DOI:
10.1039/C2SC21085A
(Edge Article)
Chem. Sci., 2013,
4, 97-102
Diversity oriented synthesis of indole-based peri-annulated compounds via allylic alkylation reactions†
Received
26th July 2012
, Accepted 23rd August 2012
First published on 24th August 2012
Abstract
Diverse indole-based peri-annulated compounds were synthesized via transition-metal-catalyzed allylic alkylation reaction. With palladium catalyst, indole-based nine-membered ring products were obtained in 40–70% yields. When iridium catalyst was used, highly enantioenriched seven-membered ring products were obtained in 40–78% yields and 91–97% ee. Meanwhile, when 3-substituted indole substrates were employed with a palladium catalyst, asymmetric allylic dearomatization of indoles occurred with products obtained in 48–78% yields and 35–78% ee with a chiral ferrocene-based Phox ligand. Interestingly, with an iridium catalyst, Friedel–Crafts type allylic alkylation reaction proceeded at the C5 position of indole, affording the products with 40–60% yields and 56–97% ee.
Diversity oriented synthesis is of great importance in organic synthesis, agrochemical chemistry, pharmaceutical chemistry, material sciences, etc. It provides quick access to structurally diversified chemical scaffolds from the same or commonly used starting materials.1 The success of diversity synthesis of various scaffolds from the same starting materials requires the precise control of the chemo-, regio- and enantio-selectivity, careful selection of the reaction conditions, as well as understanding the reactivities of both catalysts and substrates. Therefore, the development of diversity synthesis is highly desirable but poses great challenges. Meanwhile, chiral indole-based peri-annulated systems exist widely in natural products2 such as hyrtiazepine,3 aurantioclavine4 and clavicipitic acid5 (Fig. 1). Quite contrary to their great importance, limited methods are available for the synthesis of these indole-based peri-annulated frameworks, especially in an enantiopure form.
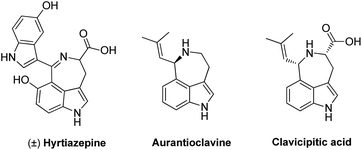 |
| Fig. 1 Structures of naturally existing indole-based peri-annulated frameworks. | |
Several methods such as intramolecular Friedel–Crafts alkylation reaction,6 Pictet–Spengler reaction7 and transition-metal-catalyzed cross-coupling reaction8 have been successfully applied in constructing polycyclic indole structures fused through C3 and C4.9 However, the size control of ring formation and the discovery of new reaction sites of indole represent a huge challenge. To our knowledge, enantioselective Friedel–Crafts alkylation reaction occurring at the C5 position of indole has not been reported before.
Transition-metal-catalyzed intramolecular allylic substitution reactions have been utilized to construct indole-based cyclic frameworks but with limited reports.10 This mismatches the fact that transition-metal-catalyzed allylic substitution reaction has been recognized as one of the most efficient methods in constructing carbon–carbon and carbon–heteroatom bonds in organic synthesis.11 Notably, for unsymmetrical allylic substrates, palladium catalysts tend to give the linear products (path A, Scheme 1),12 but other metals such as Ir,13 Rh,14 Mo,15 W,16 and Ru17 could favor the formation of optically active branched products with the aid of appropriate chiral ligands (path B, Scheme 1). These distinctive reactivities varying the metals provide an opportunity for construction of diverse scaffolds from the same starting materials.
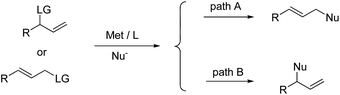 |
| Scheme 1 Transition-metal-catalyzed allylic substitution reactions with unsymmetrical allyl substrates. | |
In this paper, we report the diverse synthesis of indole-based peri-annulated compounds via transition-metal-catalyzed allylic alkylation reaction. From the 4-indolyl allylic carbonates, by different choices of iridium or palladium complex, four types of alkylation products varying the reaction site of indole and ring size could be obtained (Scheme 2).
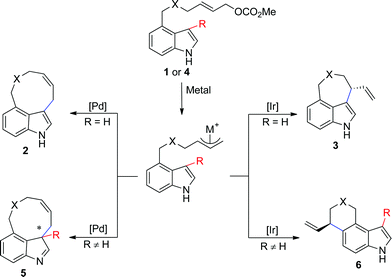 |
| Scheme 2 Proposed diversity oriented synthesis of indole-based peri-annulated structures. | |
Palladium-catalyzed Friedel–Crafts type allylic alkylation reaction of indole fused through C4–C3
Indolyl allyl carbonate 1a was chosen as a model substrate for the initial study. The reaction of 1a with 5 mol% of [Pd(C3H5)Cl]2 and 11 mol% of Phox ligand L1 in the presence of Cs2CO3 in THF at 50 °C afforded 2a in 36% yield as expected (entry 1, Table 1). The reactions with various ligands such as L3, L6, dppb, dppf, dppp and PPh3 all led to the alkylated products. The reaction with ligand L6 resulted in the best selectivity (>97/3) favoring the formation of nine-membered ring product 2a (entry 6, Table 1).
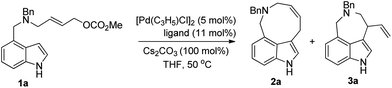
|
Entry |
Ligand |
t/h |
2a/3ab |
Yieldc (%) |
Reaction conditions: 5 mol% of [Pd(C3H5)Cl]2, 11 mol% of ligand, 0.2 mmol of 1a, and 100 mol% of Cs2CO3 in THF (2 mL) at 50 °C.
Determined by 1H NMR of the crude reaction mixture.
Isolated yield of 2a.
10 mol% of Pd(PPh3)4 was used.
KOAc was used as the base.
|
1 |
L1
|
12 |
>97/3 |
36 |
2 |
L2
|
12 |
— |
NR |
3 |
L3
|
12 |
80/20 |
45 |
4 |
L4
|
12 |
— |
Complex |
5 |
L5
|
12 |
— |
NR |
6 |
L6
|
12 |
>97/3 |
32 |
7 |
(R)-BINAP |
12 |
— |
Complex |
8 |
(R)-Tol-BINAP |
12 |
— |
Complex |
9 |
dppb |
12 |
94/6 |
33 |
10 |
dppf |
12 |
80/20 |
26 |
11 |
dppp |
12 |
84/16 |
38 |
12d |
— |
12 |
87/13 |
48 |
13e |
L6
|
2 |
>97/3 |
70 |
Further studies on the effect of bases and solvents were carried out (see ESI† for details), and the use of KOAc as base in THF gave the best result (2a/3a > 97/3, 70% yield, entry 13, Table 1). Under the optimized reaction conditions [5 mol% of [Pd(C3H5)Cl]2, 11 mol% of L6, 100 mol% of KOAc, THF, 50 °C], various substrates were tested to examine the scope of the reaction. As to substituents on the nitrogen, both benzyl and allyl groups could be tolerated, although only moderate yield (40%) was obtained with allyl protected substrate 1b (entry 2, Table 2). Reaction of 7-substituted indole substrates generated the products with good yields (entries 3–5, Table 2). 2-Substituted indoles with either aryl or aliphatic group could be used in the reaction. With aliphatic groups, the products were obtained in moderate yields (entries 9 and 10, Table 2). The carbon tethered substrate 1k could also be used in the reaction, affording the product in 70% yield (entry 11, Table 2). Notably, all the yields are reported referring to the isolated yields of the nine-membered ring products 2.
Table 2 Substrate scope for Pd-catalyzed Friedel–Crafts type allylic alkylation of indole fused through C4–C3a
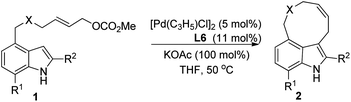
|
Entry |
1, X, R1, R2 |
t/min |
2
|
Yieldb (%) |
Reaction conditions: 5 mol% of [Pd(C3H5)Cl]2, 11 mol% of L6, 0.2 mmol of 1, and 100 mol% of KOAc in THF (2 mL) at 50 °C.
