DOI:
10.1039/C4QO00005F
(Research Article)
Org. Chem. Front., 2014,
1, 284-288
P(O)R2 directed Pd(II)-catalyzed C(sp2)–H acylation†
Received
7th January 2014
, Accepted 17th February 2014
First published on 5th March 2014
Abstract
A novel method for the Pd(II)-catalyzed C–H acylation of 2-phosphorylbiphenyl with α-oxocarboxylic acids, aldehydes, alcohols and toluene is described. This reaction provides efficient access to various substituted 2′-phosphorylbiphenyl-2-acyl compounds.
Within the field of organic chemistry, the carbonyl moiety is central to many broadly used synthetic modifications and fragment coupling steps.1 Moreover, many aryl ketones are also key functionalities found in natural products, medicinally relevant molecules, and functional materials.2 Therefore, the synthesis of various aryl ketones has raised continuous interest from the chemistry community. Generally, the preparation of aryl ketones mainly relies on the Friedel–Crafts acylation of aromatic compounds, however the limited functional group tolerance and large amounts of waste restrict its applications greatly.3 In many cases, aryl ketones can also be obtained from the corresponding secondary alcohols by oxidation with chromium reagents4 or from alkenes and alkynes by Wacker oxidation.5 Recently, transition-metal-catalyzed decarboxylative cross-coupling reactions using aryl carboxylic acids as coupling partners have emerged as a novel strategy and have been successfully applied to the construction of aryl ketones.6 In particular, combining these discoveries with the transition-metal catalyzed ortho-directed C–H functionalization,7 a new pathway for the palladium-catalyzed directed acylation of unactivated arenes with α-oxocarboxylic acids, aldehydes, alcohols and aryl methanes via ortho-directed C–H bond activation and functionalization has been recently reported.8 These methods provide a more simple and efficient approach for the preparation of aryl ketones. Furthermore, the procedures involved are environmentally friendly. In the last year, several R2(O)P-directed C–H activations have attracted significant attention.9 Our group has also disclosed a series of R2(O)P-directed Pd-catalyzed C–H functionalizations involving olefination, hydroxylation and arylation through a seven-membered cyclopalladium pretransition state.10 Based on these positive results, we proposed an efficient approach for the synthesis of 2-phosphorylbiphenyl ketones by palladium-catalyzed C–H acylation with α-oxocarboxylic acids, aldehydes, alcohols and aryl methanes (Scheme 1). In contrast to previous examples of various directing groups that guide selective C–H activation, the R2(O)P group not only acts as the directing group, but also serves to the construction of the P,O-ligands. Furthermore, these compounds could also be converted into other diversified phosphorus ligand by appropriate carbonyl group transformation.
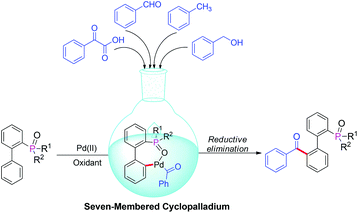 |
| Scheme 1 Pd(II)-catalyzed R2(O)P-directed C–H acylation. | |
In our initial investigation, we chose 2-(tert-butyl(phenyl)phosphoryl)biphenyl and phenylglyoxylic acid as the model substrates in the presence of Pd(OAc)2 (10 mol%) and K2S2O8 (2.5 equiv.) in CH3CN at 100 °C. To our delight, the desired product (2a) was obtained in 65% yield (Table 1, entry 1). Further solvent screening indicated that CH3CN was still the best solvent (Table 1, entries 1–5). Interestingly, when we used other solvents such as CH3NO2, diglyme and DME at 100 °C, no product 2a was observed. If the temperature was decreased to 60 °C, the product was obtained in lower yields (Table 1, entries 3–5). Subsequently, we investigated the effect of other oxidants, including (NH4)2S2O8, oxone, Ag2CO3, Ag2O, and the results showed that K2S2O8 was the best choice, while Ag2CO3 and Ag2O were completely ineffective (Table 1, entries 1, 6–9). Further studies showed that Pd(TFA)2, Pd(NO3)2 and Pd(acac)2 could also catalyze the reaction while PdCl2 gave only trace amounts of the desired product 2a (Table 1, entries 10–13). When the temperature was increased to 120 °C and 130 °C, the yield of 2a was improved to 71% and 72% respectively (Table 1, entries 14–15). Increasing or reducing the amount of K2S2O8 did not result in any significant variation in the yield of 2a (Table 1, entries 16–17). When the loading of Pd(OAc)2 was decreased to 5 mol%, the yield of 2a also decreased synchronously (Table 1, entry 18). Moreover, the control experiment showed that Pd(OAc)2 was necessary for the reaction (Table 1, entry 19). Thus, we decided to set 2.5 equiv. K2S2O8 in the presence of 10 mol% Pd(OAc)2 at 130 °C as our standard conditions (Table 1, entry 15).
