DOI:
10.1039/C3FO60383K
(Paper)
Food Funct., 2014,
5, 123-128
Effectiveness of treatment of iron deficiency anemia in rats with squid ink melanin–Fe
Received
4th September 2013
, Accepted 4th November 2013
First published on 4th November 2013
Abstract
Iron deficiency anemia (IDA) is the one of the most common nutritional problems and is encountered all over the world. This study analysed the effects of squid ink melanin–Fe (SM–Fe) on IDA in rats. Forty weanling SD male rats were used and thirty-two rats were fed an iron-deficient diet for 4 weeks. Then SM–Fe (dosages of iron is 6 mg kg−1 BW) was given to the IDA rats once a day for 3 weeks by intragastric administration, with FeCl3 and FeSO4 (dosages of iron is 6 mg kg−1 BW) as positive controls. While the IDA model group and the control group were administrated distilled deionized water each day for 3 weeks. The content of haemoglobin (Hb), serum iron (SI), total iron binding capacity (TIBC), serum ferritin (SF), transferrin receptor (sTfR), erythropoietin (EPO), and iron content in the liver and spleen were measured. The results showed that the content of Hb, SI, SF, EPO, iron content in the liver and spleen were significantly increased in the iron supplement groups (SM–Fe, FeCl3 and FeSO4) compared with the model group (P < 0.05), while TIBC and sTfR were significantly decreased in the iron supplement groups compared with the model group (P < 0.05). In comparison with the FeCl3 and FeSO4 groups, a higher bioavailability of iron and fewer side effects were observed in the SM–Fe group. The present study indicated that SM–Fe is an effective source of iron supplement for IDA rats and might be exploited as a new iron fortifier.
Introduction
Iron deficiency anemia (IDA) is one of the highest incidence nutritional-deficiency diseases, which is encountered all over the world, especially in infants and children. The World Health Organization (WHO) estimates that 39% of children younger than 5 years, 48% of children between 5 and 14 years, 42% of all women, and 52% of pregnant women in developing countries are anemic,1 with half having IDA.2 According to the WHO, the frequency of iron deficiency in developing countries is about 2.5 times that of anemia, which is not iron deficiency related.1
IDA can result in a wide variety of adverse outcomes including reduced psychomotor development in infants,3 poor cognitive and motor development in children,4,5 poor pregnancy outcomes,6 decreased immune function,7 tiredness and poor work performance.8
IDA is presently one of the most important nutritional problems that remains to be solved. Targeted iron supplementation, iron fortification of foods, or both, can control iron deficiency in populations.9 Several iron compounds have been used with this preventive objective. One of them, ferrous sulfate contains a high iron content with better absorption, and to a certain extent, may help relieve the symptoms of iron deficiency and anemia, it causes many adverse reactions, such as diarrhoea, epigastric discomfort and constipation.10 Iron bioavailability and side effects from its therapeutic use have stimulated studies on other iron compounds.
A squid contains a 1.3% ink sac of its total weight. About 300 to 400 thousand tons of squid are processed each year in China and a considerable portion are processed in Zhoushan of the Zhejiang province. The ink sac is discarded, which pollutes the environment and depletes a potentially highly useful resource. It is documented that squid ink has many biological properties, such as immunity regulation, anti-tumor, anti-oxidant, anti-radiation and anti-bacterial activities.11–13 The main components of squid ink are melanin and a non-sulfated glycosaminoglycan.14
Melanin accounts for about 15.5% of fresh squid ink, and is a highly irregular polymer consisting of an indole structure,15 which can resist free radical chain reactions as an acceptor of free radicals,16,17 and chelate many kinds of metal ions.18,19 Since squid melanin is a natural product and can be combined with a high content of Fe, it may be developed as a safe and efficient iron supplement. Therefore, in this study, high-purity squid ink melanin (SM) was extracted from squid ink sac, and squid ink melanin–Fe (SM–Fe) was prepared, and the objective of this investigation was to compare the effects of SM–Fe, FeCl3 and FeSO4 on IDA in rats and to determine whether SM–Fe can effectively normalize the body iron status in IDA rats. The possible mechanism of SM–Fe on the effects was studied as well.
