DOI:
10.1039/C2SC21485G
(Edge Article)
Chem. Sci., 2013,
4, 460-467
A self-referenced nanodosimeter for reaction based ratiometric imaging of hypochlorous acid in living cells†
Received 6th August 2012, Accepted 26th September 2012
First published on 3rd October 2012
Abstract
Hypochlorous acid (HOCl) is biosynthesized from hydrogen peroxide via catalysis of myeloperoxidase in lysosomes of immunological cells. Despite being harnessed by immune systems against invading pathogens, biogenic HOCl can also damage host tissues and has been associated with a number of diseases. In this edge article, Förster resonance energy transfer based ratiometric imaging of lysosomal HOCl was achieved with silica nanoparticles comprising FITC (donor dye) and a nonfluorescent chemodosimeter which turned into rhodamine (acceptor dye) upon HOCl triggered tandem oxidation and β-elimination of the doped chemodosimeter. The nanodosimeter exhibited distinct biochemical properties relative to small molecule-based free chemodosimeters, e.g. lysosome-homing specificity and compatibility with aqueous media, enabling facile monitoring of lysosomal HOCl by conventional flow cytometry. The nanoprobe would be of broad utility for studies on in vivo generation and the impact of lysosomal HOCl in living cells or even in animals.
Introduction
Hypochlorous acid (HOCl) is a natural oxidant that can be synthesized from hydrogen peroxide and chloride with the aid of myeloperoxidase in a number of immunological cells.1 Despite being harnessed by immune systems against microorganism infections, in vivo generated HOCl can also be detrimental and has been implicated in multiple diseases such as rheumatoid arthritis and neuron degeneration.2 Chemosensors that allow imaging or quantitation of HOCl will be valuable for investigations of HOCl related biological processes. In line with this, a number of imaging agents that become emissive upon analyte triggered oxidation have been developed by integration of fluorophores with HOCl-responsive functionalities.3 Single-intensity based probes are often hindered in quantitative assays due to many uncertainties, e.g. uneven distribution of imaging agents in biological specimens. Ratiometric probes that can normalize these interferences have been largely unexplored for HOCl.4Lysosomes, hallmarked by acidic intracompartmental pH (6.0–4.5), are the major degradation machinery for internalized microorganisms. Given the role of HOCl in inactivation of microorganisms in host defense and the fact that myeloperoxidase is a lysosomal enzyme dedicated to the biogenesis of HOCl, probes that can selectively report levels of lysosomal HOCl will be of tremendous interest for real time studies of the in vivo generation and impact of HOCl. With a pKa of 7.46, HOCl readily dissociates into hypochlorite (OCl−) in cytosol. Small molecule based probes are cell membrane permeable and diffuse into cells. Due to their lack of capability in targeting lysosomes, existing chemosensors mostly respond to cytosolic hypochlorite inside cells. Here we report Förster resonance energy transfer (FRET) based ratiometric imaging of lysosomal HOCl with mesoporous silica nanoparticles (MSN) functionalized with FITC, which serves as the donor dye, and a highly selective rhodamine-derived chemodosimeter which turns into a highly fluorescent rhodamine acceptor upon analyte triggered oxidative opening of the intramolecular thioether (Fig. 1).
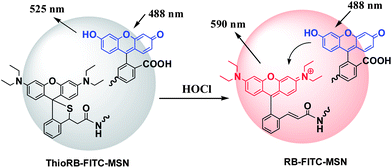 |
| Fig. 1 FRET based ratiometric imaging of HOCl with ThioRB-FITC-MSN via analyte triggered oxidative opening of the intramolecular spirothioether to give acceptor fluorophore. | |
Experimental
Materials and methods
LysoTracker Blue DND-22 was purchased from Invitrogen. Methoxy-PEG-succinimidyl propionate was obtained from Jiaxing Biomatrix Inc. The aqueous solution of sodium hypochlorite (NaOCl) and other chemicals were obtained from Alfa Aesar. Column chromatography was performed on silica gel (100–200 mesh). NMR spectra (1H at 400 MHz and 13C at 100 MHz) were recorded on a Bruker instrument using tetramethylsilane as the internal reference. Mass analysis was performed in a Bruker En Apex ultra 7.0T FT-MS. Fluorescence spectra and UV-vis absorption spectra were recorded on a spectrofluorometer (SpectraMax M5, Molecular Device). L929 cells and HeLa cells were obtained from the American Type Culture Collection (ATCC). Confocal fluorescence microscopy images were obtained on a Leica SP5 using the following filters: λex@543 nm and λem@565–625 nm for rhodamine B signal; λex@488 nm and λem@500–530 nm for FITC signal. The fluorescence of LysoTracker Blue and that of fluorescein inside cells were merged by using Photoshop CS 5.0. Flow cytometric data were obtained on a Beckman Coulter. The fluorescence emission intensity of FITC was recorded with filter FL1 (510–535 nm) while that of ThioRB was recorded with filter FL2 (565–625 nm). 10
000 cells were analyzed and the data were processed using Origin 8.5.Synthesis of compound 1