Table 1 Reaction conditions screeninga
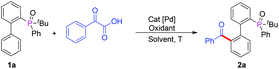
|
Entry |
Cat. (mol%) |
Oxidant (equiv.) |
Solvent |
T (°C) |
Yieldb [%] |
Reaction conditions: 1a (0.3 mmol), phenylglyoxylic acid (0.6 mmol), catalyst, and oxidant in dry CH3CN (3 mL) for 3 h under air atmosphere unless otherwise noted.
Isolated yield.
12 h.
|
1 |
Pd(TFA)2 (10) |
K2S2O8 (2.5) |
CH3CN |
100 |
65 |
2 |
Pd(OAc)2 (10) |
K2S2O8 (2.5) |
DCE |
100 |
n.r. |
3 |
Pd(OAc)2 (10) |
K2S2O8 (2.5) |
CH3NO2 |
60 |
22c |
4 |
Pd(OAc)2 (10) |
K2S2O8 (2.5) |
Diglyme |
60 |
35c |
5 |
Pd(OAc)2 (10) |
K2S2O8 (2.5) |
DME |
60 |
29c |
6 |
Pd(OAc)2 (10) |
(NH4)2S2O8 (2.5) |
CH3CN |
100 |
<5 |
7 |
Pd(OAc)2 (10) |
Oxone (2.5) |
CH3CN |
100 |
55 |
8 |
Pd(OAc)2 (10) |
Ag2CO3 (2.5) |
CH3CN |
100 |
n.r. |
9 |
Pd(OAc)2 (10) |
Ag2O (2.5) |
CH3CN |
100 |
n.r. |
10 |
Pd(TFA)2 (10) |
K2S2O8 (2.5) |
CH3CN |
100 |
22 |
11 |
Pd(TFA)2 (10) |
K2S2O8 (2.5) |
CH3CN |
100 |
38 |
12 |
PdCl2 (10) |
K2S2O8 (2.5) |
CH3CN |
100 |
<5 |
13 |
Pd(acac)2 (10) |
K2S2O8 (2.5) |
CH3CN |
100 |
29 |
14 |
Pd(OAc)2 (10) |
K2S2O8 (2.5) |
CH3CN |
120 |
71 |
15 |
Pd(OAc)2 (10) |
K2S2O8 (2.5) |
CH3CN |
130 |
72 |
16 |
Pd(OAc)2 (10) |
K2S2O8 (2.5) |
CH3CN |
120 |
68 |
17 |
Pd(OAc)2 (10) |
K2S2O8 (2.5) |
CH3CN |
120 |
62 |
18 |
Pd(OAc)2 (5) |
K2S2O8 (2.5) |
CH3CN |
120 |
58 |
19 |
|
K2S2O8 (2.5) |
CH3CN |
120 |
n.r. |
With the optimized reaction conditions in hand, we first examined the scope of substrates by changing the phosphate directing group (Table 2). In addition to 2-(tert-butyl(phenyl)phosphoryl)biphenyl, 2-(diisopropylphosphoryl)biphenyl, 2-(di-tert-butylphosphoryl)biphenyl, 2-(dicyclophosphoryl)biphenyl and 2-(diphenylphosphoryl)biphenyl were also compatible with this reaction and afforded the desired products in moderate yields (Table 2, 2a–2e). However, when diethyl biphenyl-2-ylphosphonate was used, no product was detected (2f). Furthermore, other phosphates such as triphenylphosphine oxide, naphthyl diphenylphosphine, styrylphosphine oxide and phenethylphosphine oxide did not afford any product (2g–2j). These results illustrated that the seven-membered cyclopalladium pretransition state may play a critical role in this transformation. Next, we investigated the scope of various substituted α-oxocarboxylic acids and 2-(tert-butyl(phenyl)phosphoryl)biphenyl derivatives. The steric effect and electronic effect were obvious in the reactions. When the methyl group was located on the para-position of tert-butyl(phenyl)phosphine oxide, a higher yield of 2l was obtained with respect to the case when the methyl group was located on the ortho-position of 2m. Furthermore, biphenyls possessing electron-donating groups such as 2k and 2l gave higher yields than those with electron-withdrawing groups (2n). With regard to the phenylglyoxylic acids, substituents such as methyl-, methoxyl-, chloro-, bromo- and trifluoromethyl- at the para- or meta-position of the α-oxocarboxylic acids were well tolerated and afforded the corresponding ketones in moderate to good yields and the electronic effect was insignificant (2o–2u). However, when the substituent was at the ortho-position, the electronic effect was evident and the yield of product (2v) decreased significantly. α-Keto acids with a naphthyl moiety also participated in the reaction and provided the product in moderate yield (2o). Unfortunately, couplings with alkylglyoxylic acids did not give the desired products. It should be noted that the reaction gave the monoacylation products selectively in all cases.
