SU-79: a novel germanate with 3D 10- and 11-ring channels templated by a square-planar nickel complex†
Received
20th November 2013
, Accepted 23rd January 2014
First published on 5th March 2014
Abstract
An open-framework germanate denoted as SU-79 with the chemical formula [Ge12.5O26(OH)2][Ni(C3N2H10)2]1.1(NH4)0.8(C3N2H12)0.5(C3N2H10)1.5(H2O)2 has been synthesized under hydro/solvothermal conditions using [Ni(1,2-pda)2]2+ (1,2-pda = 1,2-diaminopropane) and 1,2-pda as templates. Owing to the complicated pseudo-merohedral twinning in the crystals, the rotation electron diffraction (RED) method was used for the unit cell and space group determination. The structure of SU-79 was solved and refined based on synchrotron single crystal X-ray diffraction data. SU-79 exhibits a 3D open germanate framework built with Ge13 clusters, consisting of a 3D channel system with interconnected 10- and 11-ring channels. Interestingly, helical GeO4 tetrahedral chains with left-handed/right-handed chirality were found in the structure. The [Ni(1,2-pda)2]2+ complexes, adopting in a square-planar geometry, show a structure directing role on the SU-79 framework via hydrogen bonds. Comparing with its related structure, SU-67, the formation of the pseudo-merohedric twinning in SU-79 was also discussed.
Introduction
Open-framework germanates are a relatively new family of microporous compounds and have been of particular interest in the past couple of decades due to their structural diversity.1 Germanate frameworks can be built not only of tetrahedral building units to form zeolitic structures but more often, more than one type of polyhedral building units are found in the framework, such as trigonal bipyramids and octahedra. Through corner- or edge-shared oxygen atoms, these polyhedral units can be linked into large clusters. In general, there are four commonly formed germanium clusters: Ge7(O,OH,F)19 (Ge7),2 Ge8(O,OH,F)20 (Ge8),3 Ge9(O,OH,F)25–26 (Ge9)4 and Ge10(O,OH)28 (Ge10).5 The tendency to form these clusters opens opportunities to develop new open-framework materials with wide structural diversity and large pore sizes.
Various species, from alkaline metal ions, organic amines to transition metal complexes, have been used as structure directing agents (SDAs) in the synthesis of a large number of new frameworks. The use of nickel organic complexes in the synthesis of germanates was pioneered by Yang's and Yu's group, which led to the discovery of several novel germanates, such as FJ-1,6 FJ-67 and JLG-4.8 In these compounds, the nickel complexes show remarkable structure-directing effects for the formation of germanate frameworks. More interestingly, in FJ-1, nickel complexes show a capacity of transferring their chirality to the framework. This concept of chirality transfer of the metal complex into the inorganic framework has been further demonstrated in the synthesis of GaGeO-CJ63,9 a gallogermanate zeolite with racemic [Ni(en)3]2+ (en = ethylenediamine) cations as an SDA. In the aforementioned work, nickel complexes are in an octahedral geometry and mainly play a structure directing role in the formation of the germanate framework and as counter ions to balance negative framework charges. SUT-1 and SUT-210 are the first examples where nickel complexes are directly linked to the framework and act as both templates and framework-forming units. More recently, in a newly reported germanate compound, [Ni(H2O)(μ-C2H6NO)2Ge7O14(C2H7NO)]·C2H5OH,11 a Ni complex moiety, [Ni(H2O)(μ-C2H6NO)2], also acts as framework units and links the 2D layers of Ge7 clusters into a three-dimensional network. Here, we present a new 3D open-framework germanate SU-79 with a square-planar nickel complex [Ni(1,2-pda)2]2+ (1,2-pda = 1,2-diaminopropane) acting as an SDA. The SU-79 framework is built with a double square layer of Ge10 clusters. The same building unit was recently reported in the open-framework germanate SU-67 with intersecting 10- and 11-ring.12 The different interactions between the SDAs and the framework resulted in a different stacking of the double square net layers, as well as the pore structure in SU-79, compared to those in SU-67.