To a solution of rhodamine B (10 g) in anhydrous tetrahydrofuran (50 ml) was added lithium aluminium hydride (4 g) in portions. The mixture was stirred at rt for 48 h and then quenched with the dropwise addition of ethyl acetate (50 ml). The mixture was poured into water (200 ml) and then extracted with ethyl acetate (200 ml). The organic layer was collected, dried over sodium sulfate, and then concentrated by rotary evaporation. The residue was purified by silica gel chromatography using ethyl acetate–hexanes–triethylamine (10
:
2
:
1) as the eluent to afford the desired product (6.5 g, 65%). 1H-NMR (400 MHz, CDCl3): δ 7.45 (m, 1H), 7.29 (m, 3H), 6.71 (dd, 2H, J1 = 0.64 Hz, J2 = 0.64 Hz), 6.42 (m, 2H), 6.30 (m, 2H), 4.59 (s, 2H), 3.35 (q, 8H, J = 7.08 Hz), 1.18 (t, 12H, J = 7.04 Hz); 13C-NMR (100 MHz, CDCl3): 151.67, 147.77, 138.66, 131.38, 129.96, 129.61, 129.47, 127.96, 126.89, 124.24, 120.45, 111.43, 107.85, 107.42, 98.77, 97.78, 62.94, 46.18, 44.39, 12.64, 11.46.Synthesis of compound 2
To a solution of compound 1 (5 g) in dichloromethane (30 ml) was slowly added PCC (5 g). The mixture was stirred at rt for 20 min, poured into water (150 ml) and then extracted with dichloromethane (200 ml). The organic layer was collected, dried over sodium sulfate, and then concentrated by rotary evaporation. The residue was purified by silica gel chromatography using ethyl acetate–hexanes–triethylamine (10
:
2
:
1) as the eluent to afford compound 2 as the desired product (4 g, 80%). 1H-NMR (400 MHz, CDCl3): δ 7.55 (d, 1H), 7.40 (m, 2H), 6.94 (dd, 2H, J1 = 6.0 Hz, J2 = 8.4 Hz), 6.65 (m, 2H), 6.41 (m, 4H), 3.37 (q, 8H, J = 6.92 Hz), 1.19 (t, 12H, J = 7.02 Hz); 13C-NMR (100 MHz, CDCl3): 152.66, 152.33, 148.66, 130.50, 130.06, 129.27, 128.18, 123.95, 122.31, 111.68, 108.00, 107.90, 99.34, 97.66, 97.50, 44.50, 12.71; HRMS (C28H31N2O2+) calculated (M+): 427.2380, found: 427.2359.Synthesis of RB-CM
(Methoxycarbonylmethylene)triphenylphosphorane (4 g) was added to a flask containing methanol (20 ml) and compound 2 (4 g). The mixture was stirred at rt for 12 h. The solution was concentrated by rotary evaporation to remove the solvent, and the residue was purified by silica gel chromatography using dichloromethane–triethylamine (10
:
1) as the eluent to afford RB-CM in 85% yield. 1H-NMR (400 MHz, CD3OD): δ 8.34 (dd, 1H, J1 = 1.04 Hz, J2 = 1.16 Hz), 7.81 (m, 3H), 7.56 (m, 3H), 7.06 (m, 4H), 5.51 (s, 1H), 3.68 (q, 8H, J = 7.12 Hz), 3.21 (q, 3H, J = 7.32 Hz), 1.31 (t, 12H, J = 4.16 Hz); 13C-NMR (100 MHz, CD3OD): 165.56, 158.90, 157.90, 155.71, 133.81, 132.77, 132.46, 130.90, 130.85, 130.26, 130.13, 129.99, 127.61, 125.78, 114.04, 113.34, 95.86, 51.56, 46.30, 45.44, 11.43, 7.80; HRMS (C31H35N2O3+) calculated (M+): 483.2642, found: 483.2646.Synthesis of ThioRB-ester
Sodium hydrosulfide (0.2 g) was added to a flask containing DMF (10 ml) and RB-CM (1 g). The mixture was stirred at rt for 10 min. The solution was poured into water (200 ml) and then extracted using dichloromethane/water (200 ml). The organic layer was collected, dried over sodium sulfate and then evaporated in vacuo. The residue was purified by silica gel chromatography using hexanes–triethylamine (10
:
1) as the eluent to afford ThioRB-ester as the desired product in 55% yield. 1H-NMR (400 MHz, DMSO): δ 7.49 (d, 1H, J1 = 7.68 Hz), 7.32 (t, 1H, J = 7.68 Hz), 7.22 (t, 1H, J = 7.52 Hz), 6.71 (m, 3H), 6.36 (m, 2H), 6.23 (m, 2H), 5.22 (dd, 1H, J1 = 3.36 Hz, J2 = 3.44 Hz), 3.64 (s, 1H), 3.35 (s, 3H), 3.30 (q, 8H, J = 2.28 Hz), 1.08 (t, 12H, J = 3.80 Hz); 13C-NMR (100 MHz, DMSO): 171.94, 151.32, 150.79, 149.88, 147.82, 147.73, 141.85, 131.89, 131.15, 128.80, 127.81, 127.05, 124.54, 114.95, 114.11, 108.54, 96.97, 62.20, 52.04, 48.60, 44.15, 44.05, 12.92; HRMS (C31H36N2O3S): calculated (M + H+): 517.2519, found: 517.2521.Preparation of ThioRB-FITC-MSN
Synthesis of ThioRB-APTS: ThioRB-ester (0.1 g) was added to (3-aminopropyl)triethoxysilane (APTS) (2 ml) in a capped vial. The mixture was stirred at rt in the dark for 7 days. The resultant product, ThioRB-APTS, was directly used for the preparation of mesoporous silica nanoparticles.Synthesis of FITC-APTS: FITC (50 mg) was added to DMF (500 μl) containing APTS (300 μl) in a capped vial. The mixture was stirred at rt in the dark for 2 h. The resultant product, FITC-APTS, was directly used for the preparation of mesoporous silica nanoparticles.
Preparation of ThioRB-FITC-MSN: for MSNs assayed in Na2HPO4–citrate buffer (100 mM, pH 5), the as-prepared solutions of ThioRB-APTS (100 μl) and FITC-APTS (100 μl) and tetraethyl orthosilicate (TEOS, 2.3 ml) were added to a clean flask containing deionized water (240 ml), cetyltrimethylammonium bromide (CTAB) (0.5 g) and 1.75 ml of aqueous NaOH (2 M) at 80 °C. The mixture was stirred at 80 °C for 1 h and then centrifuged at 10
000 rpm for 10 min to collect the silica MSNs. The MSNs were further treated with methanol containing HCl (5%) under reflux for 3 h. The sample was centrifuged and the pellet was further subjected to repeated resuspension in methanol by ultrasonication, centrifugation and decantation to remove the unreacted chemicals and CTAB.
For MSNs assayed in PBS (pH 7.4), the nanoparticles were prepared using the same procedure as described above with the exception that a lower volume of FITC-APTS (30 μl) was utilized in co-condensation with ThioRB-APTS (100 μl) and tetraethyl orthosilicate (2.3 ml).
Surface modification of MSNs with poly(ethylene glycol)
The as-prepared MSNs (200 mg) were added to dimethylformamide (20 ml) containing methoxy-PEG-succinimidyl propionate (MW 5000, 0.145 g) and triethylamine (200 μl). The mixture was sonicated for 90 min followed by addition of saturated NaHCO3 solution (30 ml). The mixture was further sonicated for 90 min, and then centrifuged. The nanoparticles were collected, extensively washed with deionized water to remove any residual chemicals, and then stored in water (50 mg ml−1) for subsequent cell assays. The nanoparticles before and after pegylation were analyzed by transmission electron microscopy (TEM), scanning electron microscopy (SEM) and dynamic light scattering.Kinetic profiles on the reaction of ThioRB-FITC-MSN with HOCl
Aqueous NaOCl solution was respectively added into PBS (pH 7.4) containing ThioRB-FITC-MSN (1 mg ml−1) to make a series of assay solutions containing NaOCl (0–100 μM). The rates of fluorescence development in the reaction solutions were recorded on a SpectraMax M5 using λex@560 nm and λem@586.pH titration of ThioRB-FITC-MSN
An aliquot of ThioRB-FITC-MSN stock solution in water was added to Na2HPO4–H3PO4 buffer (200 mM) of various pH values (8.0, 7.5, 7.0, 6.5, 6.0, 5.5, 5.0, or 4.5) to a final concentration of 1 mg ml−1. The fluorescence emission at 586 nm was recorded as a function of pH using λex@560 nm for activated ThioRB doped in MSN.Effects of pH on HOCl dependent activation of ThioRB-FITC-MSN
An aliquot of NaOCl solution was added to a series of Na2HPO4–H3PO4 buffers of different pH values (8.0, 7.5, 7.0, 6.5, 6.0, 5.5, 5.0, or 4.5) containing ThioRB-FITC-MSN (1 mg ml−1) to a final concentration of 100 μM. The fluorescence emission intensity at 586 nm was recorded as a function of pH using λex@560 nm for ThioRB doped in MSN.Effects of biomacromolecules on activation of ThioRB-FITC-MSN by NaOCl
NaOCl solution was added to DMEM culture medium spiked with or without ThioRB-FITC-MSN (1 mg ml−1) to a final concentration of 500 μM. The samples were mixed and then aqueous HCl (1 M, 10 μl) was added to both samples. Visual images of the samples were recorded using a digital camera. In parallel, NaOCl solution was added to PBS spiked with or without ThioRB-FITC-MSN (1 mg ml−1) to a final concentration of 500 μM. The samples were mixed and then recorded using a digital camera for visual images.Effects of media on activation of ThioRB-ester by NaOCl
An aliquot of ThioRB-ester stock solution in dimethylsulfoxide was respectively added to PBS, acetonitrile, or PBS buffered acetonitrile (25%, 50%, and 75% v/v) to a final concentration of 10 μM. NaOCl was spiked in the samples to a final concentration of 100 μM. The samples were mixed and then recorded using a digital camera for visual images of the samples. Control experiments were carried out under identical conditions without the addition of NaOCl.Characterization of ThioRB-ester for HOCl with high resolution mass spectrometry
To aqueous acetonitrile (50%, v/v) containing ThioRB-ester (100 mg) was added NaOCl solution (100 μl). The mixture was directly analyzed by high resolution mass spectrometry. The solution was evaporated and the residue was purified by silica gel chromatography using dichloromethane–triethylamine (10
:
1). 1H-NMR (400 MHz, CD3OD): δ 8.10 (d, 1H, J = 7.60 Hz), 7.67 (m, 4H), 7.18 (m, 2H), 7.05 (m, 4H), 6.60 (m, 1H), 5.50 (s, 1H), 3.71 (q, 8H, J = 7.16 Hz), 3.21 (m, 3H), 1.31 (t, 12H, J = 2.8 Hz); HRMS (C31H35N2O3+) calculated (M+): 483.2642, found: 483.2650.Ratiometric titration of ThioRB-FITC-MSNs for HOCl
Aliquots of ThioRB-FITC-MSN stock solution (50 mg ml−1) in water were added to Na2HPO4–citrate buffer (100 mM, pH 5) or PBS (pH 7.4) supplemented with various amounts of NaOCl to a final concentration of 1 mg ml−1. The fluorescence emission spectra were recorded as a function of NaOCl concentration using λex@490 nm or λex@560 nm.Selectivity of ThioRB-FITC-MSN for HOCl over selected chemical species
The reactive nitrogen species and reactive oxygen species were prepared following published procedures.3c Briefly, O2−˙ was generated by adding KO2 to the assay solutions. ˙OH radicals were generated in situ by the addition of Fe(ClO4)2 and H2O2 into assay solutions. In the case of ROO˙, ammonium persulfate was added directly into the assay solution. Nitric oxide was formed from sodium nitroprusside added to the assay solution.For assays performed in Na2HPO4–citrate buffer (100 mM, pH 5): to a series of solutions of ThioRB-FITC-MSN (1 mg ml−1) were respectively added each of the following compounds: KCl (1 mM), NaCl (1 mM), CuSO4 (1 mM), MnCl2 (1 mM), MgCl2 (1 mM), CaCl2 (1 mM), ZnCl2 (1 mM), Fe(ClO4)3 (1 mM), Fe(ClO4)2 (1 mM), CoCl2 (1 mM), NiCl2 (1 mM), Pb(NO3)2 (1 mM) or NaOCl (0.08 mM); H2O2 (1 mM), ˙OH radicals generated from H2O2 (1 mM) with Fe(ClO4)2 (1 mM), ammonium persulfate (1 mM), sodium nitroprusside (1 mM), KO2 (1 mM) or NaOCl (0.08 mM). The samples were mixed and incubated at rt for 30 min. The fluorescence emission spectra of the samples were recorded using λex@490 nm.