Table 2 Pd(II)-catalyzed C–H acylation of various substratesa,b
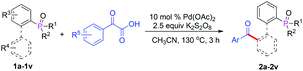
|
All the reactions were carried out in the presence of 0.3 mmol of 1a–1t, in 3 mL CH3CN at 130 °C.
Isolated yield.
|
|
Some reports of the acylation with alcohols8h,u,w also encouraged us to carry out our reactions using cheaper and readily available benzyl alcohols. Firstly, we chose 2-(tert-butyl(phenyl)phosphoryl)biphenyl and benzyl alcohol as our template substrates to proceed with the acylation under the previously established standard conditions (Table 1, entry 15). However, only trace amounts of product were obtained. This result urged us to screen different oxidants and solvents again, and the desired product 2a was obtained in moderate yield by using TBHP as oxidant and DCE as solvent (Table S1,† entries 2–7). Bisacylated compound 3a was also observed. The catalyst screening showed that Pd(TFA)2 was the best choice and the yield of acylated product was improved to 70% (Table S1,† entries 8–10). Reducing the temperature was very helpful and the acylated product was obtained in 80% yield with a 10
:
1 ratio of 2a and 3a at 60 °C (Table S1,† entries 11–12). Then the direct acylation of biphenyl with different directing groups was investigated with benzyl alcohol and the corresponding products were obtained in moderate yields with good regioselectivity (Table 3, 2b–2e). The examination of different substituted 2-(tert-butyl(phenyl)phosphoryl)biphenyl derivatives indicated that the electronic and steric effects are very evident (Table 2, 2f–2i), resulting in 2h and 2i being obtained in low yields. The electron-donating groups can increase the reactivity of 2-(tert-butyl(phenyl)phosphoryl)biphenyl. Next, we evaluated the scope of different kinds of primary alcohols. To our delight, the reactions with benzylic alcohols bearing electron-donating groups and electron-withdrawing groups on the aromatic ring proceeded to give the desired products in good yields with a small amount of the bisacylated compounds and the steric effect was also negligible (Table 3, 2j–2r). It is very interesting that aliphatic alcohols such as ethyl alcohol were also compatible with this reaction in spite of the yield of 2s being relatively low.
Table 3 Pd(II)-catalyzed C–H acylation of alcoholsa,b
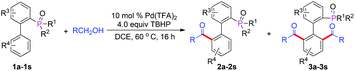
|
All the reactions were carried out in the presence of 0.3 mmol of 1a–1s in 1.5 mL DCE at 60 °C under air atmosphere.
Isolated yield.
|
|
Inspired by this impressive progress, we further selected toluene and benzaldehyde as acylation reagents and expanded the wide application of acylations. As we expected, the acylated reactions readily occurred and the monoacylated and bisacylated products were obtained in good yields with 11
:
1 and 4
:
1 ratios of 2a and 3a respectively (Scheme 2).