Experiments
Synthesis
SU-79 was synthesized under mild hydro/solvothermal conditions from a mixture of GeO2, Ni(NO3)2·6H2O, 1,2-diaminopropane (1,2-pda), pyridine and water with a typical molar ratio of 1.00
:
0.12
:
40
:
26
:
78. The mixture was stirred constantly until a clear pink solution was formed. The solution was then transferred into a 30 mL Teflon-lined stainless steel autoclave. After being kept statically at 180 °C for 7 days, yellow square plate crystals with a typical size of 50 × 50 × 10 μm (Fig. 1a) were obtained by filtration, washed with deionized water and dried at room temperature.
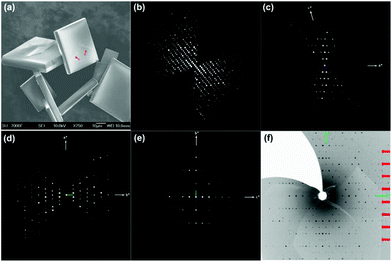 |
| Fig. 1 (a) SEM image of SU-79 crystals. The twinning grain boundaries were marked by the red arrows. (b) The 3D reciprocal lattice reconstructed from the RED data, from which the unit cell of SU-79 could be determined. (c–e) 2D slices cut from the reconstructed 3D reciprocal lattice showing the (c) h0l, (d) hk0 and (e) 0kl plane. (f) The 0kl slice is obtained from the single crystal XRD data. The additional reflection rows due to the twinning are indicated by the red arrows in (f). | |
Single crystal X-ray diffraction
Single crystal X-ray diffraction data of SU-79 were collected at 150(2) K on the Beamline I19, Diamond Light Source, Ltd, Didcot, UK, using a Rigaku CrystalLogic Kappa goniometer with a Saturn 724+ detector using synchrotron radiation (λ = 0.6889 Å). Data reduction and empirical absorption correction were applied using CrysAlisPro. The structure was solved by direct methods using the program SHELXS-97.13 All heavy atoms (Ge, Ni) could be located directly from the difference Fourier maps. Framework hydrogen atoms were placed geometrically and constrained using the riding model to the parent atoms. Finally structure refinement was done with the program SHELXL-9713 by minimizing the sum of squared deviations of F2 using a full-matrix technique. X-ray crystallographic data in CIF format for the structure can be found in ESI.† CCDC 970381.
Rotation electron diffraction
An SU-79 powder sample was dispersed in absolute ethanol and treated by ultra-sonication for three minutes. One droplet of the suspension was transferred onto a carbon-coated copper grid. Three-dimensional rotation electron diffraction (RED) data of SU-79 were collected at 200 kV using the software RED-data collection14 on a JEOL JEM2100 TEM. The RED data were collected in the selected-area electron diffraction (SAED) mode. During the RED data collection, the electron beam was fully spread over the whole phosphorus screen. The selected-area aperture used for RED data collection was about 1.6 μm in diameter. We tracked the crystal in image mode to ensure that the entire crystal was inside the aperture throughout the data collection. In total, 275 ED frames were recorded using a single-tilt tomography sample holder. The crystal tilt range was from −61.57° to 49.80° with a tilt step of 0.60°. Each ED frame was recorded at spot size 3 with the exposure time of 1.4 s. The total data collection time was 16 minutes. The data processing was conducted using the software RED—data processing, including peak search, unit cell determination, and indexation of reflections.
General characterizations
X-ray powder diffraction of SU-79 was collected at room temperature at Diamond Light Source, Beamline I11, λ = 0.82695 Å. A powder sample of SU-79 was loaded into a 0.7 mm borosilicate glass capillary tube which was spinning during data collection. Thermo-gravimetric (TG) analysis was conducted using a Perkin-Elmer TGA7 in air from 40 to 900 °C at a heating rate of 10 °C min−1. Elemental analysis of carbon, nitrogen and hydrogen was carried out with an Elementar Vario EL III microanalyzer. The elemental ratio (Ge/Ni) was measured using the inductively coupled plasma (ICP) method on an ESCALAB2000 analyzer. FT-IR spectroscopy was performed on a Varian 670-IR spectrometer in the region of 4000–400 cm−1. The morphology of SU-79 crystals was examined under a JSM-7000F scanning electron microscope (SEM) with an accelerating voltage of 10 kV. UV-visible spectra were acquired from single crystals of SU-79, using a Cary 500 UV-VIS-NIR Spectrophotometer. Crystals were placed on quartz slides under Krytox oil, and the data were collected from 200 nm to 1000 nm. X-ray photoelectron spectroscopy (XPS) analysis was performed on a Kratos AXIS Ultra Imaging X-ray Photoelectron Spectrometer with a monochromatized Al Kα X-ray source.