Isolated yield of 2.
|
1 |
1a, NBn, H, H |
60 |
2a
|
70 |
2 |
1b, N-allyl, H, H |
40 |
2b
|
40 |
3 |
1c, NBn, Me, H |
30 |
2c
|
68 |
4 |
1d, NBn, F, H |
30 |
2d
|
57 |
5 |
1e, NBn, Cl, H |
30 |
2e
|
60 |
6 |
1f, NBn, H, Ph |
30 |
2f
|
64 |
7 |
1g, NBn, H, p-MeC6H4 |
30 |
2g
|
68 |
8 |
1h, NBn, H, p-ClC6H4 |
30 |
2h
|
68 |
9 |
1i, NBn, H, Me |
30 |
2i
|
40 |
10 |
1j, NBn, H, allyl |
30 |
2j
|
50 |
11 |
1k, C(CO2Me)2, H, H |
30 |
2k
|
70 |
Iridium-catalyzed Friedel–Crafts type allylic alkylation reaction of indole fused through C4–C3
In order to test the hypothesis for the seven-membered ring formation, we next explored the reaction under Ir-catalyzed allylic substitution reaction conditions.18 With the Ir-complex derived from [Ir(cod)Cl]2 (4 mol%) and ligands L7–10 (8 mol%) as the catalyst (Fig. 2), the reaction conditions were optimized for the enantioselective Friedel–Crafts type allylic alkylation of 1a, forming a seven-membered ring fused through C4–C3 (see ESI† for details).
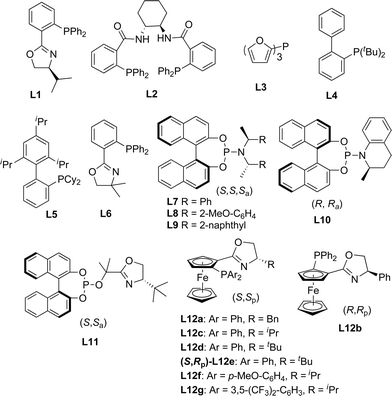 |
| Fig. 2 Selected ligands used in the present work. | |
After considerable optimization studies, the following conditions were found to be optimal: 4 mol% of [Ir(cod)Cl]2, 8 mol% of L7, 100 mol% of Cs2CO3, 0.04 mol L−1, DCM, reflux. Then various substrates were tested to examine the scope of the reaction. The results are summarized in Table 3. As to substituents on the nitrogen, both benzyl and allyl groups could be tolerated delivering alkylated products in excellent enantioselectivity (94% ee) (entries 1 and 2, Table 3). Reaction of 7-substituted indole substrates generated the products with good yields and high enantioselectivity (entries 3–5, Table 3). Substrates bearing either an aryl or aliphatic group at the 2-position of indole could be used in the reaction. With 2-aryl indole substrates, the products were obtained in good yields and high enantioselectivity (entries 6–8, Table 3). With 2-aliphatic group substituted indole substrates, high enantioselectivity could be obtained, but in moderate yields (entries 9 and 10, Table 3). Unfortunately, the carbon tethered substrate 1k could not be applied in the reaction (entry 11, Table 3).
Table 3 Substrate scope for Ir-catalyzed enantioselective Friedel–Crafts type allylic alkylation of indole fused through C4–C3a
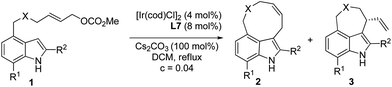
|
Entry |
1, X, R1, R2 |
2/3b |
Yieldc (%) |
eed (%) |
Reaction conditions: 4 mol% of [Ir(cod)Cl]2, 8 mol% of L7, 0.2 mmol of 1, and 100 mol% Cs2CO3 in DCM (5 mL) under reflux.
Determined by 1H NMR of the crude reaction mixture.
Isolated yield of 3.
Determined by HPLC analysis.
|
1 |
1a, NBn, H, H |
2/98 |
3a: 60 |
94 |
2 |
1b, N-allyl, H, H |
1/99 |
3b: 51 |
94 |
3 |
1c, NBn, Me, H |
1/99 |
3c: 46 |
94 |
4 |
1d, NBn, F, H |
4/96 |
3d: 64 |
93 |
5 |
1e, NBn, Cl, H |
6/94 |
3e: 62 |
93 |
6 |
1f, NBn, H, Ph |
2/98 |
3f: 78 |
94 |
7 |
1g, NBn, H, p-MeC6H4 |
1/99 |
3g: 76 |
91 |
8 |
1h, NBn, H, p-ClC6H4 |
2/98 |
3h: 68 |
91 |
9 |
1i, NBn, H, Me |
1/99 |
3i: 40 |
95 |
10 |
1j, NBn, H, allyl |
1/99 |
3j: 60 |
97 |
11 |
1k, C(CO2Me)2, H, H |
— |
NR |
— |
According to the general rule of stereochemistry in the Ir-catalyzed allylic substitution reactions,14,19 the absolute configuration of the reaction product 3 was proposed to be (S).
Palladium-catalyzed allylic dearomatization of indole fused through C4–C3
Recently, 3-subsituted indoles were shown to be compatible in asymmetric allylic dearomatization reaction in an either intramolecular or intermolecular version.20 Encouraged by the Pd-catalyzed intramolecular allylic alkylation of indole fused through C4–C3, we then explored the allylic dearomatization reaction by installing a substituent at the C-3 position of indole. Notably, the reaction would provide interesting fused ring structures bearing a stereogenic quaternary carbon center. When 3-allyl substituted indole substrate was used in the above palladium-catalyzed allylic alkylation reaction, indeed compound 5a, a dearomatization product of indole, could be obtained. We further carried out the optimization of reaction condition (see ESI† for details). After screening various Phox type ligands (Fig. 2), the reaction with planar chiral ferrocene-based ligand (S,Sp)-L12c led to product 5a in 74% yield and 78% ee (Scheme 3).
![Substrate scope for Pd-catalyzed allylic dearomatization reaction fused through C4–C3. Reaction conditions: 5 mol% of [Pd(C3H5)Cl]2, 11 mol% of (S,Sp)-L12c, 0.2 mmol of 4, and 100 mol% KOAc in THF (2 mL) at 50 °C.](/image/article/2013/SC/c2sc21085a/c2sc21085a-s3.gif) |
| Scheme 3 Substrate scope for Pd-catalyzed allylic dearomatization reaction fused through C4–C3. Reaction conditions: 5 mol% of [Pd(C3H5)Cl]2, 11 mol% of (S,Sp)-L12c, 0.2 mmol of 4, and 100 mol% KOAc in THF (2 mL) at 50 °C. | |
Then, various substrates were tested under these optimized conditions to examine the substrate scope of the Pd-catalyzed allylic dearomatization reaction fused through C4–C3. The results are summarized in Scheme 3. As to substituents on the nitrogen, both benzyl and allyl groups could be tolerated delivering alkylated products in good enantioselectivity (76–78% ee, 5a and 5b, Scheme 3). Substrates bearing either methyl, allylic or benzyl group at the 3-position of indole could be used in the reaction. With 3-benzyl indole substrates, the products were obtained in good yields and moderate enantioselectivity (5d–5g, Scheme 3). Substrates bearing a nucleophilic side chain at the 3-position of indole could be applied in the reaction, and a cascade reaction proceeded to afford polycyclic products in good yields and moderate enantioselectivity (5h–5j, Scheme 3). The carbon tethered substrate 4l could also be used in the reaction, affording the product with 78% yield and 42% ee (5l, Scheme 3).
When substrate 4m (Scheme 4) was used, a macrocyclic product (13-membered ring) was obtained in 82% yield under the optimized conditions (eqn (1)). Treatment of 5g with 20 mol% of TsOH·H2O led to Friedel–Crafts product 5n in 60% yield (eqn (2)). When treated with excess TFA, product 5k underwent deprotection of the Boc group and then addition to imine, affording compound 5o in 80% yield and 73% ee (eqn (3)).
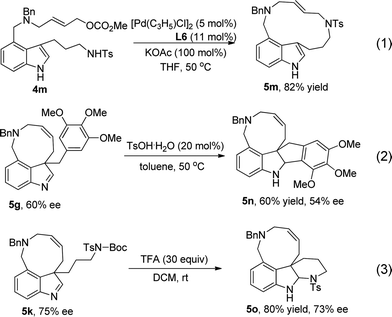 |
| Scheme 4 The reaction of 4m and product transformation. | |
Iridium-catalyzed Friedel–Crafts type allylic alkylation reaction of indole fused through C4–C5
Since Ir-catalysts display different tendency towards the regioselectivity in allylic substitution reactions, we then explored substrates 4 in Ir-catalyzed allylic substitution reactions. Initially, an allylic dearomatization product bearing two chiral centers was expected as a result of reaction at the C3 position of indole. However, to our surprise, when substrate 4a was used in the Ir-catalyzed allylic alkylation reaction conditions, Friedel–Crafts type allylic alkylation product 6a was obtained without observation of the formation of dearomatization product by reaction at the C3 position of indole.
After extensive screening of the reaction conditions (see ESI† for details), the Ir-complex derived from [Ir(dbcot)Cl]2 (ref. 21) and ligand L8 was found an optimal catalyst [optimized reaction conditions: 4 mol% of [Ir(dbcot)Cl]2, 8 mol% of L8, 200 mol% of Cs2CO3, toluene, 50 °C].
Under the optimized reaction conditions, various substrates were tested to examine the scope of the reaction, as shown in Scheme 5. As to substituents on the nitrogen, both benzyl and allyl groups could be tolerated delivering alkylated products with good enantioselectivity (83–90% ee). Substrates bearing either methyl, allyl or benzyl group at the 3-position of indole could be used in the reaction. With 3-benzyl indole substrates, the products were obtained with good yields and enantioselectivity (80–86% ee, Scheme 5). Substrates having an aryl group at the 3-position of indole were also compatible in the reaction. Varying the electronic and substituent pattern on the benzyl group at the C3 position of indole displayed no notable effect on the reaction outcome, and products were obtained with good yields and moderate enantioselectivity (6r–6t, Scheme 5). Notably, for substrate 4t, the dearomatization product occurring at the C3 position was also observed with 2.7/1 dr.
![Substrate scope for Ir-catalyzed Friedel–Crafts type allylic alkylation fused through C4–C5. Reaction conditions: 4 mol% of [Ir(dbcot)Cl]2, 8 mol% of L8, 0.2 mmol of 4, and 200 mol% Cs2CO3 in toluene (2 mL) at 50 °C.](/image/article/2013/SC/c2sc21085a/c2sc21085a-s5.gif) |
| Scheme 5 Substrate scope for Ir-catalyzed Friedel–Crafts type allylic alkylation fused through C4–C5. Reaction conditions: 4 mol% of [Ir(dbcot)Cl]2, 8 mol% of L8, 0.2 mmol of 4, and 200 mol% Cs2CO3 in toluene (2 mL) at 50 °C. | |
Discussions on the reaction pathway
For unsymmetrical allylic substrates, palladium catalysts tend to give the linear products mainly due to steric effects, and nucleophilic attack towards the less substituted π-allyl terminus is therefore dominating. This explains the nine-membered ring formation when substrate 1a is subjected to palladium catalysis. For substrate 4a bearing an allyl group at the C3 position of indole, a dearomative product is given. With Ir-catalysts, the electronic effect is dominant in controlling the regioselectivity. The positive charge can be stabilized by the substituent at the more substituted π-allyl terminus, and then the latter is favored for the nucleophilic attack. Consequently, substrate 1a under Ir-catalyst leads to the seven-membered ring product. However, when substrate 4a is utilized, the branch selective attack on the π-allyl intermediate from the C3 position of indole will generate two adjacent chiral centers including one quaternary carbon center, which is highly unfavorable in terms of the steric hindrance. As a result, the electron-rich nature of the C5 carbon of indole enables the enantioselective C4–C5 intramolecular Friedel–Crafts type allylic alkylation reaction of indole.
In summary, indole-based peri-annulated structures fused through C4–C3 were synthesized via Pd- or Ir-catalyzed intramolecular Friedel–Crafts type allylic alkylation reaction. With palladium catalyst, the indole-based nine-membered ring products were obtained in moderate to good yields (40–70%). When chiral iridium catalyst was used, highly enantioenriched seven-membered ring products were obtained in 40–78% yields and 91–97% ee. Meanwhile, when 3-substituted indoles were employed with a chiral palladium catalyst, allylic dearomatization of indole products were obtained in 48–78% yields and 35–78% ee. With an iridium catalyst, Friedel–Crafts type allylic alkylation reaction fused through C4 to C5 were obtained in 40–60% yields and 56–97% ee. These studies provide a diverse synthesis of indole pericycles starting from readily available substrates. Meanwhile, Friedel–Crafts type allylic alkylation reaction fused through C4 to C5 was reported for the first time. The direct enantioselective Friedel–Crafts alkylation of indole at the C5 position and asymmetric allylic dearomatization reaction of indole reported herein will likely inspire further studies in this area.
Acknowledgements
We thank the National Basic Research Program of China (973 Program 2009CB825300) and National Natural Science Foundation of China (20923005, 20932008, 21025209, 21121062) for generous financial support.
Notes and references
- Selected examples:
(a) B. L. Gray and S. L. Schreiber, J. Comb. Chem., 2007, 9, 1028 CrossRef CAS;
(b) J.-F. Brazeau, S. Zhang, I. Colomer, B. K. Corkey and F. D. Toste, J. Am. Chem. Soc., 2012, 134, 2742 CrossRef CAS.
- D. J. Faulkner, Nat. Prod. Rep., 2001, 18, 1 RSC , and references cited therein.
- P. Sauleau, M. T. Martin, M. E. T. H. Dau, D. T. A. Youssef and M. L. Bourguet-Kondracki, J. Nat. Prod., 2006, 69, 1676 CrossRef CAS.
- A. G. Kozolovskii, T. F. Solovéva, V. G. Sahkarovskii and V. D. Adanin, Dokl. Akad. Nauk SSSR, 1981, 260, 230 Search PubMed.
-
(a) J. E. Robbers and H. G. Floss, Tetrahedron Lett., 1969, 10, 1857 CrossRef;
(b) G. S. King, E. S. Waight, P. G. Mantle and C. A. Szczyrbak, J. Chem. Soc., Perkin Trans. 1, 1977, 2099 RSC;
(c) J. E. Robbers, H. Otsuka and H. G. Floss, J. Org. Chem., 1980, 45, 1117 CrossRef CAS.
-
(a) J. B. Hendrickson and J. Wang, Org. Lett., 2004, 6, 3 CrossRef CAS;
(b) E. Fillion and A. M. Dumas, J. Org. Chem., 2008, 73, 2920 CrossRef CAS.
-
(a) K. Yamada, Y. Namerikawa, T. Haruyama, Y. Miwa, R. Yanada and M. Ishikura, Eur. J. Org. Chem., 2009, 5752 CrossRef CAS;
(b) K. Yamada, Y. Namerikawa, T. Abe and M. Ishikura, Heterocycles, 2009, 77, 825 CrossRef CAS;
(c) M. Saifuddin, P. K. Agarwal, S. K. Sharma, A. K. Mandadapu, S. Gupta, V. K. Harit and B. Kundu, Eur. J. Org. Chem., 2010, 5108 CrossRef CAS;
(d) K. Yamada, S. Yamaguchi, N. Hatae, T. Abe, T. Iwamura and M. Ishikura, Heterocycles, 2011, 83, 815 CrossRef CAS;
(e) D.-J. Cheng, H.-B. Wu and S.-K. Tian, Org. Lett., 2011, 13, 5636 CrossRef CAS;
(f) H. Schönherr and J. L. Leighton, Org. Lett., 2012, 14, 2610 CrossRef.
-
(a) S. Katayama, N. Ae and R. Nagata, J. Org. Chem., 2001, 66, 3474 CrossRef CAS;
(b) H. J. Lim, J. C. Gallucci and T. V. RajanBabu, Org. Lett., 2010, 12, 2162 CrossRef CAS.
-
(a) A. V. Samet, A. N. Yamskov, Y. A. Strelenko and V. V. Semenov, Tetrahedron, 2009, 65, 6868 CrossRef CAS;
(b) S. L. Crawley and R. L. Funk, Org. Lett., 2003, 5, 3169 CrossRef CAS.
- Selected examples for transition-metal-catalyzed allylic substitution reactions with indoles:
(a) M. Bandini, A. Melloni and A. Umani-Ronchi, Org. Lett., 2004, 6, 3199 CrossRef CAS;
(b) M. Kimura, M. Futamata, R. Mukai and Y. Tamaru, J. Am. Chem. Soc., 2005, 127, 4592 CrossRef CAS;
(c) M. Bandini, A. Melloni, F. Piccinelli, R. Sinisi, S. Tommasi and A. Umani-Ronchi, J. Am. Chem. Soc., 2006, 128, 1424 CrossRef CAS;
(d) H. Y. Cheung, W.-Y. Yu, F. L. Lam, T. T.-L. Au-Yeung, Z. Zhou, T. H. Chan and A. S. C. Chan, Org. Lett., 2007, 9, 4295 CrossRef CAS;
(e) W.-B. Liu, H. He, L.-X. Dai and S.-L. You, Org. Lett., 2008, 10, 1815 CrossRef CAS;
(f) T. Hoshi, K. Sasaki, S. Sato, Y. Ishii, T. Suzuki and H. Hagiwara, Org. Lett., 2011, 13, 932 CrossRef CAS;
(g) Z. Cao, Y. Liu, Z. Liu, X. Feng, M. Zhuang and H. Du, Org. Lett., 2011, 13, 2164 CrossRef CAS;
(h) B. Sundararaju, M. Achard, B. Demerseman, L. Toupet, G. V. M. Sharma and C. Bruneau, Angew. Chem., Int. Ed., 2010, 49, 2782 CrossRef CAS.
-
(a) B. M. Trost and D. L. Van Vranken, Chem. Rev., 1996, 96, 395 CrossRef CAS;
(b) B. M. Trost and M. L. Crawley, Chem. Rev., 2003, 103, 2921 CrossRef CAS;
(c) Z. Lu and S. Ma, Angew. Chem., Int. Ed., 2008, 47, 258 CrossRef CAS;
(d) B. M. Trost, T. Zhang and J. D. Sieber, Chem. Sci., 2010, 1, 427 RSC.
- Branched selectivity in Pd-catalyzed allylic reactions with directing groups:
(a) B. M. Trost and F. D. Toste, J. Am. Chem. Soc., 1999, 121, 4545 CrossRef CAS;
(b) B. M. Trost, R. C. Bunt, R. C. Lemoine and T. L. Calkins, J. Am. Chem. Soc., 2000, 122, 5968 CrossRef CAS;
(c) M. E. Krafft, M. Sugiura and K. A. Abboud, J. Am. Chem. Soc., 2001, 123, 9174 CrossRef CAS;
(d) G. R. Cook, H. Yu, S. Sankaranarayanan and P. S. Shanker, J. Am. Chem. Soc., 2003, 125, 5115 CrossRef CAS;
(e) S. Sebelius, V. J. Olsson, O. A. Wallner and K. J. Szabo, J. Am. Chem. Soc., 2006, 128, 8150 CrossRef CAS. With special ligands:
(f) R. Prétôt and A. Pfaltz, Angew. Chem., Int. Ed., 1998, 37, 323 CrossRef;
(g) T. Hayashi, M. Kawatsura and Y. Uozumi, J. Am. Chem. Soc., 1998, 120, 1681 CrossRef CAS;
(h) S.-L. You, X.-Z. Zhu, Y.-M. Luo, X.-L. Hou and L.-X. Dai, J. Am. Chem. Soc., 2001, 123, 7471 CrossRef CAS;
(i) W.-H. Zheng, B.-H. Zheng, Y. Zhang and X.-L. Hou, J. Am. Chem. Soc., 2007, 129, 7718 CrossRef CAS;
(j) J.-P. Chen, C.-H. Ding, W. Liu, X.-L. Hou and L.-X. Dai, J. Am. Chem. Soc., 2010, 132, 15493 CrossRef CAS.
- For reviews:
(a) R. Takeuchi and S. Kezuka, Synthesis, 2006, 3349 CrossRef CAS;
(b) G. Helmchen, A. Dahnz, P. Dübon, M. Schelwies and R. Weihofen, Chem. Commun., 2007, 675 RSC;
(c)
G. Helmchen, in Iridium Complexes in Organic Synthesis, ed. L. A. Oro and C. Claver, Wiley-VCH, Weinheim, 2009, pp. 211–250 Search PubMed;
(d) J. F. Hartwig and L. M. Stanley, Acc. Chem. Res., 2010, 43, 1461 CrossRef CAS;
(e) J. F. Hartwig and M. J. Pouy, Top. Organomet. Chem., 2011, 34, 169 CrossRef CAS;
(f) W.-B. Liu, J.-B. Xia and S.-L. You, Top. Organomet. Chem., 2012, 38, 155 CrossRef.
-
(a) J. Tsuji, I. Minami and I. Shimizu, Tetrahedron Lett., 1984, 25, 5157 CrossRef CAS;
(b) T. Hayashi, A. Okada, T. Suzuka and M. Kawatsura, Org. Lett., 2003, 5, 1713 CrossRef CAS;
(c) R. Takeuchi and N. Kitamura, New J. Chem., 1998, 22, 659 RSC;
(d) P. A. Evans and J. D. Nelson, Tetrahedron Lett., 1998, 39, 1725 CrossRef CAS.
-
(a) B. M. Trost and M. Lautens, J. Am. Chem. Soc., 1982, 104, 5543 CrossRef CAS;
(b) B. M. Trost, K. Dogra, I. Hachiya, T. Emura, D. L. Hughes, S. W. Krska, R. A. Reamer, M. Palucki, N. Yasuda and P. J. Reider, Angew. Chem., Int. Ed., 2002, 41, 1929 CrossRef CAS.
- B. M. Trost and M.-H. Hung, J. Am. Chem. Soc., 1983, 105, 7757 CrossRef CAS.
-
(a) H. Ono, N. Satake and Y. Watanabe, Organometallics, 1995, 14, 1945 CrossRef;
(b) Y. Morisaki, T. Kondo and T. Mitsudo, Organometallics, 1999, 18, 4742 CrossRef CAS;
(c) B. M. Trost, P. Fraisse and Z. Ball, Angew. Chem., Int. Ed., 2002, 41, 1059 CrossRef CAS.
-
(a) C. A. Kiener, C. Shu, C. Incarvito and J. F. Hartwig, J. Am. Chem. Soc., 2003, 125, 14272 CrossRef CAS;
(b) S. T. Madrahimov, D. Markovic and J. F. Hartwig, J. Am. Chem. Soc., 2009, 131, 7228 CrossRef CAS;
(c) S. Spiess, J. A. Raskatov, C. Gnamm, K. Brödner and G. Helmchen, Chem.–Eur. J., 2009, 15, 11087 CrossRef CAS;
(d) S. T. Madrahimov and J. F. Hartwig, J. Am. Chem. Soc., 2012, 134, 8136 CrossRef CAS.
-
(a) H. He, X.-J. Zheng, Y. Li, L.-X. Dai and S.-L. You, Org. Lett., 2007, 9, 4339 CrossRef CAS;
(b) W.-B. Liu, S.-C. Zheng, H. He, X.-M. Zhao, L.-X. Dai and S.-L. You, Chem. Commun., 2009, 6604 RSC;
(c) J.-B. Xia, W.-B. Liu, T.-M. Wang and S.-L. You, Chem.–Eur. J., 2010, 16, 6442 CAS;
(d) Q.-L. Xu, W.-B. Liu, L.-X. Dai and S.-L. You, J. Org. Chem., 2010, 75, 4615 CrossRef CAS;
(e) W.-B. Liu, X. Zhang, L.-X. Dai and S.-L. You, Angew. Chem., Int. Ed., 2012, 51, 5183 CrossRef CAS.
- For asymmetric allylic dearomatization of indoles, see:
(a) B. M. Trost and J. Quancard, J. Am. Chem. Soc., 2006, 128, 6314 CrossRef CAS;
(b) Q.-F. Wu, H. He, W.-B. Liu and S.-L. You, J. Am. Chem. Soc., 2010, 132, 11418 CrossRef CAS;
(c) Q.-F. Wu, W.-B. Liu, C.-X. Zhuo, Z.-Q. Rong, K.-Y. Ye and S.-L. You, Angew. Chem., Int. Ed., 2011, 50, 4455 CrossRef CAS.
- For early examples employing [Ir(dbcot)Cl]2 in allylic substitution reactions:
(a) S. Spiess, C. Welter, G. Franck, J.-P. Taquet and G. Helmchen, Angew. Chem., Int. Ed., 2008, 47, 7652 CrossRef CAS;
(b) M. Gärtner, S. Mader, K. Seehafer and G. Helmchen, J. Am. Chem. Soc., 2011, 133, 2072 CrossRef;
(c) K.-Y. Ye, H. He, W.-B. Liu, L.-X. Dai, G. Helmchen and S. L. You, J. Am. Chem. Soc., 2011, 133, 19006 CrossRef CAS.
Footnote |
† Electronic supplementary information (ESI) available: Experimental procedures and analysis data for new compounds. See DOI: 10.1039/c2sc21085a |
|
This journal is © The Royal Society of Chemistry 2013 |
Click here to see how this site uses Cookies. View our privacy policy here.