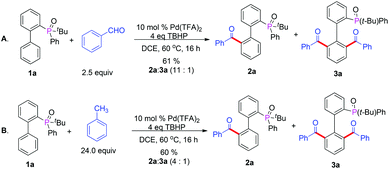 |
| Scheme 2 Pd-catalyzed acylation of 2-phosphorylbiphenyl with toluene and benzaldehyde. | |
It is well known that the carbonyl moiety is a very important synthon and can be transformed into different functional groups under appropriate conditions. In order to show the utility of our chemistry, we selected several acylated products and made derivatizations (Scheme 3). By using 2a, we could transform the carbonyl group into olefin 4a in 81% yield by Wittig reaction11 and alcohol 4b in 51% yield by lithium chloride hydrogen reduction.12 In addition, we used 2b as starting material, which was converted into benzophenone oxime 4c in 72% yield by treatment with hydroxylamine hydrochloride.13 We also obtained the tertiary alcohol 4d in 61% yield by the reaction of the ketone with an aryl Grignard regent.14
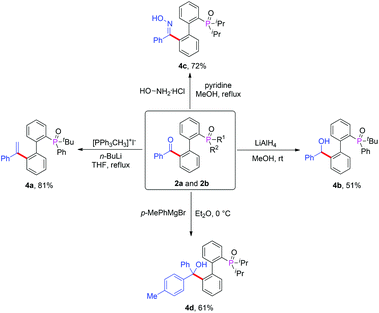 |
| Scheme 3 Transformations of acylated products into other compounds. | |
Conclusions
In conclusion, we have developed a novel R2(O)P-directed Pd(II)-catalyzed C–H acylation to synthesise various substituted 2′-phosphorylbiphenyl-2-acyl compounds. This method provides a simple and efficient pathway for the preparation of diverse biaryl ketones. Notably, we simultaneously achieved the reaction using different acylation reagents, which offers the possibility to select the conditions according to the properties of products and substrates.
Acknowledgements
We are grateful to the NSFC (Nos. 21272100), the Program for Changjiang Scholars and Innovative Research Team in University (PCSIRT: IRT1138) and the Program for New Century Excellent Talents in University (NCET-11-0215 and lzujbky-2013-k07) for financial support.
Notes and references
- For selected reviews see:
(a) E. Vedejs and C. F. March, J. Am. Chem. Soc., 1990, 112, 3905 CrossRef CAS;
(b) K. Maruyama and T. Katagiri, J. Phys. Org. Chem., 1989, 2, 205 CrossRef CAS.
- For selected reviews see:
(a) Y. Deng, Y. W. Chin, H. Chai, W. J. Keller and A. D. Kinghorn, J. Nat. Prod., 2007, 70, 2049 CrossRef CAS PubMed;
(b) K. R. Romines, G. A. Freeman, L. T. Schaller, J. R. Coman, S. S. Gonzales, J. H. Tidwell, C. W. Andrews, D. K. Stammers, R. J. Hazen, R. G. Ferris, S. A. Short, J. H. Chan and L. R. Boone, J. Med. Chem., 2006, 49, 727 CrossRef CAS PubMed.
-
(a)
G. Sartori and R. Maggi, Advances in Friedel-Crafts Acylation Reactions, CRC Press, FL, 2010 Search PubMed;
(b)
G. A. Olah, Friedel–Crafts Chemistry, Wiley, New York, 1973 Search PubMed.
-
M. Fernanderz and G. Tojo, in Oxidation of Alcohols to Aldehydes and Ketones: A Guide to Current Common Practice, ed. E. Tojo, Springer, New York, 2006 Search PubMed.
- G. J. Brink, I. W. C. E. Wrends, G. Papadogianakis and R. A. Sheldon, Chem. Commun., 1998, 2359 RSC.
-
(a) J. Hu, N. Zhao, B. Yang, G. Wang, L. N. Guo, Y. M. Liang and S. D. Yang, Chem.–Eur. J., 2011, 17, 5516 CrossRef CAS PubMed;
(b) N. Rodriguez and L. J. Goossen, Chem. Soc. Rev., 2011, 40, 5030 RSC;
(c) J. T. Mohr, T. Nishimata, D. C. Behenna and B. M. Stoltz, J. Am. Chem. Soc., 2006, 128, 11348 CrossRef CAS PubMed;
(d) A. G. Myers, D. Tanaka and M. R. Mannion, J. Am. Chem. Soc., 2002, 124, 11250 CrossRef CAS PubMed;
(e) P. Hu, J. Kan, W. P. Su and M. C. Hong, Org. Lett., 2009, 11, 2341 CrossRef CAS PubMed;
(f) D. Tanaka and A. G. Myers, Org. Lett., 2004, 6, 433 CrossRef CAS PubMed;
(g) W. Y. Yu, W. N. Sit, Z. Y. Zhou and A. S.-C. Chan, Org. Lett., 2009, 11, 3174 CrossRef CAS PubMed;
(h) C. Y. Wang, S. Rakshit and F. Glorious, J. Am. Chem. Soc., 2010, 132, 14006 CrossRef CAS PubMed.
- For selected reviews see:
(a)
G. Dyker, Handbook of C-H Transformation, 2005, Wiley-VCH Search PubMed;
(b) T. W. Lyons and M. S. Sanford, Chem. Rev., 2010, 110, 1147 CrossRef CAS PubMed;
(c) P. B. Arockiam, C. Bruneau and P. H. Dixneuf, Chem. Rev., 2012, 112, 5879 CrossRef CAS PubMed;
(d) J. Wencel-Delord, T. Dröge, F. Liu and F. Glorius, Chem. Soc. Rev., 2011, 40, 4740 RSC.
-
(a) P. Fang, M. Z. Li and H. B. Ge, J. Am. Chem. Soc., 2010, 132, 11898 CrossRef CAS PubMed;
(b) J. M. Miao and H. B. Ge, Org. Lett., 2013, 15, 2930 CrossRef CAS PubMed;
(c) M. Z. Li and H. B. Ge, Org. Lett., 2010, 12, 3463 Search PubMed;
(d) M. Y. Kim, A. Park, S. Sharma, A. Kim, E. Park, J. H. Kwak, Y. H. Jung and I. S. Kim, Chem. Commun., 2013, 49, 925 RSC;
(e) S. Sharma, J. Park, E. Park, A. Kim, M. Kim, J.
H. Kwak, Y. H. Jung and I. S. Kim, Adv. Synth. Catal., 2013, 355, 332 CAS;
(f) J. Park, E. Park, A. Kim, Y. Lee, K.-W. Chi, J. H. Kwark, Y. H. Jung and I. S. Kim, Org. Lett., 2011, 13, 4390 CrossRef CAS PubMed;
(g) J. Park, M. Kim, S. Sharma, E. Park, A. Kim, S. H. Lee, J. H. Kwak, Y. H. Jung and I. S. Kim, Chem. Commun., 2013, 49, 1654 RSC;
(h) F. Xiao, Q. Shuai, F. Zhao, Q. Basle, G. Deng and C.-J. Li, Org. Lett., 2011, 13, 1614 CrossRef CAS PubMed;
(i) Q. Basle, J. Bidange, Q. Shuai and C.-J. Li, Adv. Synth. Catal., 2010, 352, 1145 CrossRef;
(j) C.-W. Chan, Z. Y. Zhou and W.-Y. Yu, Adv. Synth. Catal., 2011, 353, 2999 CrossRef CAS;
(k) C.-W. Chan, Z. Y. Zhou, A. S. C. Chan and W.-Y. Yu, Org. Lett., 2010, 12, 3926 CrossRef CAS PubMed;
(l) Z. Y. Yang, X. Chen, J. Liu, Q. W. Gui, K. Xie, M. M. Li and Z. Tan, Chem. Commun., 2013, 49, 1560 RSC;
(m) H. Wang, L.-N. Guo and X.-H. Duan, Org. Lett., 2012, 14, 4358 CrossRef CAS PubMed;
(n) F. Xiong, C. Qian, D. Lin, W. Zeng and X. X. Lu, Org. Lett., 2013, 15, 5444 CrossRef CAS PubMed;
(o) X. Jia, S. H. Zhang, W. H. Wang, F. Luo and J. Cheng, Org. Lett., 2009, 11, 3120 CrossRef CAS PubMed;
(p) S. Guin, S. K. