers (see also Fig. S11–S14†) have a large singlet corresponding to the (CH2-CH2-O) PEO backbone signal. These signals were used as an integration calibration which enabled determination of the number of PMCL and PFPO chains and thus the molar mass and composition (see ESI† for details). Furthermore, vinyl signals from the Sty and MI end groups were absent in the spectra for the terpolymers indicating that any Sty-PFPO and MI-PMCL had been incorporated into the backbone of the terpolymer and were not present as their respective MMs. This also gave a good indication of the purity of the terpolymer samples upon purification i.e. no MM impurities remained. We further confirmed the composition of the μ-O(CF)n terpolymers by elemental analyses and the data was generally very consistent with that found by 1H NMR analysis (see Table S1†).
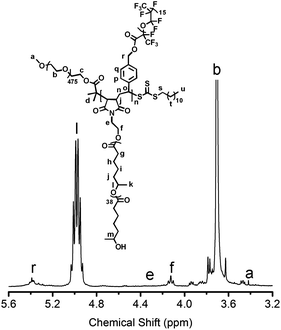 |
| Fig. 5 1H NMR spectrum of μ-O(C15F20) terpolymer in (9 : 2, v/v) 1,1,2-trichlorofluoroethane (Freon-113)–CDCl3. | |
The molar mass (MMALSn) and molar mass distributions (Đ) of μ-O(CF)n terpolymers were analysed by size exclusion chromatography (SEC) in THF (Table 2). μ-O(CF)n terpolymers showed narrow and symmetrical traces by SEC with no indication of the presence of MM impurities, consistent with 1H NMR data (Fig. 6a). We analysed each terpolymer using triple detection; UV-vis, refractive index (RI) and multi angle light scattering (MALS), see Fig. 6b. We analyzed absolute molar masses by MALS, purity and Đ by RI and RAFT CTA end group retention by UV-vis at 310 nm for each terpolymer. RAFT end group fidelity was good for the majority of terpolymers and absolute molar masses determined by MALS were in good agreement with those found by 1H NMR and elemental analyses (Table S1†).
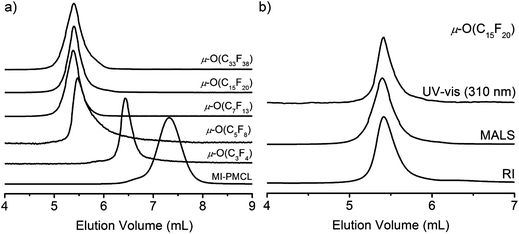 |
| Fig. 6 (a) Representative SEC traces of polymers (b) representative SEC traces with triple detection for μ-O(C15F20) terpolymer. | |
Conclusions
We have described a versatile synthetic protocol for the preparation of three end functionalized homopolymer ‘building blocks’ which when combined under alternating controlled radical copolymerization conditions yield a new class of ‘miktobrush’ μ-O(CF)n block terpolymers. A range of well-defined block lengths, compositions and molar masses have been synthesized and characterized by SEC, NMR spectroscopy and elemental analyses. We anticipate interesting self-assembly behaviour of these terpolymers in block selective solvents and in bulk as a consequence of the extremely strong segregation between the constituent segments and their unique connectivity.Acknowledgements
We acknowledge support from the MRSEC Program of the National Science Foundation, through Award DMR-8019885. Partial support from the Center for Sustainable Polymers, a National Science Foundation supported Center for Chemical Innovation (CHE-1136607) is also acknowledged. Parts of this work were carried out in the Institute of Technology Characterization Facility, University of Minnesota, a member of the NSF-funded Materials Research Facilities Network.References
- J. L. Hedrick, T. Magbitang, E. F. Connor, T. Glauser, W. Volksen, C. J. Hawker, V. Y. Lee and R. D. Miller, Chem.–Eur. J., 2002, 8, 3308–3319 CrossRef CAS.
- E. S. Place, J. H. George, C. K. Williams and M. M. Stevens, Chem. Soc. Rev., 2009, 38, 1139–1151 RSC.
- E. A. Jackson and M. A. Hillmyer, ACS Nano, 2010, 4, 3548–3553 CrossRef CAS.
- C. K. Ober, S. Z. D. Cheng, P. T. Hammond, M. Muthukumar, E. Reichmanis, K. L. Wooley and T. P. Lodge, Macromolecules, 2008, 42, 465–471 CrossRef.
- N. S. Cameron, M. K. Corbierre and A. Eisenberg, Can. J. Chem., 1999, 77, 1311–1326 CAS.
- S. Jain and F. S. Bates, Science, 2003, 300, 460–464 CrossRef CAS.
- T. P. Lodge, Macromol. Chem. Phys., 2003, 204, 265–273 CrossRef CAS.
- F. S. Bates and G. H. Fredrickson, Phys. Today, 1999, 52, 32–38 CrossRef CAS.
- F. S. Bates, M. A. Hillmyer, T. P. Lodge, C. M. Bates, K. T. Delaney and G. H. Fredrickson, Science, 2012, 336, 434–440 CrossRef CAS.
- J.-F. Lutz and A. Laschewsky, Macromol. Chem. Phys., 2005, 206, 813–817 CrossRef CAS.
- A. O. Moughton, M. A. Hillmyer and T. P. Lodge, Macromolecules, 2012, 45, 2–19 CrossRef CAS.
- T. P. Lodge, A. Rasdal, Z. Li and M. A. Hillmyer, J. Am. Chem. Soc., 2005, 127, 17608–17609 CrossRef CAS.
- G.-E. Yu and A. Eisenberg, Macromolecules, 1998, 31, 5546–5549 CrossRef CAS.
- T. P. Lodge, M. A. Hillmyer, Z. Zhou and Y. Talmon, Macromolecules, 2004, 37, 6680–6682 CrossRef CAS.
- S. Kubowicz, J.-F. Baussard, J.-F. Lutz, A. F. Thünemann, H. von Berlepsch and A. Laschewsky, Angew. Chem., Int. Ed., 2005, 44, 5262–5265 CrossRef CAS.
- I. Katsampas, Y. Roiter, S. Minko and C. Tsitsilianis, Macromol. Rapid Commun., 2005, 26, 1371–1376 CrossRef CAS.
- G. Njikang, D. Han, J. Wang and G. Liu, Macromolecules, 2008, 41, 9727–9735 CrossRef CAS.
- H. von Berlepsch, C. Bottcher, K. Skrabania and A. Laschewsky, Chem. Commun., 2009, 2290–2292 RSC.
- A. Walther, C. Barner-Kowollik and A. H. E. Müller, Langmuir, 2010, 26, 12237–12246 CrossRef CAS.
- K. Skrabania, H. von Berlepsch, C. Böttcher and A. Laschewsky, Macromolecules, 2010, 43, 271–281 CrossRef CAS.
- A. Kotzev, A. Laschewsky and R. H. Rakotoaly, Macromol. Chem. Phys., 2001, 202, 3257–3267 CrossRef CAS.
- A. Kotzev, A. Laschewsky, P. Adriaensens and J. Gelan, Macromolecules, 2002, 35, 1091–1101 CrossRef CAS.
- A. Laschewsky, Curr. Opin. Colloid Interface Sci., 2003, 8, 274–281 CrossRef CAS.
- Z. Li, M. A. Hillmyer and T. P. Lodge, Macromolecules, 2004, 37, 8933–8940 CrossRef CAS.
- Z. Li, E. Kesselman, Y. Talmon, M. A. Hillmyer and T. P. Lodge, Science, 2004, 306, 98–101 CrossRef CAS.
- Z. Li, M. A. Hillmyer and T. P. Lodge, Langmuir, 2006, 22, 9409–9417 CrossRef CAS.
- K. V. Butsele, C. A. Fustin, J. F. Gohy, R. Jérôme and C. Jérôme, Langmuir, 2008, 25, 107–111 CrossRef.
- Y. Zhang, H. Liu, J. Hu, C. Li and S. Liu, Macromol. Rapid Commun., 2009, 30, 941–947 CrossRef CAS.
- A. Walther and A. H. E. Müller, Chem. Commun., 2009, 1127–1129 RSC.
- C. Li, Z. Ge, H. Liu and S. Liu, J. Polym. Sci., Part A: Polym. Chem., 2009, 47, 4001–4013 CrossRef CAS.
- Z. Li, M. A. Hillmyer and T. P. Lodge, Macromolecules, 2006, 39, 765–771 CrossRef CAS.
- J. Xin, D. Liu and C. Zhong, J. Phys. Chem. B, 2007, 111, 13675–13682 CrossRef CAS.
- J. W. Ma, X. Li, P. Tang and Y. Yang, J. Phys. Chem. B, 2007, 111, 1552–1558 CrossRef CAS.
- J. Zhu and R. C. Hayward, Macromolecules, 2008, 41, 7794–7797 CrossRef CAS.
- J. Xin, D. Liu and C. Zhong, J. Phys. Chem. B, 2009, 113, 9364–9372 CrossRef CAS.
- B. Iskin, G. Yilmaz and Y. Yagci, Polym. Chem., 2011, 2, 2865–2871 RSC.
- Z. Li, M. A. Hillmyer and T. P. Lodge, Nano Lett., 2006, 6, 1245–1249 CrossRef CAS.
- C. Liu, M. A. Hillmyer and T. P. Lodge, Langmuir, 2008, 24, 12001–12009 CrossRef CAS.
- N. Saito, C. Liu, T. P. Lodge and M. A. Hillmyer, Macromolecules, 2008, 41, 8815–8822 CrossRef CAS.
- C. Liu, M. A. Hillmyer and T. P. Lodge, Langmuir, 2009, 25, 13718–13725 CrossRef CAS.
- N. Saito, C. Liu, T. P. Lodge and M. A. Hillmyer, ACS Nano, 2010, 4, 1907–1912 CrossRef CAS.
- Y. Zhang, C. Li and S. Liu, J. Polym. Sci., Part A: Polym. Chem., 2009, 47, 3066–3077 CrossRef CAS.
- K. Khanna, S. Varshney and A. Kakkar, Macromolecules, 2010, 43, 5688–5698 CrossRef CAS.
- Y.-Y. Yuan, Q. Du, Y.-C. Wang and J. Wang, Macromolecules, 2010, 43, 1739–1746 CrossRef CAS.
- M. Zhang and A. H. E. Müller, J. Polym. Sci., Part A: Polym. Chem., 2005, 43, 3461–3481 CrossRef CAS.
- S. S. Sheiko, B. S. Sumerlin and K. Matyjaszewski, Prog. Polym. Sci., 2008, 33, 759–785 CrossRef CAS.
- H.-i. Lee, J. Pietrasik, S. S. Sheiko and K. Matyjaszewski, Prog. Polym. Sci., 2010, 35, 24–44 CrossRef CAS.
- Y. Chen, Macromolecules, 2012, 45, 2619–2631 CrossRef CAS.
- H. G. Börner, D. Duran, K. Matyjaszewski, M. da Silva and S. S. Sheiko, Macromolecules, 2002, 35, 3387–3394 CrossRef.
- S. S. Sheiko, M. da Silva, D. Shirvaniants, I. LaRue, S. Prokhorova, M. Moeller, K. Beers and K. Matyjaszewski, J. Am. Chem. Soc., 2003, 125, 6725–6728 CrossRef CAS.
- J. R. Boyce, D. Shirvanyants, S. S. Sheiko, D. A. Ivanov, S. Qin, H. Börner and K. Matyjaszewski, Langmuir, 2004, 20, 6005–6011 CrossRef CAS.
- S. J. Lord, S. S. Sheiko, I. LaRue, H.-i. Lee and K. Matyjaszewski, Macromolecules, 2004, 37, 4235–4240 CrossRef CAS.
- H.-i. Lee, W. Jakubowski, K. Matyjaszewski, S. Yu and S. S. Sheiko, Macromolecules, 2006, 39, 4983–4989 CrossRef CAS.
- S. S. Sheiko, F. C. Sun, A. Randall, D. Shirvanyants, M. Rubinstein, H.-i. Lee and K. Matyjaszewski, Nature, 2006, 440, 191–194 CrossRef CAS.
- H. Gao and K. Matyjaszewski, J. Am. Chem. Soc., 2007, 129, 6633–6639 CrossRef CAS.
- K. Min, S. Yu, H.-i. Lee, L. Mueller, S. S. Sheiko and K. Matyjaszewski, Macromolecules, 2007, 40, 6557–6563 CrossRef CAS.
- H.-i. Lee, K. Matyjaszewski, S. Yu-Su and S. S. Sheiko, Macromolecules, 2008, 41, 6073–6080 CrossRef CAS.
- N. V. Lebedeva, F. C. Sun, H.-i. Lee, K. Matyjaszewski and S. S. Sheiko, J. Am. Chem. Soc., 2008, 130, 4228–4229 CrossRef CAS.
- S. Y. Yu-Su, S. S. Sheiko, H.-i. Lee, W. Jakubowski, A. Nese, K. Matyjaszewski, D. Anokhin and D. A. Ivanov, Macromolecules, 2009, 42, 9008–9017 CrossRef CAS.
- A. Nese, Y. Kwak, R. Nicolaÿ, M. Barrett, S. S. Sheiko and K. Matyjaszewski, Macromolecules, 2010, 43, 4016–4019 CrossRef CAS.
- A. Nese, N. V. Lebedeva, G. Sherwood, S. Averick, Y. Li, H. Gao, L. Peteanu, S. S. Sheiko and K. Matyjaszewski, Macromolecules, 2011, 44, 5905–5910 CrossRef CAS.
- H. Tang, Y. Li, S. H. Lahasky, S. S. Sheiko and D. Zhang, Macromolecules, 2011, 44, 1491–1499 CrossRef CAS.
- A. Nese, Y. Li, S. Averick, Y. Kwak, D. Konkolewicz, S. S. Sheiko and K. Matyjaszewski, ACS Macro Lett., 2012, 1, 227–231 CrossRef CAS.
- A. Sundararaman, T. Stephan and R. B. Grubbs, J. Am. Chem. Soc., 2008, 130, 12264–12265 CrossRef CAS.
- Y. Xia, J. A. Kornfield and R. H. Grubbs, Macromolecules, 2009, 42, 3761–3766 CrossRef CAS.
- Y. Xia, B. D. Olsen, J. A. Kornfield and R. H. Grubbs, J. Am. Chem. Soc., 2009, 131, 18525–18532 CrossRef CAS.
- J. A. Johnson, Y. Y. Lu, A. O. Burts, Y. Xia, A. C. Durrell, D. A. Tirrell and R. H. Grubbs, Macromolecules, 2010, 43, 10326–10335 CrossRef CAS.
- Y. Xia, A. J. Boydston and R. H. Grubbs, Angew. Chem., Int. Ed., 2011, 50, 5882–5885 CrossRef CAS.
- Y. Xia, Y. Li, A. O. Burts, M. F. Ottaviani, D. A. Tirrell, J. A. Johnson, N. J. Turro and R. H. Grubbs, J. Am. Chem. Soc., 2011, 133, 19953–19959 CrossRef CAS.
- J. A. Johnson, Y. Y. Lu, A. O. Burts, Y.-H. Lim, M. G. Finn, J. T. Koberstein, N. J. Turro, D. A. Tirrell and R. H. Grubbs, J. Am. Chem. Soc., 2011, 133, 559–566 CrossRef CAS.
- L. Zhao, M. Byun, J. Rzayev and Z. Lin, Macromolecules, 2009, 42, 9027–9033 CrossRef CAS.
- K. Huang and J. Rzayev, J. Am. Chem. Soc., 2009, 131, 6880–6885 CrossRef CAS.
- J. Rzayev, Macromolecules, 2009, 42, 2135–2141 CrossRef CAS.
- K. Huang, D. P. Canterbury and J. Rzayev, Chem. Commun., 2010, 46, 6326–6328 RSC.
- K. Huang, D. P. Canterbury and J. Rzayev, Macromolecules, 2010, 43, 6632–6638 CrossRef CAS.
- K. Huang, A. Jacobs and J. Rzayev, Biomacromolecules, 2011, 12, 2327–2334 CrossRef CAS.
- K. Huang and J. Rzayev, J. Am. Chem. Soc., 2011, 133, 16726–16729 CrossRef CAS.
- W. Han, M. Byun, L. Zhao, J. Rzayev and Z. Lin, J. Mater. Chem., 2011, 21, 14248–14253 RSC.
- J. Bolton, T. S. Bailey and J. Rzayev, Nano Lett., 2011, 11, 998–1001 CrossRef CAS.
- J. Bolton and J. Rzayev, ACS Macro Lett., 2012, 1, 15–18 CrossRef CAS.
- S. Jha, S. Dutta and N. B. Bowden, Macromolecules, 2004, 37, 4365–4374 CrossRef CAS.
- M. B. Runge, S. Dutta and N. B. Bowden, Macromolecules, 2005, 39, 498–508 CrossRef.
- M. B. Runge and N. B. Bowden, J. Am. Chem. Soc., 2007, 129, 10551–10560 CrossRef CAS.
- M. B. Runge, C. E. Lipscomb, L. R. Ditzler, M. K. Mahanthappa, A. V. Tivanski and N. B. Bowden, Macromolecules, 2008, 41, 7687–7694 CrossRef CAS.
- M. Byun, N. B. Bowden and Z. Lin, Nano Lett., 2010, 10, 3111–3117 CrossRef CAS.
- C. Cheng, K. Qi, E. Khoshdel and K. L. Wooley, J. Am. Chem. Soc., 2006, 128, 6808–6809 CrossRef CAS.
- C. Cheng, E. Khoshdel and K. L. Wooley, Nano Lett., 2006, 6, 1741–1746 CrossRef CAS.
- C. Cheng, K. Qi, D. S. Germack, E. Khoshdel and K. L. Wooley, Adv. Mater., 2007, 19, 2830–2835 CrossRef CAS.
- Z. Li, K. Zhang, J. Ma, C. Cheng and K. L. Wooley, J. Polym. Sci., Part A: Polym. Chem., 2009, 47, 5557–5563 CrossRef CAS.
- A. Li, Z. Li, S. Zhang, G. Sun, D. M. Policarpio and K. L. Wooley, ACS Macro Lett., 2012, 1, 241–245 CrossRef CAS.
- A. Li, J. Ma, G. Sun, Z. Li, S. Cho, C. Clark and K. L. Wooley, J. Polym. Sci., Part A: Polym. Chem., 2012, 50, 1681–1688 CrossRef CAS.
- G. Cheng, A. Böker, M. Zhang, G. Krausch and A. H. E. Müller, Macromolecules, 2001, 34, 6883–6888 CrossRef CAS.
- N. Gunari, M. Schmidt and A. Janshoff, Macromolecules, 2006, 39, 2219–2224 CrossRef CAS.
- M. Schappacher and A. Deffieux, Science, 2008, 319, 1512–1515 CrossRef CAS.
- Y. Li, E. Themistou, J. Zou, B. P. Das, M. Tsianou and C. Cheng, ACS Macro Lett., 2012, 1, 52–56 CrossRef CAS.
- M. Sahl, S. Muth, R. Branscheid, K. Fischer and M. Schmidt, Macromolecules, 2012, 45, 5167–5175 CrossRef CAS.
- H. Zhu, G. Deng and Y. Chen, Polymer, 2008, 49, 405–411 CrossRef CAS.
- N. Xia, G. Zhang, T. Li, W. Wang, H. Zhu, Y. Chen and G. Deng, Polymer, 2011, 52, 4581–4589 CrossRef CAS.
- A. Matsumoto, T. Kubota and T. Otsu, Macromolecules, 1990, 23, 4508–4513 CrossRef CAS.
- M. El-Guweri, P. Hendlinger and A. Laschewsky, Macromol. Chem. Phys., 1997, 198, 401–418 CrossRef CAS.
- S. Pfeifer and J.-F. Lutz, Chem.–Eur. J., 2008, 14, 10949–10957 CrossRef CAS.
- J.-F. Lutz, Polym. Chem., 2010, 1, 55–62 RSC.
- J. Weiss, A. Li, E. Wischerhoff and A. Laschewsky, Polym. Chem., 2012, 3, 352–361 RSC.
- K. Ishizu, C. Takashimizu, T. Shibuya and S. Uchida, Polym. Int., 2003, 52, 1010–1015 CrossRef CAS.
- T. Çakir, I. E. Serhatli and A. Önen, J. Appl. Polym. Sci., 2006, 99, 1993–2001 CrossRef.
- X. Xiong, Y. Chen, S. Feng and W. Wang, Macromolecules, 2007, 40, 9084–9093 CrossRef CAS.
- G. Deng and Y. Chen, J. Polym. Sci., Part A: Polym. Chem., 2009, 47, 5527–5533 CrossRef CAS.
- Y. Shi, Z. Fu and W. Yang, J. Polym. Sci., Part A: Polym. Chem., 2006, 44, 2069–2075 CrossRef CAS.
- S. Pfeifer and J.-F. Lutz, J. Am. Chem. Soc., 2007, 129, 9542–9543 CrossRef CAS.
- S. Pfeifer and J.-F. Lutz, Chem.–Eur. J., 2008, 14, 10949–10957 CrossRef CAS.
- R. J. Pounder, M. J. Stanford, P. Brooks, S. P. Richards and A. P. Dove, Chem. Commun., 2008, 5158–5160 RSC.
- M. T. Martello and M. A. Hillmyer, Macromolecules, 2011, 44, 8537–8545 CrossRef CAS.
- Y. Matter, R. Enea, O. Casse, C. C. Lee, J. Baryza and W. Meier, Macromol. Chem. Phys., 2011, 212, 937–949 CrossRef CAS.
- J. Braun, N. Bruns, T. Pfohl and W. Meier, Macromol. Chem. Phys., 2011, 212, 1245–1254 CrossRef CAS.
- Z. Li, Ph.D. thesis, University of Minnesota, 2006.
- R. He, P. H. Toy and Y. Lam, Adv. Synth. Catal., 2008, 350, 54–60 CrossRef CAS.
- S. Zhu, W. F. Edmonds, M. A. Hillmyer and T. P. Lodge, J. Polym. Sci., Part B: Polym. Phys., 2005, 43, 3685–3694 CrossRef CAS.
- R. R. Taribagil, M. A. Hillmyer and T. P. Lodge, Macromolecules, 2009, 42, 1796–1800 CrossRef CAS.
- R. R. Taribagil, M. A. Hillmyer and T. P. Lodge, Macromolecules, 2010, 43, 5396–5404 CrossRef CAS.
- Y. He, P. G. Boswell, P. Bühlmann and T. P. Lodge, J. Phys. Chem. B, 2006, 111, 4645–4652 CrossRef.
- Y. He and T. P. Lodge, Macromolecules, 2007, 41, 167–174 CrossRef.
- F. R. Mayo, J. Am. Chem. Soc., 1943, 65, 2324–2329 CrossRef CAS.
- G. Odian, Principles of Polymerization, John Wiley & Sons, Inc, Hoboken, NJ, 4th edn, 2004, p. 247 Search PubMed.
- G. Moad, E. Rizzardo and S. H. Thang, Aust. J. Chem., 2009, 62, 1402–1472 CrossRef CAS.
- G. Odian, Principles of Polymerization, John Wiley & Sons, Inc., Hoboken, NJ, 4th edn, 2004, p. 481 Search PubMed.
- A. Sanguineti, P. A. Guarda, G. Marchionni and G. Ajroldi, Polymer, 1995, 36, 3697–3703 CrossRef CAS.
Footnote |
† Electronic supplementary information (ESI) available. See DOI: 10.1039/c2py20656k |
|
This journal is © The Royal Society of Chemistry 2013 |
Click here to see how this site uses Cookies. View our privacy policy here.