Results and discussion
The structure of SU-79 was determined by the combination of synchrotron single crystal X-ray diffraction and rotation electron diffraction (RED) techniques. The single crystal synchrotron diffraction data were collected from one of the square plate crystals. However, the direct indexing of the data results in unreliable unit cell parameters and many unindexed reflections. This indicates the presence of serious twinning in the crystal, which can be observed as grain boundaries on the crystal surface (Fig. 1a). Therefore, RED, a newly developed method for collecting 3D electron diffraction data on micro- or nano-sized single crystals in a transmission electron microscope,14,15 was used for unit cell determination on SU-79. A series of ED patterns were collected from a selected micro-domain (about 1 × 1 μm) of the SU-79 crystal (Fig. 1b–e and Fig. S1†). The 3D reciprocal lattice was reconstructed from the RED data, from which the unit cell parameters were determined as a = 37.54 Å, b = 10.48 Å, c = 21.00 Å, α = 89.41°, β = 106.46° and γ = 89.74°. The additional spots observed in the single crystal X-ray diffraction data are absent in the RED data, as shown in the 0kl plane from RED (Fig. 1e) and the 0kl plane from single crystal diffraction (Fig. 1f). This indicates the RED data came from a single twin domain of SU-79. Thus the twinning problem was avoided. The reflection condition could be deduced from the h0l, hk0 and 0kl plane (Fig. 1c–e) as hkl: h + k = 2n and h0l: l = 2n, indicating a monoclinic space group C2/c. The results were confirmed by the Le Bail fitting of powder X-ray diffraction data. With this unit cell, the synchrotron single crystal X-ray diffraction data were successfully indexed with the following three twinning matrices:
and
The structure of SU-79 was then successfully solved based on the single crystal X-ray diffraction data. Combined with the results from CHN elemental analysis, ICP and TG analysis, the chemical composition of SU-79 was finally determined as [Ge12.5O26(OH)2][Ni(C3N2H10)2]1.1(NH4)0.8(C3N2H12)0.5(C3N2H10)1.5(H2O)2, and the details are shown in ESI.†
The framework of SU-79 is built from Ge13 clusters, which consist of a Ge10 cluster,5 one apical GeO4 tetrahedron and two extra GeO3(OH) tetrahedra, as shown in Fig. 2a. The Ge10 clusters link to each other by vertex-sharing to form 2D layers in the bc-plane (Fig. 2b) with 12-ring windows. Three additional tetrahedra (colored in yellow in Fig. 2b) connect the vertex-sharing tetrahedra pairs along the c-axis, which converts the original 12-rings into fan-shaped 11-rings in the bc-plane (Fig. S3†). Two adjacent Ge10 layers, related by the 2-fold rotation along the b-axis, connect to each other by sharing GeO4 tetrahedra, forming a double layer in the bc-plane. The double layers are stacked along the a-axis with a shift of 1/2b and connected through the additional tetrahedra to form a 3D germanate framework, as shown in Fig. 2c. Interestingly, helical GeO4 chains are formed along the c-axis, with left- and right-handed chirality, respectively (Fig. S4†). 2D 10-ring channels are found within the double layer, running along the b- and c-axis, respectively, and forming a channel system that follows a square net. Fig. 2d represents two such channel systems within two neighboring double layers (shown as red and green rods, respectively). Those 2D channels are further connected by 1D zigzag 11-ring channels along the a-axis (blue rods in Fig. 2d), resulting in a 3D channel system.
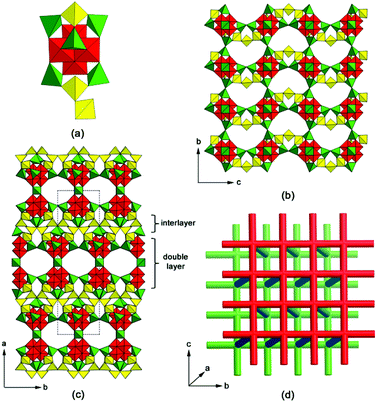 |
| Fig. 2 The framework of SU-79. (a) The basic building unit Ge13 cluster; (b) 2D layer perpendicular to the a-axis, exhibiting fan-shaped 11-ring windows; (c) 10-ring channels along the c-axis are formed by the connection of 2D layers; (d) the channel system in the SU-79 framework. The red and green square nets represent the 2D intersecting 10-ring channels within neighboring double layers, and the blue rods represent zigzag 11-ring channels connecting the 10-ring channels into a 3D channel system. Colour codes in (a)–(c): octahedra, red; tetrahedra inside Ge10 clusters, green; additional tetrahedra, yellow. | |
Topological analysis revealed that the SU-79 framework can be described as a vmu net, a uninodal 8-connected net with the point symbol of {36·416·56} where each node represents a Ge13 cluster. The vertex is first linked to four neighboring vertices to form a 44 square net, which corresponds to the Ge10 layer in Fig. 2b. By connecting the adjacent square net, a double 44 square net layer is formed. Then, three additional edges from each vertex connect the adjacent double 44 square net layers together to generate the 8-connected vmu net. It is worth mentioning that the recently reported open-framework germanate SU-67 is also built by 8-coordinated Ge13 clusters. However, in SU-79, the relationship between the adjacent double layers is the 1/2b shift, while in SU-67, the neighboring double layers are rotated to one another by 90° around the c-axis. The different stacking modes result in different connections between the double layers in SU-79 and in SU-67 and consequently different framework topologies. The structural similarity allows these two germanate frameworks to be derived from a common nce net constructed from the stacking and connection of double 44 nets. To obtain the corresponding nets for SU-79 and SU-67, the linkages between the double 44 net in the nce net are partly removed, according to the different connections between the double layers in SU-79 and SU-67. As a result, the framework of SU-79 adopts the vmu net, while the SU-67 framework has a topology of an nce-8-I41/acd net, as shown in Fig. 3a and 3b, respectively. The alternative connection between the double layers is proposed to be the origin of the twinning problem in SU-79. If some of neighboring double layers in SU-79 crystals are connected by 90° rotation as in SU-67, the twin boundary is formed with two twin domains connected by a 90° rotation along the direction normal to the double layers, i.e. the a*-axis. Thus, in total, four twin domains can be found in the SU-79 crystal, which are related to the original one by a rotation of 90°, 180° and 270°, respectively along the a*-axis and their basic axes are related by the twin matrices aforementioned.
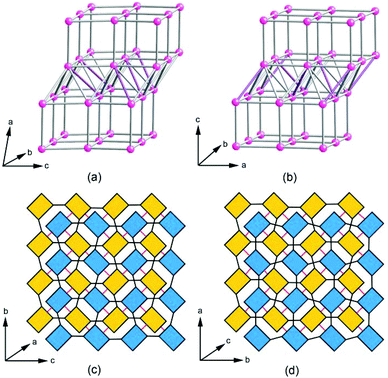 |
| Fig. 3 Topological relation between the (a) SU-79 (vmu) and (b) SU-67 (nce-8-I41/acd) nets. Both nets can be deduced from the nce net by the removal of one of the nine edges (purple) around a vertex. The two different connection modes between the double layers can be illustrated by simplified representations in (c), (d), where the yellow and blue nets represent two double 44 layers. The red lines show the connections between the double layers. In SU-79 (c), the blue layer is shifted by 1/2b with the yellow layer, while in SU-67 (d), the blue layer is rotated by 90° with respective to the yellow layer. | |
In order to understand the formation of different connections between the double layers, the interactions between the guest species and the framework of SU-79 were investigated carefully. The negatively charged SU-79 framework is balanced by [Ni(1,2-pda)2]2+, 1,2-H2pda2+ and NH4+ cations. The 2-H2pda2+ and NH4+ cations and H2O molecules are located in the 10-ring channels within the double layers, while the [Ni(1,2-pda)2]2+ cations are found in the 11-rings between the double layers. Thus, the [Ni(1,2-pda)2]2+ cations could be the key which leads to the connection mode of double layers in SU-79. This nickel complex is 4-coordinated in a square planar mode as confirmed by the UV-Vis diffuse-reflection spectrum. Two absorption bands at 314 nm and 441 nm are observed in the UV-Vis spectrum of SU-79. They can be assigned to d–d transition bands of a [Ni(1,2-pda)2]2+ complex (Fig. S7†), which is similar to that of nickel metformin, a nickel complex with the square-planar geometry. The nickel complex is located in the 11-ring window and shows weak hydrogen-bond interactions with the framework and one water molecule (Ow1). The Ow1 molecule interacts with the framework by strong hydrogen bonding (Ow1⋯O14 2.738 Å and Ow1⋯O27 2.676 Å). The other 1,2-H2pda2+ cation is trapped in the 10-ring channels along the c-axis with a weak hydrogen bonding to the framework. The details of these hydrogen bonds are given in Table S2.†
The hydrogen bonds formed by 2-methypiperazine cations in the 10-ring channels in SU-67 are similar to those in SU-79. However, the hydrogen bonds in the 11-ring windows are quite different, which significantly affect the formation of the different connections between the double layers. Firstly, the larger [Ni(1,2-pda)2]2+ cation is not only hydrogen-bonded within the 11-ring window, but also interacts with the neighboring layer (the blue tetrahedron in Fig. 4a), while in SU-67, the 2-methypiperazine cations only form hydrogen-bonds within the 11-ring windows. Secondly, if one keeps the Ni complex and the same hydrogen-bonds in SU-79, but uses the SU-67 connection mode between the layers, collision of the Ni complex with the next double layer would be observed. This means that the [Ni(1,2-pda)2]2+ cation does not fit into the void between the double layers in SU-67 so that the connection mode in SU-79 is not present in SU-67 crystals and no twinning problem is observed in SU-67.
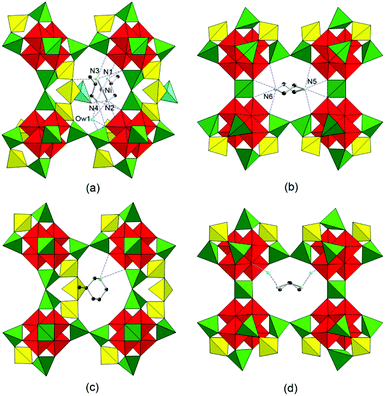 |
| Fig. 4 Representation of the guest species in SU-79. (a) [Ni(1,2-pda)2]2+ cation and O1w residing in the 11-ring window in the bc-plane; (b) 1,2-H2pda2+ cation located in the 10-ring channels along the c-axis. Hydrogen bonds in SU-67 (c, d) are shown for comparison. Colour codes: carbon, black; nitrogen, blue. | |
Conclusions
In summary, a novel open-framework germanate templated by nickel complexes, SU-79, has been synthesized under hydro/solvothermal conditions. Due to the complicated pseudo-merohedric twinning problem in SU-79 crystals, the unit cell parameters and the space group were determined by the RED method. With the information from RED, the structure was then successfully solved based on the single crystal X-ray diffraction data. The framework shows intersecting 10- and 11-ring channels running in three directions. The [Ni(1,2-pda)2]2+ complex with square planar geometry plays a structure directing role via hydrogen-bonding interactions with the framework. The twinning in the framework of SU-79 is caused by two possible connection types between the double layers in the bc-plane.
Acknowledgements
This project was supported by the National Basic Research Program of China (no. 2013CB933402), the Swedish Research Council (VR) and the Swedish Governmental Agency for Innovation Systems (VINNOVA) through the Berzelii Center EXSELENT and the Göran-Gustafsson Foundation for Nature Sciences and Medical Research. We thank the Knut & Alice Wallenberg Foundation through the project grant 3DEM-NATUR and a grant for purchasing the TEM. We also thank Beamline I19 and I11 in Diamond Light Source, UK for data collection. Jie Su thanks the post-doctoral grant from the Wenner-Gren Foundation.