For assays performed in PBS (pH 7.4): the assays were performed using the same procedure as described above with the exception that 0.5 mg ml−1 ThioRB-FITC-MSN and 0.1 mM NaOCl were used in the assays.
Endocytosis of ThioRB-FITC-MSN into lysosomes in living cells
L929 cells were grown at 37 °C under 5% CO2 in DMEM supplemented with 10% fetal bovine serum. Cells were seeded on 35 mm glass-bottomed dishes (NEST) and incubated for 24 h. The cells were further incubated in DMEM supplemented with ThioRB-FITC-MSN (25 μg ml−1) for 2 h. The cells were washed with PBS and then respectively incubated in PBS spiked with or without NaOCl (200 μM) for 10 min. The cells were further incubated in DMEM containing LysoTracker Blue DND-22 (1 μM) for 10 min. Cells were then analyzed by confocal fluorescence microscopy.Imaging of HOCl with ThioRB-ester in L929 cells
L929 cells were seeded on 35 mm glass-bottomed dishes (NEST) and incubated for 24 h, and then further incubated in DMEM containing ThioRB-ester (10 μM) for 1 h. The cells were then incubated in PBS spiked with or without NaOCl (200 μM) for 15 min. The cells were then cultured in DMEM containing LysoTracker Blue DND-22 for 10 min. Cells were washed with fresh DMEM and then analyzed by confocal fluorescence microscopy.Ratiometric assay of lysosomal HOCl with ThioRB-FITC-MSN by flow cytometry
L929 cells and HeLa cells were respectively cultured in DMEM containing ThioRB-FITC-MSN (25 μg ml−1) for 2 h, and then incubated in PBS supplemented with various amounts of NaOCl (0, 0.1, 0.5, 1 mM) for 10 min. Cells harvested by trypsin digestion were washed with PBS and then analyzed by flow cytometry.Cytotoxicity of ThioRB-FITC-MSN
L929 cells were cultured with DMEM containing ThioRB-FITC-MSN (0, 10, 25, 50, 100 μg ml−1) for various periods of time (0–24 h) at 37 °C with 5% CO2. Cell viability was determined by trypan blue exclusion test.Results and discussion
Design and synthesis of a small molecule fluorogenic chemodosimeter for HOCl
To develop a selective chemodosimeter for HOCl that can be immobilized into silica nanoparticles, ThioRB-ester was designed and synthesized as shown in Scheme 1. Historically, a rhodamine derivative containing an intramolecular spirothioether moiety has been reported for the fluorogenic sensing of OCl− ions via analyte triggered oxidative opening of the spirothioether.3b ThioRB-ester is a bifunctional molecule comprised of a nonfluorescent rhodamine-spirothioether functionality which is HOCl responsive and an ester group that can be exploited for subsequent immobilization in MSN. Reduction of rhodamine B with lithium aluminium hydride in diethyl ether gave 1 in 65% yield. Oxidation of 1 with pyridinium chlorochromate (PCC) in dichloromethane afforded 2 in 80% yield. Treatment of 2 with (methoxycarbonylmethylene)triphenylphosphorane in methanol at room temperature gave rhodamine-cinnamate, methyl ester (RB-CM) as a deep red colored solid in 85% yield. Treatment of RB-CM with hydrosulfide (HS−) in dimethylformamide readily afforded nonfluorescent and colorless ThioRB-ester via analyte triggered tandem Michael addition and intramolecular cyclization (Scheme 1).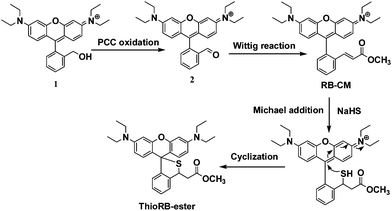 |
| Scheme 1 Synthesis of HOCl activatable rhodamine-spirothioether based chemodosimeter for incorporation into silica nanoparticles. | |
Preparation and characterization of the dual-colored nanodosimeter containing a HOCl responsive motif
Aminolysis of ThioRB-ester in (3-aminopropyl)triethoxysilane (APTS) afforded ThioRB-APTS (ESI,† Scheme S1). Co-condensation of ThioRB-APTS with FITC-APTS and tetraethyl orthosilicate (TEOS) in the presence of cetyltrimethylammonium bromide (CTAB) readily afforded silica nanoparticles doped with FITC and ThioRB, which were further processed following a published procedure to remove CTAB.5 Transmission electron microscopy (TEM) and scanning electron microscopy (SEM) images showed that the as-prepared porous nanoparticles are uniform in size (Fig. 2A and B). To minimize nonspecific interactions in subsequent cell studies and increase the colloidal stability, the dual colored nanoparticles were further pegylated to give the desired nanodosimeter (referred to as ThioRB-FITC-MSN). Dynamic light scattering analysis revealed that the average diameter of pegylated MSNs increased to 140 nm as compared to unmodified MSN (91.3 nm) (Fig. 2D). Consistently zeta potential of the pegylated MSN decreased from 28.8 mV to −33.9 mV after modification (Fig. 2C), indicating efficient pegylation of the nanoparticles.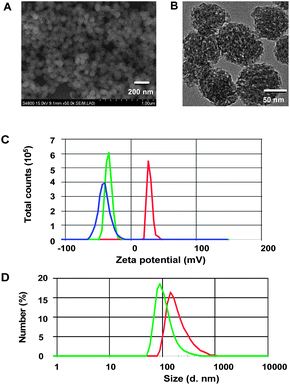 |
| Fig. 2 Physical properties of ThioRB-FITC-MSN. SEM (A) and TEM (B) images of the dual colored nanodosimeter before pegylation; (C) zeta potential of the nanodosimeter (1 mg ml−1) in water before (in red) and after pegylation (ThioRB-FITC-MSN, in green) as compared to ThioRB-FITC-MSN (1 mg ml−1) in water supplemented with NaOCl (100 μM) (in blue), and (D) diameter size of non-pegylated nanodosimeter (1 mg ml−1) (in green) and ThioRB-FITC-MSN (in red) in water as measured by dynamic light scattering. | |
To probe the reactivity of ThioRB attached MSN, various amounts of NaOCl were spiked into phosphate buffered saline (PBS) containing ThioRB-FITC-MSN. Formation of fluorescent species in solution was monitored by fluorometry. Time course studies showed that the reactions completed immediately upon addition of various levels of NaOCl (5–100 μM) (ESI,† Fig. S1). The intense fluorescence centered at 586 nm suggested opening of the spirothioether of ThioRB doped in MSN. In contrast, albeit responsive to HOCl in acetonitrile and PBS buffered acetonitrile (ESI,† Fig. S5), ThioRB ester, the free small molecule chemodosimeter, failed to detect NaOCl in PBS (Fig. 3A and D). The findings revealed the unexpected and yet essential role of the silica platforms of the reactive nanoprobe in sensing HOCl in aqueous media.
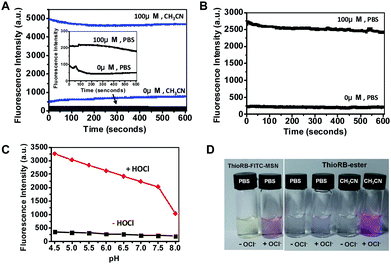 |
| Fig. 3 Chemical properties of ThioRB-FITC-MSN. (A) HOCl mediated turn-on fluorescence of ThioRB-ester (10 μM) in PBS (dark line) or acetonitrile (blue line) spiked with or without NaOCl (100 μM); the inset shows the zoom of the kinetic profiles of ThioRB-ester in PBS; (B) kinetic profiles of HOCl triggered activation of the spirothioether of ThioRB-FITC-MSN (1 mg ml−1) in PBS spiked with or without NaOCl (100 μM); (C) titration of ThioRB-FITC-MSN (1 mg ml−1) in Na2HPO4–H3PO4 buffer (200 mM) of various pH values supplemented with or without NaOCl (100 μM); the pH profile of ThioRB-FITC-MSN is shown as a dark line while the pH correlated titration curve on activation of ThioRB-FITC-MSN by HOCl is shown in red; the fluorescence intensity at 586 nm was monitored using an excitation wavelength of 560 nm; (D) visual images of ThioRB-FITC-MSN (1 mg ml−1) and ThioRB-ester (10 μM) in PBS or acetonitrile supplemented with or without NaOCl (100 μM). | |
To probe the assay mechanism, the fluorescent species generated from ThioRB-ester and HOCl in aqueous acetonitrile was characterized using high resolution mass spectrometry. A major peak located at 483.2650 was revealed which is consistent with the theoretical molecular weight of RB-CM (ESI,† Fig. S2). Formation of RB-CM was further confirmed by 1H-NMR analysis of the colored species generated in the assay solution, indicating β-elimination of the in situ generated rhodamine-sulfonyl chloride intermediate (Scheme 2B). Compared with the reported hydrolysis of a rhodamine-sulfonyl chloride intermediate generated in HOCl sensing with a structurally analogous chemodosimeter (Scheme 2A),3b the occurrence of β-elimination in the ThioRB-ester based assay was presumably due to the increased acidity of the proton next to the carbonyl group (Scheme 2B).
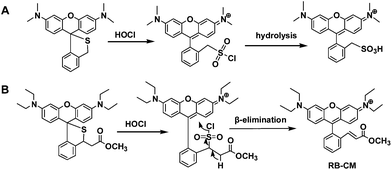 |
| Scheme 2 Sensing mechanism of ThioRB-ester (B) to HOCl as compared to that of a reported chemodosimeter (A).3b | |
Ratiometric responses and selectivity of ThioRB-FITC-MSN to HOCl
To access the ratiometric responses of ThioRB-FITC-MSN towards HOCl, various amounts of NaOCl were supplemented in PBS (pH 7.4) or Na2HPO4–citrate buffer (pH 5.0) in the presence of ThioRB-FITC-MSN. Fig. 4 shows that the fluorescence emission of activated ThioRB which was centered at 586 nm intensified as a function of NaOCl concentration whereas a concomitant decrease in fluorescein emission was observed in Na2HPO4–citrate buffer of lysosomal pH, proving efficient FRET between the fluorescein donor and the newly formed rhodamine acceptor by single wavelength excitation of the fluorescein donor or dual wavelength excitation (ESI,† Fig. S3). The ratio of fluorescence intensities (I586nm/I526nm) increased significantly, enabling estimation of HOCl concentrations from ratios of the fluorescence intensities of the dual-colored nanoprobe. In parallel experiments, ratiometric responses of ThioRB-FITC-MSN towards HOCl were also shown to be efficient in PBS of cytosolic pH (pH 7.4) (ESI,† Fig. S4). As low as 5 μM HOCl can be detected in buffers of pH 5.0 or 7.4.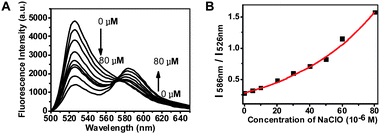 |
| Fig. 4 Titration of ThioRB-FITC-MSN with HOCl in Na2HPO4–citrate buffer (100 mM, pH 5.0) by fluorometry. (A) Fluorescence emission spectra of ThioRB-FITC-MSN (1 mg ml−1) in Na2HPO4–citrate buffer spiked with NaOCl recorded using an excitation wavelength of 490 nm. Analyte concentrations used: 0, 5, 10, 20, 30, 40, 50, 60, and 80 μM; (B) titration curve plotted with fluorescence emission intensity at 586 nm over that at 526 nm as a function of HOCl concentration. | |
Lysosomes are intracellular vesicles with acidic luminal pH (6.0–4.5). Rhodamine (deoxy)lactams, the structural analogs of ThioRB-ester, have been employed for fluorescent staining of lysosomes via proton mediated opening of the intramolecular lactams.6 To image lysosomal HOCl, it is essential that the ThioRB moiety displayed on the nanodosimeter is inert to lysosomal acidity. pH titration of ThioRB-FITC-MSN in buffers of different pH showed that the ThioRB group remained non-emissive in both cytosolic pH and the pH window of lysosomes (Fig. 3C). Being capable of forming gaseous chlorine or OCl− ions in aqueous solution, HOCl is the predominant species in acidic media of pH 4–6.7 The pH dependent activation of the nanodosimeter with HOCl revealed that HOCl is an effective species to activate the ThioRB moiety in the nanoprobe (Fig. 3C), which is consistent with the documentation that HOCl is a superior oxidizer relative to OCl− ions in certain organic transformations.8 Collectively, these results suggested the applicability of the nanodosimeter in ratiometric and real-time imaging of HOCl under both cytosolic and lysosomal pH.
ThioRB-FITC-MSN was further evaluated for its selectivity towards potential interferents that could be present in biological specimens, e.g. reactive oxygen species and reactive nitrogen species. Compared with the dramatic fluorescence response of ThioRB-FITC-MSN towards HOCl, negligible emission of ThioRB-FITC-MSN was observed in buffers containing H2O2, nitric oxide (NO), ˙OH, ROO˙ or O2−˙ at concentrations up to 1 mM in buffers of pH 5.0 or pH 7.4 (Fig. 5B, ESI,† Fig. S7). Additionally, no influence on ThioRB-FITC-MSN was observed in buffers (pH 5 or 7.4) supplemented with various metal ions such as Na+, K+, Ca2+, Zn2+, Pb2+, Fe3+ and Fe2+ (Fig. 5A, ESI,† Fig. S8). These findings demonstrated the stringent sensitivity of ThioRB-FITC-MSN to HOCl over a variety of interfering species that could be present in cells.
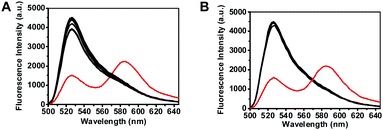 |
| Fig. 5 Selectivity of ThioRB-FITC-MSN for HOCl over selected cations or reactive oxygen species in Na2HPO4–citrate buffer (100 mM, pH 5.0). The fluorescence emission of the nanodosimeter in buffers spiked with various analytes which was due to opening of the ThioRB moiety was recorded using an excitation wavelength of 490 nm. (A) Fluorescence spectra of ThioRB-FITC-MSN (1 mg ml−1) in Na2HPO4–citrate buffer with no addition or with each of the following species (1 mM): K+, Na+, Cu2+, Mn2+, Mg2+, Ca2+, Zn2+, Fe3+, Fe2+, Co2+, Ni2+, Pb2+, or HOCl (0.08 mM, in red); (B) fluorescence spectra of ThioRB-FITC-MSN (1 mg ml−1) in Na2HPO4–citrate buffer with no addition, or with each of the following species (1 mM): H2O2, ˙OH, ROO˙, NO, O2−˙ or HOCl (0.08 mM, in red) (λex@490 nm). | |
Site-specific internalization of ThioRB-FITC-MSN into lysosomes in living cells
With the advantageous features demonstrated, we next investigated the feasibility of imaging lysosomal HOCl with ThioRB-FITC-MSN. MSNs, readily internalized into cells by endocytosis, are often accumulated in lysosomes,9 which underlies our proposed regioselective detection of lysosomal HOCl with ThioRB-FITC-MSN in living cells. To determine the intracellular locations of internalized ThioRB-FITC-MSN, L929 cells were co-stained with ThioRB-FITC-MSN and LysoTracker Blue DND-22 (referred to as LysoTracker Blue) which is an established lysosome marker, and then incubated in PBS supplemented with NaOCl for 10 min. The cells were rinsed and then visualized with confocal fluorescence microscopy. Rhodamine and FITC signals, both clearly present in cells, overlayed with the fluorescence of LysoTracker Blue, demonstrate that ThioRB-FITC-MSN was site-specifically internalized in lysosomes in living cells (Fig. 6).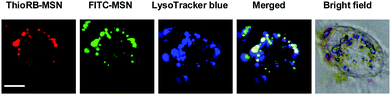 |
| Fig. 6 Intracellular distribution of ThioRB-FITC-MSN in L929 cells treated with NaOCl. Cells pre-loaded with LysoTracker Blue (1 μM) and ThioRB-FITC-MSN (25 μg ml−1) were incubated in PBS containing NaOCl (200 μM) for 10 min, and then probed using confocal fluorescence microscopy. Activated ThioRB fluorescence is shown in red, FITC signals are shown in green and those of LysoTracker Blue are shown in blue. The merging of the three signals is shown in white. Bars, 5 μm. | |
Ratiometric imaging of lysosomal HOCl with ThioRB-FITC-MSN
To probe the effectiveness of HOCl imaging, L929 cells pre-loaded with ThioRB-FITC-MSN or ThioRB-ester were respectively incubated in PBS supplemented with or without NaOCl for 10 min. No rhodamine signal was observed in control cells that were incubated in PBS in the absence of NaOCl, whereas intense rhodamine fluorescence was recorded in virtually all cells treated with ThioRB-FITC-MSN and NaOCl, suggesting efficient incorporation of the nanoprobe into the targeted cell populations (Fig. 7A: broad view; B: single cell image) and HOCl mediated opening of the spirothioether of ThioRB-FITC-MSN in lysosomes. In contrast, no obvious rhodamine fluorescence was observed in cells stained with the small molecule chemodosimeter of ThioRB-ester in the absence or presence of HOCl (Fig. 8), highlighting the superior efficacy of ThioRB-FITC-MSN in sensing lysosomal HOCl over ThioRB-ester.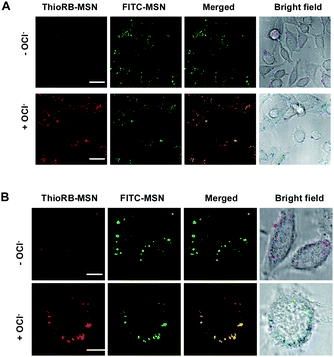 |
| Fig. 7 Imaging of lysosomal HOCl in L929 cells with ThioRB-FITC-MSN. Cells loaded with ThioRB-FITC-MSN (25 μg ml−1) were incubated in PBS spiked with or without NaOCl (200 μM) for 10 min. The cells were analyzed using confocal fluorescence microscopy. Merging of the fluorescence of activated ThioRB (shown in red) and FITC (in green) is shown in yellow. Bars: 20 μm for (A), and 10 μm for (B). | |
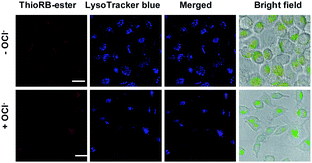 |
| Fig. 8 Detection of HOCl with ThioRB-ester. L929 cells were incubated with ThioRB-ester (10 μM). The cells were washed and then treated with or without NaOCl in PBS for 10 min, followed by supplementing with LysoTracker Blue (1 μM) in DMEM for 10 min. The cells were analyzed by confocal fluorescence microscopy. ThioRB-ester fluorescence is shown in red and that of LysoTracker Blue is shown in blue. Bars: 20 μm. | |
To explore the efficacy of ratiometric reporting of lysosomal HOCl with ThioRB-FITC-MSN, L929 and HeLa cells were respectively loaded with ThioRB-FITC-MSN and then cultured in PBS containing different concentrations of NaOCl. The cells were then analyzed by flow cytometry. As can be seen in Fig. 9B, rhodamine fluorescence (FL2 value) increased as a function of NaOCl concentration, suggesting dose dependent turn-on fluorescence of the ThioRB moiety. FL2/FL1 values, ratios of fluorescein signal over that of rhodamine, are the indicator of FRET efficiency of the nanoprobe inside cells. Analysis revealed that the average FL2/FL1 value of L929 and HeLa cell populations treated with higher levels of NaOCl was significantly elevated relative to cells with no treatment or treated with lower levels of NaOCl (Fig. 9A, ESI,† Fig. S9). Since the slopes of cell populations represent FL2/FL1 values (Fig. 9A), monitoring lysosomal HOCl levels in different cell samples could be readily achieved by comparison of the relative slopes of the cell populations in flow cytometric images. It is noteworthy that the assay is compatible with conventional flow cytometers as single wavelength excitation of ThioRB-FITC-MSN (fluorescein donor) can be achieved by the 488 nm blue laser which is equipped with all flow cytometers.
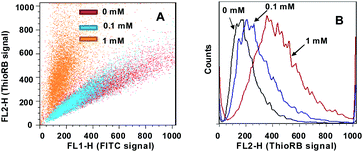 |
| Fig. 9 Flow cytometric analysis of lysosomal HOCl in L929 cells with ThioRB-FITC-MSN under single wavelength excitation (λex@488 nm) (A). (B) Dose-dependent formation of ThioRB fluorescence in L929 cells treated with different levels of NaOCl as indicated. The fluorescence of FITC (FL1) was collected @510–535 nm while that of the ThioRB signal was collected @565–625 nm (FL2). | |
HOCl has been reported to oxidize a wide variety of biomolecules, including proteins, cholesterol, and NADH, etc.10 ThioRB-FITC-MSN, albeit that it effectively detected HOCl in aqueous buffers, failed to detect NaOCl in Dulbecco's Modified Eagle Medium (DMEM) which is a cell culture medium containing a myriad of biomolecules (ESI,† Fig. S6), suggesting the depletion of NaOCl by ingredients of DMEM. Hence, the relaxed assay efficiency for exogenous HOCl in the cell-based flow cytometric assays as compared to that in PBS is partially due to consumption of the spiked NaOCl by cellular components. It is anticipated that endogenous HOCl produced in lysosomes could be more efficiently imaged by the lysosome-residing ThioRB-FITC-MSN.
Cytotoxicity of ThioRB-FITC-MSN
The cytotoxicity of ThioRB-FITC-MSN was evaluated in L929 cells by trypan blue exclusion test. No toxic effects were observed on cell viability after incubation with the nanoprobe for 24 h at doses up to 100 μg ml−1 (Fig. 10). Taken together, the studies demonstrated the utility of the reactive nanoprobes in ratiometric reporting of lysosomal HOCl in living cells.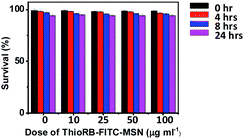 |
| Fig. 10 Cytotoxicity of ThioRB-FITC-MSN. L929 cells were incubated with various amounts of ThioRB-FITC-MSN (0, 10, 25, 50, 100 μg ml−1) for 0–24 h. Cell viability was determined by trypan blue exclusion assay. | |
Conclusions
Self-referenced silica nanoparticles featuring an HOCl activatable chemodosimeter were fabricated for FRET based ratiometric detection of HOCl in aqueous media of different pH values. Particularly, the nanodosimeter is suitable for ratiometric reporting of HOCl levels in lysosomes in living cells. The assay is compatible with conventional flow cytometry via single-wavelength excitation of the fluorescein-rhodamine dye pair in situ generated upon HOCl triggered oxidation, allowing facile monitoring of alterations of intralysosomal HOCl. Disruption of the homeostasis of HOCl generated in lysosomes of immune cells has been linked with a number of chronic diseases. The distinguished biochemical features of the nanodosimeter, e.g. compatibility with aqueous media and stringent chemo-/regioselectivity for lysosomal HOCl, suggest its broad utility for biomedical studies on biogenesis or the impact of HOCl in living cells or in animals.Acknowledgements
Dr S. Han was supported by grants from NSF China 21272196 and 21072162, the Natural Science Foundation of Fujian Province 2011J06004 and the Fundamental Research Funds for the Central Universities 2011121020; Dr J. Han was supported by grants from NSF China 30830092, 30921005, 91029304, 81061160512 and 973 program 2009CB522200.Notes and references
- J. E. Harrison and J. Schultz, J. Biol. Chem., 1976, 251, 1371 CAS.
-
(a) S. M. Wu and S. V. Pizzo, Arch. Biochem. Biophys., 2001, 391, 119 CrossRef CAS;
(b) T. Hasegawa, E. Malle, A. Farhood and H. Jaeschke, Am. J. Physiol.: Gastrointest. Liver Physiol., 2005, 289, G760 CAS;
(c) C. J. Venglarik, J. Giron-Calle, A. F. Wigley, E. Malle, N. Watanabe and H. J. Forman, J. Appl. Physiol., 2003, 95, 2444 CAS;
(d) S. Sugiyama, K. Kugiyama, M. Aikawa, S. Nakamura, H. Ogawa and P. Libby, Arterioscler., Thromb., Vasc. Biol., 2004, 24, 1309 CrossRef CAS;
(e) E. Malle, T. Buch and H. J. Grone, Kidney Int., 2003, 64, 1956 CrossRef CAS;
(f) A. Hammer, G. Desoye, G. Dohr, W. Sattler and E. Malle, Lab. Invest., 2001, 81, 543 Search PubMed;
(g) S. Hammerschmidt, N. Buchler and H. Wahn, Chest, 2002, 121, 573 CrossRef CAS.
-
(a) T. I. Kim, S. Park, Y. Choi and Y. Kim, Chem.–Asian J., 2011, 6, 1358 CrossRef;
(b) S. Kenmoku, Y. Urano, H. Kojima and T. Nagano, J. Am. Chem. Soc., 2007, 129, 7313 CrossRef CAS;
(c) Y. Koide, Y. Urano, K. Hanaoka, T. Terai and T. Nagano, J. Am. Chem. Soc., 2011, 133, 5680 CrossRef CAS;
(d) Y. Zhou, J. Y. Li, K. H. Chu, K. Liu and C. Yao, Chem. Commun., 2012, 48, 4677 RSC;
(e) L. Yuan, W. Lin, Y. Xie, B. Chen and J. Song, Chem.–Eur. J., 2012, 18, 2700 Search PubMed;
(f) J. Shepherd, S. A. Hilderbrand, P. Waterman, J. W. Heinecke, R. Weissleder and P. Libby, Chem. Biol., 2007, 14, 1221 CrossRef CAS;
(g) X. Chen, X. Wang, S. Wang, W. Shi, K. Wang and H. Ma, Chem.–Eur. J., 2008, 14, 4719 CrossRef CAS;
(h) Z. N. Sun, F. Q. Liu, Y. Chen, P. K. Tam and D. Yang, Org. Lett., 2008, 10, 2171 CrossRef CAS;
(i) W. Zhang, C. Guo, L. Liu, J. Qin and C. Yang, Org. Biomol. Chem., 2011, 9, 5560 RSC;
(j) P. Panizzi, M. Nahrendorf, M. Wildgruber, P. Waterman, J. L. Figueiredo, E. Aikawa, J. McCarthy, R. Weissleder and S. A. Hilderbrand, J. Am. Chem. Soc., 2009, 131, 15739 CrossRef CAS;
(k) Y. K. Yang, H. J. Cho, J. Lee, I. Shin and J. Tae, Org. Lett., 2009, 11, 859 CAS;
(l) X. Chen, K. A. Lee, E. M. Ha, K. M. Lee, Y. Y. Seo, H. K. Choi, H. N. Kim, M. J. Kim, C. S. Cho, S. Y. Lee, W. J. Lee and J. Yoon, Chem. Commun., 2011, 47, 4373 RSC;
(m) X. Cheng, H. Jia, T. Long, J. Feng, J. Qin and Z. Li, Chem.
Commun., 2011, 47, 11978 RSC.
- G. Chen, F. Song, J. Wang, Z. Yang, S. Sun, J. Fan, X. Qiang, X. Wang, B. Dou and X. Peng, Chem. Commun., 2012, 48, 2949 RSC.
- Y. Zhao, B. G. Trewyn, I. I. Slowing and V. S. Lin, J. Am. Chem. Soc., 2009, 131, 8398 CrossRef CAS.
-
(a) Z. Li, S. Wu, J. Han and S. Han, Analyst, 2011, 136, 3698 RSC;
(b) S. Wu, Z. Li, J. Han and S. Han, Chem. Commun., 2011, 47, 11276 RSC;
(c) Z. Li, Y. Song, Y. Yang, L. Yang, X. Huang, J. Han and S. Han, Chem. Sci., 2012, 3, 2941 RSC.
- M. Deborde and U. V. Gunten, Water Res., 2008, 42, 13 CrossRef CAS.
- R. V. Stevens, K. T. Chapman and H. N. Weller, J. Org. Chem., 1980, 45, 2030 CrossRef CAS.
-
(a) J. Lu, M. Liong, Z. Li, J. I. Zink and F. Tamanoi, Small, 2010, 6, 1794 CrossRef CAS;
(b) J. L. Vivero-Escoto, I. I. Slowing, B. G. Trewyn and V. S. Lin, Small, 2010, 6, 1952 CrossRef CAS.
-
(a) J. Arnhold, O. M. Panasenko, J. Schiller, A. Vladimirov Yu and K. Arnold, Chem. Phys. Lipids, 1995, 78, 55 CrossRef CAS;
(b) A. C. Carr and C. C. Winterbourn, Biochem. J., 1997, 327, 275 Search PubMed;
(c) D. I. Pattison and M. J. Davies, Chem. Res. Toxicol., 2001, 14, 1453 CrossRef CAS;
(d) D. I. Pattison, C. L. Hawkins and M. J. Davies, Biochemistry, 2007, 46, 9853 CrossRef CAS;
(e) C. C. Winterbourn, Biochim. Biophys. Acta, Gen. Subj., 1985, 840, 204 CrossRef CAS;
(f) C. C. Winterbourn, J. J. van den Berg, E. Roitman and F. A. Kuypers, Arch. Biochem. Biophys., 1992, 296, 547 CAS.
Footnote |
† Electronic supplementary information (ESI) available: Characterization of HOCl triggered activation of ThioRB-ester in different media, selectivity of ThioRB-FITC-MSN in cytosolic pH, characterization of the assay mechanism, and cytotoxicity of HOCl on L929 cells. See DOI: 10.1039/c2sc21485g |
|
This journal is © The Royal Society of Chemistry 2013 |
Click here to see how this site uses Cookies. View our privacy policy here.