Materials and methods
Preparation of the SM–Fe
The ink was obtained from squid (Ommastrephes bartrami) from Zhou-Shan Fishery Company (Zhejiang, China) and stored at −40 °C before use. The melanin was isolated and purified by high-speed centrifugation.20 Then melanin was added into 10 mmol L−1 FeCl3 solution at the ratio of 1
:
250 (w/v) followed by slow stirring at 4 °C for 24 h. The mixture was centrifuged at 8000g for 30 min, and crude SM–Fe was collected. It was then purified by successive washings with distilled water and centrifugation, the pellet was lyophilized using a freeze dryer (see Fig. 1 for the IR spectra of the melanin and melanin–Fe). The iron content of SM–Fe was 85 mg g−1, as determined by atomic absorption spectrophotometry (Perkin-Elmer AA700, USA) with an air-acetylene flame. SM–Fe was stored at −20 °C and prepared as a suspension with distilled deionized water for use.
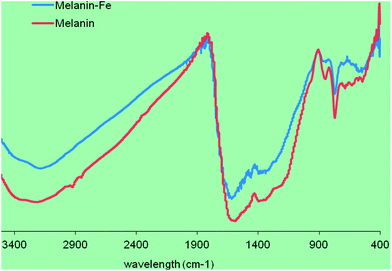 |
| Fig. 1 The IR spectra of the melanin and melanin–Fe. Compared to the native melanin, the intensity of the protonated NH (3200 cm−1), phenolic OH (3400 cm−1) and COOH groups (1710 cm−1) bands decreased notably in melanin–Fe, which indicated the chemical binding of Fe ions with the melanin. | |
Experimental animals
All experimental procedures involving animals received the approval from the Animal Care and Use Committee of Zhejiang University. Guidelines and the Policy on the care and use of laboratory animals were followed at all times. Forty male Sprague-Dawley (SD) rats with an initial weight of 80 ± 5 g were purchased from Shanghai-Silaike Co., and were housed in stainless steel cages, in a temperature-controlled room (24 ± 1 °C, 55 ± 5% humidity) with a 12 h light/dark cycle, and distilled deionized water was provided continuously. After adaptation for 3 days, the rats were randomly divided into the control group and the IDA model groups. Eight rats were selected randomly and given normal diets as the control group. Others were given low iron diets for 4 weeks to generate an IDA animal model.21,22 The whole experimental process was strictly controlled to avoid iron stain. Hb levels were tested weekly, and IDA was defined as Hb values less than 90 g L−1.
Thirty-two IDA rats were randomly assigned into 4 groups of eight animals, each with equal mean Hb values: the IDA model group, the positive groups (FeSO4, dosage of iron is 6 mg kg−1 BW; FeCl3, dosage of iron is 6 mg kg−1 BW), SM–Fe group (dosage of iron is 6 mg kg−1 BW). Rats in the positive groups were administered FeSO4 solution or FeCl3 solution, rats in the SM–Fe group was administered the SM–Fe suspension, while rats in the control and model groups were administered distilled deionized water (placebo). All supplements were freshly prepared every day and intragastric administration was performed once at 10:00 AM each day for 3 weeks.
Table 1 presents a summary of the iron deficient diets supplied during the experimental period. The diets and mineral and vitamin supplements provided were prepared according to recommendations of the AIN (American Institute of Nutrition), containing 5.2 mg iron kg−1 diet.22
Table 1 Ingredient composition of the IDA model diets
Ingredient |
g kg−1 diet |
AIN 76 mineral mix had no iron addition.
|
Cornstarch |
630.0 |
Casein, low trace element |
200.0 |
Fiber |
50.0 |
Corn oil |
50.0 |
Mineral mixa |
35.0 |
Sucrose |
20.0 |
Vitamin mix |
10.0 |
DL-Methionine |
3.0 |
Choline bitartrate |
2.0 |
Tissue and blood collection
After overnight food deprivation, rats were first anesthetized with barbital sodium and blood samples were collected from the abdominal aorta into anticoagulant blood vessels for immediate Hb analysis and into centrifuge tubes for separation of the serum for future analyses. The rats were killed by decapitation and the organs were removed and weighed. The serum was separated by centrifugation at 3000g for 15 min and stored at −20 °C for future analyses. The wet weights of the heart, liver, spleen and kidneys were recorded and tissues were rapidly frozen in liquid nitrogen, and then stored at −80 °C for future analyses.
Chemical analyses
The hemoglobin (Hb) concentrations of the collected blood samples were determined by the cyanmethemoglobin method.23 Serum iron (SI) concentrations and the total iron binding capacity (TIBC) were determined spectrophotometrically using test kits (Nanjing Jiancheng Bioengineering Inst., Nanjing, PR China). The concentrations of serum ferritin (SF), transferrin receptor (sTfR) and erythropoietin (EPO) were measured by the enzyme-linked immunoassays (ELISAs) kits (R&D Systems Inc, USA). The iron content in the rat liver and spleen were determined by atomic absorption spectrophotometry (Perkin-Elmer AA700, USA) with an air-acetylene flame. After the samples had been dried in a vacuum dryer for 48 h and wet digested with a mixture of nitric acid and perchloric acid (3
:
1, v/v). Appropriate dilutions were made by using deionized water. Iron in the diets was measured similarly without prior drying of the samples.
Statistical analysis
All the data were analysed by SPSS 18.0 software for Windows (SPSS Inc., Chicago, IL, USA). The differences between the groups among treatments in each group were determined by one-way analysis of variances ANOVA. Duncan's multiple-range tests were performed. Comparisons of the means with P values equal or less than 0.05 were considered significantly different. Data were expressed as mean ± SD.
Results
The Hb changes of rats
The Hb content in the experiment groups and the control groups were not significantly different at the beginning of the experiment (P > 0.05) (Table 2). The Hb content in the rats fed the iron-deficient diet groups were significantly lower than the control group after 4 weeks depletion (P < 0.05). Therefore, the rats fed the iron-deficient diet induced anemia. After the IDA rats were fed iron supplements (FeCl3, FeSO4 and SM–Fe) for 3 weeks, the Hb content approached normal values, and did not differ from the control group (P > 0.05).
Table 2 The Hb content of rats before depletion, after depletion, and after repletiona
Groups |
Dosage Fe (mg kg−1 bw) |
HB (g L−1) (before depletion) |
HB (g L−1) (after depletion) |
HB (g L−1) (after repletion) |
a and b, means in the same row with no common superscripts differ significantly (P < 0.05).
|
Control |
0 |
115.31 ± 6.01a |
125.13 ± 9.38b |
132.81 ± 13.07b |
Model |
0 |
115.36 ± 7.42a |
86.07 ± 3.40a |
83.88 ± 6.3a |
FeSO4 |
6 |
115.09 ± 8.43a |
85.80 ± 5.27a |
127.32 ± 4.77b |
FeCl3 |
6 |
115.22 ± 7.14a |
85.45 ± 4.76a |
126.79 ± 6.17b |
SM–Fe |
6 |
114.91 ± 16.67a |
85.09 ± 4.17a |
127.95 ± 6.68b |
The body weight changes of rats
The body weight changes of the rats are shown in Table 3. Initially, the mean body weight of the rats in the experiment groups and the control group did not differ (P > 0.05). After 4 weeks of low iron dietary treatment, the mean body weight of the rats in the IDA groups were significantly lower than the control group (P < 0.05). After 3 weeks repletion, the mean body weight of the SM–Fe group, the FeCl3 group and the FeSO4 group were higher than the IDA model group (P < 0.05), but still lower than the control group (P < 0.05).
Table 3 The body weight changes of rats before depletion, after depletion, and after repletiona
Groups |
Dosage Fe (mg kg−1 bw) |
Body weight (before depletion) |
Body weight (after depletion) |
Body weight (after repletion) |
a–c, means in the same row with no common superscripts differ significantly (P < 0.05).
|
Control |
0 |
86.96 ± 7.29a |
261.25 ± 35.79b |
340.75 ± 13.45c |
Model |
0 |
90.35 ± 1.86a |
195.13 ± 24.18a |
239.13 ± 9.40a |
FeSO4 |
6 |
90.75 ± 5.35a |
196.25 ± 11.57a |
258.75 ± 15.42b |
FeCl3 |
6 |
90.23 ± 3.92a |
195.39 ± 12.73a |
258.25 ± 14.32b |
SM–Fe |
6 |
90.03 ± 4.74a |
194.50 ± 18.39a |
258.78 ± 16.25b |
Effects of SM–Fe on organ indices in IDA rats
Table 4 shows the relative tissue (heart, liver, spleen and kidney) weights in each group. The relative weights of the heart, liver and kidney were greater in the IDA rats compared with the control group (P < 0.05). The relative weights of the heart and kidney were greater in the SM–Fe group, the FeCl3 group and the FeSO4 group compared with the control group (P < 0.05). The relative weights of the spleen in the control group, the IDA model group and the iron supplement groups did not differ (P > 0.05).
Table 4 Effects of SM–Fe on organ indices in IDA ratsa
Groups |
Dosage Fe (mg kg−1 BW) |
Heart (g per 100 g) |
Liver (g per 100 g) |
Spleen (g per 100 g) |
Kidney (g per 100 g) |
a and b, means in the same row with no common superscripts differ significantly (P < 0.05).
|
Control |
0 |
0.32 ± 0.01a |
3.57 ± 0.49a |
0.27 ± 0.04a |
0.90 ± 0.04a |
Model |
0 |
0.38 ± 0.04b |
4.06 ± 0.52b |
0.30 ± 0.11a |
1.31 ± 0.24b |
FeSO4 |
6 |
0.36 ± 0.03b |
3.68 ± 0.23ab |
0.27 ± 0.03a |
1.17 ± 0.07b |
FeCl3 |
6 |
0.36 ± 0.05b |
3.67 ± 0.32ab |
0.27 ± 0.04a |
1.16 ± 0.09b |
SM–Fe |
6 |
0.36 ± 0.04b |
3.69 ± 0.51ab |
0.27 ± 0.07a |
1.17 ± 0.14b |
Effects of SM–Fe on SI, TIBC, liver and spleen iron of the IDA model rats
The content of SI, TIBC, liver and spleen iron are shown in Table 5. The iron content of the serum, liver and spleen in the IDA rats were significantly lower than the control group (P < 0.05). The iron content of the serum, liver and spleen in the SM–Fe group, the FeCl3 group and the FeSO4 group rats were significantly higher than the IDA model group (P < 0.05), and did not differ from the control group (P > 0.05). Serum TIBC in the IDA rats was significantly higher than the control group and iron supplement groups (the SM–Fe group, the FeCl3 group and the FeSO4 group) (P < 0.05).
Table 5 Effects of SM–Fe on SI, TIBC, liver and spleen iron of the IDA model ratsa
Groups |
Dosage Fe (mg kg−1 bw) |
SI (mg L−1) |
TIBC (mg L−1) |
Liver iron (mg kg−1) |
Spleen iron (mg kg−1) |
a and b, means in the same row with no common superscripts differ significantly ( P < 0.05).
|
Control |
0 |
16.34 ± 1.19b |
28.84 ± 1.10a |
597.57 ± 23.02b |
1046.77 ± 161.06b |
Model |
0 |
9.73 ± 1.43a |
35.43 ± 1.43b |
285.64 ± 38.92a |
607.94 ± 22.28a |
FeSO4 |
6 |
16.28 ± 1.55b |
28.87 ± 1.36a |
575.57 ± 50.38b |
1032.48 ± 120.84b |
FeCl3 |
6 |
16.13 ± 1.37b |
28.95 ± 1.29a |
573.22 ± 37.57b |
1029.74 ± 97.45b |
SM–Fe |
6 |
16.35 ± 1.52b |
28.77 ± 1.23a |
583.57 ± 35.66b |
1047.97 ± 94.59b |
Effects of SM–Fe on SF, sTfR and EPO in the IDA model rats
The concentrations of the SF, sTfR and EPO are shown in Table 6. The SF and EPO content in the IDA model group were significantly lower than the control group and iron supplement groups (SM–Fe group, the FeCl3 group and the FeSO4 group) (P < 0.05), SF and EPO content in the iron supplement groups were lower than the control group (P < 0.05). The sTfR content in the iron supplement groups (SM–Fe group, the FeCl3 group and the FeSO4 group) were significantly lower than the IDA model group (P < 0.05), but still higher than the control group (P < 0.05).
Table 6 Effects of SM–Fe on SF, sTfR and EPO of the IDA model ratsa
Groups |
Dosage Fe (mg kg−1 bw) |
SF (ng ml−1) |
sTfR (μg ml−1) |
EPO (IU L−1) |
a–d, means in the same row with no common superscripts differ significantly ( P < 0.05).
|
Control |
0 |
104.91 ± 5.71c |
4.04 ± 0.17a |
14.63 ± 0.85c |
Model |
0 |
25.52 ± 2.71a |
7.17 ± 0.17d |
8.03 ± 0.58a |
FeSO4 |
6 |
73.16 ± 2.68b |
5.64 ± 0.21b |
9.92 ± 0.42b |
FeCl3 |
6 |
72.94 ± 2.08b |
5.84 ± 0.21c |
9.77 ± 0.52b |
SM–Fe |
6 |
77.35 ± 2.64b |
5.16 ± 0.11b |
10.33 ± 0.65b |
Discussion
The objective of the present study was to evaluate the effects of SM–Fe supplements on IDA rats. After iron deprivation (5.2 mg kg−1 of diet), hematologic parameters in the IDA group were dramatically different from those of the control group, with a low mean blood Hb concentration. Similarly, SI, SF and EPO concentrations were lower in the IDA group, while TIBC and sTfR concentrations increased markedly due to progressive Fe depletion from the body stores. All these findings were expected and consistent with induced severe IDA in rats. The degree of iron deficiency produced by our iron-restricted diet was severe enough to impair weight gain and to reduce final body weight, as found previously.22,24
The IDA rats had greater absolute and relative wet heart weights, demonstrating the presence of cardiac hypertrophy.25 Also, increases in the wet heart weights and size of the cardiac muscle cells were observed in anemic rats compared with the controls.26 In this study, the relative heart weights in rats fed with SM–Fe, FeCl3 and FeSO4 were significantly greater than the control group.
Iron is necessary for hemoglobin synthesis, it is logical to assume that any iron inadequacy would be reflected in a slower hemoglobin synthetic rate. A marked decrease in hemoglobin and serum iron observed in the rats fed the iron-deficient diet showed depletion of hemoglobin iron.
The liver and spleen are the main storage sites for iron.27 The IDA model rats had lower iron contents in the liver and spleen than the control rats and iron supplement rats. It is then suggested that a marked decrease of iron concentration in the liver and the spleen, showing the depletion of storage iron, and the addition of iron can ameliorate iron storage.
Ferritin is a protein that functions in vivo as an iron storage compound. Although ferritin is primarily intracellular, it can be detected in serum. Serum ferritin closely reflects the level of body stores, where the level of serum ferritin is low for an iron deficiency. Serum ferritin responds quickly to iron treatment or iron deficiency.28 The concentration of serum ferritin reflects the size of the storage iron compartment, if the subject is not in an inflammatory state.29 In this study, the IDA rats had lower serum ferritin than the SM–Fe, FeCl3 and FeSO4 supplement rats. While the IDA rats supplemented with SM–Fe, FeCl3 and FeSO4 showed increased serum ferritin.
The measurement of sTfR concentration in the serum has a diagnostic value for the assessment of IDA.30,31 The amount of sTfR in circulation has been shown to vary with individual iron status. Serum sTfR concentration increased even in mild iron deficiency of recent onset.32 The present study found that the IDA model rats had a higher sTfR concentration than the control rats. When supplemented with iron, the sTfR concentration decreased significantly.
EPO is an important factor for red blood cell production, it can stimulate the proliferation of burst forming unit-erythroid (BFU-E) and differentiation of colony forming unit-erythroid (CFU-E), essential for the amplification and terminal differentiation of erythroid progenitors and precursors.33 Regulation is believed to rely on a feed-back mechanism measuring blood oxygenation.34 The EPO level in the blood is quite low in the absence of anemia. The present study found that the EPO concentration in the IDA rats was lower than for the control rats. While supplement with SM–Fe, FeCl3 and FeSO4 could enhance EPO production and promote erythropoiesis via increasing Hb levels in the IDA rats.
Conclusion
In summary, the present study suggests that SM–Fe is an effective iron supplement and may, through upgrading SF and EPO, enhance iron bioavailability. Further research is required to apply SM–Fe to practical use as a safe and new natural iron fortifier.
Acknowledgements
This research was financially supported by a public service project of Zhejiang Province (2011C22026), a science and technology project of the Science and Technology Bureau of Zhoushan Putuo District of Zhejiang Province, and the postdoctoral scientific research project for preferential financial aid in Zhejiang Province. We thank all of the people who offered help in this study.
References
-
WHO/UNICEF/UNU, Iron Deficiency Anemia Assessment, Prevention and Control, World Health Organization, Geneva, 2001 Search PubMed.
- E. Mclean, M. Cogswell, I. Egli, D. Wijdyla and B. Benoist, Worldwide prevalence of anaemia, WHO vitamin and mineral nutrition information system, 1993–2005, Public Health Nutr., 2008, 12, 444–454 CrossRef PubMed.
- T. Walter, I. De Andraca, P. Chadud and C. G. Perales, Iron deficiency anaemia: adverse effects on infant psychomotor development, Pediatrics, 1986, 84, 7–17 Search PubMed.
- S. Grantham-McGregor and C. Ani, A review of studies on the effect of iron deficiency on cognitive development in children, J. Nutr., 2001, 131, 649S–668S CAS.
- H. P. S. Sachdev, T. Gera and P. Nestel, Effect of iron supplementation on mental and motor development in children: systematic review of randomised controlled trials, Public Health Nutr., 2005, 8, 117–132 CrossRef.
- A. Levy, D. Fraser, M. Katz, M. Mazor and E. Sheiner, Maternal anemia during pregnancy is an independent risk factor for low birth weight and preterm delivery, Eur. J. Obstet. Gynecol. Reprod. Biol., 2005, 122, 182–186 CrossRef PubMed.
- N. Ahluwalia, J. Q. Sun, D. Deanna Krause, A. Mastro and G. Handte, Immune function is impaired in iron-deficient, homebound, older women, Am. J. Clin. Nutr., 2004, 79, 516–521 CAS.
- R. Moench-Pfanner, S. Pee, M. W. Bloem, D. Foote, S. Kosen and P. Webb, Food-for-work programs in indonesia had a limited effect on anemia, J. Nutr., 2005, 135, 1423–1429 CAS.
- P. Lucca, R. Hurrell and I. Potrykus, Fighting iron deficiency anemia with iron-rich rice, J. Am. Coll. Nutr., 2002, 21, 184S–190S CrossRef CAS.
- A. I. Souza, M. B. Filho, C. C. Bresani, L. O. C. Ferreira and J. N. Figueiroa, Adherence and side effects of three ferrous sulfate treatment regimens on anemic pregnant women in clinical trials, Cad. Saúde Pública, 2009, 25, 1225–1233 CrossRef PubMed.
- J. Sasaki, K. Ishita, Y. Takaya, H. Uchisawa and H. Matsue, Anti-tumor activity of squid ink, J. Nutr. Sci. Vitaminol., 1997, 43, 455–461 CrossRef CAS.
- C. L. Lv, J. Z. Yan, P. L. Hu and S. He, Activation of squid ink extracts on macrophages in vivo, J. China Med. Univ., 1999, 18, 410–411 Search PubMed.
- H. Z. Liu, P. Luo, S. H. Chen and J. H. Shang, Effects of squid ink on growth performance, antioxidant functions and immunity in growing broiler chickens, Asian-Australas. J. Anim. Sci., 2011, 24, 1752–1756 CrossRef CAS.
- S. Chen, J. Xu, C. Xue, P. Dong, W. Sheng and G. Yu,
et al. Sequence determination of a non-sulfated glycosaminoglycan-like polysaccharide from melanin-free ink of the squid Ommastrephes bartrami by negative-ion electrospray tandem mass spectrometry and NMR spectroscopy, Glycoconjugate J., 2008, 25, 481–492 CrossRef CAS PubMed.
- M. S. Blois, A. B. Zahlan and J. E. Maling, Electron spin resonance studies on melanin, Biophys. J., 1964, 4, 471–490 CrossRef CAS.
- M. Luciana, B. Carla, C. Raffaella, F. Cesira and A. R. Maria, Melanins from tetrahydroisoquinoles: spectroscopic characteristics, scavenging activity and redox transfer properties, Free Radical Biol. Med., 1998, 24, 161–167 CrossRef.
- S. G. Chen, C. H. Xue, Y. Xue, Z. J. Li, X. Gao and Q. Ma, Studies on the free radical scavenging activities of melanin from squid ink, Chin. J. Mar. Drugs, 2007, 26, 24–27 CAS.
- S. G. Chen, Y. Xue, C. H. Xue, Y. J. Li and L. A. Yin, Adsorption of Fe(III) by squid melanin, Ion Exch. Adsorpt., 2010, 26, 310–316 CAS.
- S. G. Chen, Z. L. Li, C. H. Xue, J. F. Wang, H. Feng and Y. M. Wang,
et al., Adsorption of heavy metals with squid Ommastrephes bartrami ink melanin, Bioinorg. Chem. Appl., 2009, 2009, 1–7 CrossRef PubMed.
- Y. Liu and J. D. Simon, The effect of preparation procedures on the morphology of melanin from the ink sac of Sepia officinalis, Pigm. Cell Res., 2003, 16, 72–80 CrossRef CAS.
- Q. K. Liao and C. H. Luo, Establishment of iron deficiency anemia rat model, Chin. J. Hematol., 1990, 11, 156–157 Search PubMed.
- M. J. Borel, S. H. Smith, D. E. Brigham and J. L. Beard, The impact of varying degrees of iron nutrition on several functional consequences of iron deficiency in rats, J. Nutr., 1991, 121, 729–736 CAS.
-
I. Davidsohn and D. A. Nelson, The blood, in Clinical Diagnosis by Laboratory Methods, ed. I. Davidsohn and J. B. Henry, W. B. Saunders, Philadelphia, PA, 1974, pp. 100–310 Search PubMed.
- Y. N. J. Strube, J. L. Beard and A. C. Ross, Iron deficiency and marginal vitamin A deficiency affect growth hematological indices and the regulation of iron metabolism genes in rats, J. Nutr., 2002, 132, 3607–3615 CAS.
- D. M. Medeiros and J. L. Beard, Dietary iron deficiency results in cardiac eccentric hypertrophy in rats, Exp. Biol. Med., 1998, 218, 370–375 CrossRef CAS.
- M. A. Rossi, S. V. Carillo and J. S. Oliveira, The effect of iron deficiency anemia in the rat on catecholamine levels and heart morphology, Cardiovasc. Res., 1981, 15, 313–519 CrossRef CAS PubMed.
- J. Emerit, C. Beaumont and F. Trivin, Iron metabolism, free radicals, and oxidative injury, Biomed. Pharmacother., 2001, 55, 333–339 CrossRef CAS.
- A. S. Pollock, D. A. Lipschitz and J. D. Cook, The kinetics of serum ferritin, Exp. Biol. Med., 1978, 157, 481–485 CrossRef CAS.
- J. J. Powell, W. B. Cook, M. Chatfield, C. Hutchinson, D. I. A. Pereira and M. C. E. Lomer, Iron status is inversely associated with dietary iron intakes in patients with inactive or mildly active inflammatory bowel disease, Nutr. Metab., 2013, 10, 18 CAS.
- B. S. Skikne, Serum transferrin receptor, Am. J. Hematol., 2008, 83, 872–875 CrossRef CAS PubMed.
- F. H. Wians Jr, J. E. Urban, J. H. Keffer and S. H. Kroft, Discriminating between iron deficiency anemia and anemia of chronic disease using traditional indices of iron status vs transferrin receptor concentration, Am. J. Clin. Pathol., 2001, 115, 112–118 CrossRef CAS PubMed.
- J. W. Choi, Sensitivity, specificity, and predictive value of serum soluble transferrin receptor at different stages of iron deficiency, Ann. Clin. Lab. Sci., 2005, 35, 435–439 CAS.
- W. Jelkmann, Erythropoietin after a century of research: younger than ever, Eur. J. Haematol., 2007, 78, 183–205 CrossRef CAS PubMed.
- M. A. Goldberg, S. P. Dunning and H. F. Bunn, Regulation of the erythopoietin gene: evidence that the oxygen sensor is a heme protein, Science, 1988, 242, 1412–1415 CAS.
|
This journal is © The Royal Society of Chemistry 2014 |
Click here to see how this site uses Cookies. View our privacy policy here.