Notes and references
- A. K. Cheetham, G. Férey and T. Loiseau, Angew. Chem., Int. Ed., 1999, 38, 3268 CrossRef CAS; A. Corma and M. E. Davis, ChemPhysChem, 2004, 5, 304 CrossRef; X. D. Zou, T. Conradsson, M. Klingstedt, M. S. Dadachov and M. O'Keeffe, Nature, 2005, 437, 716 CrossRef PubMed; K. E. Christensen, L. Shi, T. Conradsson, T. Z. Ren, M. S. Dadachov and X. D. Zou, J. Am. Chem. Soc., 2006, 128, 14238 CrossRef PubMed; X. Y. Ren, Y. Li, Q. H. Pan, J. H. Yu, R. R. Xu and Y. Xu, J. Am. Chem. Soc., 2011, 131, 14128 CrossRef PubMed.
- H. L. Li, M. Eddaoudi, D. A. Richardsson and O. M. Yaghi, J. Am. Chem. Soc., 1998, 120, 8567 CrossRef CAS; J. Plévert, T. M. Gentz, A. Laine, H. L. Li, V. G. Young, O. M. Yaghi and M. O'Keeffe, J. Am. Chem. Soc., 2001, 123, 12706 CrossRef; L. Beitone, T. Loiseau and G. Férey, Inorg. Chem., 2002, 41, 3962 CrossRef PubMed; Q. H. Pan, J. Y. Li, K. E. Christensen, C. Bonneau, X. Y. Ren, L. Shi, J. L. Sun, X. D. Zou, G. H. Li, J. H. Yu and R. R. Xu, Angew. Chem., Int. Ed., 2008, 47, 7868 CrossRef PubMed; L. Shi, C. Bonneau, Y. F. Li, J. L. Sun, H. J. Yue and X. D. Zou, Cryst. Growth Des., 2008, 8, 3695 Search PubMed; B. Guo, A. K. Inge, C. Bonneau, J. L. Sun, K. E. Christensen, Z. Y. Yuan and X. D. Zou, Inorg. Chem., 2011, 50, 201 CrossRef PubMed; J. Su, Y. X. Wang, Z. M. Wang, F. H. Liao and J. H. Lin, Inorg. Chem., 2010, 49, 9765 CrossRef PubMed; L. Q. Tang, X. Y. Ren, A. K. Inge, T. Willhammar, D. Grüner, J. H. Yu and X. D. Zou, Cryst. Growth Des., 2012, 12, 3714 Search PubMed; A. K. Inge, J. L. Sun, F. Moraga, B. Guo and X. D. Zou, CrystEngComm, 2012, 14, 5465 RSC.
- H. L. Li and O. M. Yaghi, J. Am. Chem. Soc., 1998, 120, 10569 CrossRef CAS; T. Conradsson, M. S. Dadachov and X. D. Zou, Microporous Mesoporous Mater., 2000, 41, 183 CrossRef; Y. Mathieu, J.-L. Paillaud, P. Caullet and N. Bats, Microporous Mesoporous Mater., 2004, 75, 13 CrossRef PubMed.
- R. Jones, J. Chen, J. Thomas, A. George, M. Hursthouse, R. Xu, S. Lu and G. Y. Yang, Chem. Mater., 1992, 4, 808 CrossRef CAS; H. L. Li, M. Eddaoudi and O. M. Yaghi, Angew. Chem., Int. Ed., 1999, 38, 653 CrossRef; Y. M. Zhou, H. G. Zhu, Z. X. Chen, M. Q. Chen, Y. Xu, H. Y. Zhang and D. Y. Zhao, Angew. Chem., Int. Ed., 2001, 40, 2166 CrossRef; X. H. Bu, P. Y. Feng and G. D. Stucky, Chem. Mater., 2000, 12, 1505 CrossRef; K. Sun, M. S. Dadachov, T. Conradsson and X. D. Zou, Acta Crystallogr., Sect. C: Cryst. Struct. Commun., 2000, 56, 1092 Search PubMed; Z. E. Lin, J. Zhang, S. T. Zheng and G. Y. Yang, Microporous Mesoporous Mater., 2004, 74, 205 CrossRef PubMed; M. E. Medina, M. Iglesias, N. Snejko, E. Gutiérrez-Puebla and M. A. Monge, Chem. Mater., 2004, 16, 594 CrossRef; M. P. Attfield, Y. Al-Ebini, R. G. Pritchard, E. M. Andrews, R. J. Charlesworth, W. Hung, B. J. Masheder and D. S. Royal, Chem. Mater., 2007, 19, 316 CrossRef.
- M. E. Fleet, Acta Crystallogr., Sect. C: Cryst. Struct. Commun., 1990, 46, 1202 CrossRef CAS; Y. Xu, L. Cheng and W. You, Inorg. Chem., 2006, 45, 7705 CrossRef PubMed; C. Bonneau, J. L. Sun, R. Sanchez-Smith, B. Guo, D. Zhang, A. K. Inge, M. Eden and X. D. Zou, Inorg. Chem., 2009, 48, 9962 CrossRef PubMed; A. K. Inge, M. V. Peskov, J. L. Sun and X. D. Zou, Cryst. Growth Des., 2012, 12, 369 Search PubMed; K. E. Christensen, C. Bonneau, M. Gustafsson, L. Shi, J. L. Sun, J. Grins, K. Jansson, I. Sbille, B. L. Su and X. D. Zou, J. Am. Chem. Soc., 2008, 130, 3758 CrossRef PubMed; A. K. Inge, S. L. Huang, H. Chen, F. Moraga, J. L. Sun and X. D. Zou, Cryst. Growth Des., 2012, 12, 4853 Search PubMed; S. L. Huang, A. K. Inge, S. H. Yang, K. E. Christensen, X. D. Zou and J. L. Sun, Dalton Trans., 2012, 41, 12358 RSC.
- Z. E. Lin, J. Zhang, J. T. Zhao, S. T. Zheng, C. Y. Pan, G. M. Wang and G. Y. Yang, Angew. Chem., Int. Ed., 2005, 44, 6881 CrossRef CAS PubMed.
- H. X. Zhang, J. Zhang, S. T. Zheng and G. Y. Yang, Inorg. Chem., 2003, 42, 6595 CrossRef CAS PubMed.
- Q. H. Pan, J. Y. Li, X. Y. Ren, Z. P. Wang, G. H. Li, J. H. Yu and R. R. Xu, Chem. Mater., 2008, 20, 370 CrossRef CAS.
- Y. D. Han, Y. Li, J. H. Yu and R. R. Xu, Angew. Chem., Int. Ed., 2011, 123, 3059 CrossRef.
- S. L. Huang, K. E. Christensen, M. V. Peskov, S. H. Yang, K. Li, X. D. Zou and J. L. Sun, Inorg. Chem., 2011, 50, 9921 CrossRef CAS PubMed.
- W. Luo, W. Q. Mu, X. Zhang, X. Zhang, Y. Y. Pu, Q. Y. Zhu and J. Dai, Inorg. Chem., 2012, 51, 1489 CrossRef CAS PubMed.
- H. J. Yue, M. V. Peskov, J. L. Sun and X. D. Zou, Inorg. Chem., 2012, 51, 12260 CrossRef CAS PubMed.
- G. M. Sheldrick, Acta Crystallogr., Sect. A: Fundam. Crystallogr., 2008, 64, 112 CrossRef CAS PubMed.
- W. Wan, J. L. Sun, J. Su, S. Hovmöller and X. D. Zou, J. Appl. Crystallogr., 2013, 46, 1863 CAS.
- D. L. Zhang, P. Oleynikov, S. Hovmöller and X. D. Zou, Z. Kristallogr., 2010, 225, 94 CrossRef CAS.
Footnote |
† Electronic supplementary information (ESI) available: Chemical formula determination, TEM image, IR spectrum, TG curve, UV-Vis diffuse-reflection spectrum, XPS spectrum of SU-79, tables of crystallographic information and crystallographic information files in CIF format. CCDC 970381. For ESI and crystallographic data in CIF or other electronic format see DOI: 10.1039/c3qi00088e |
|
This journal is © the Partner Organisations 2014 |
Click here to see how this site uses Cookies. View our privacy policy here.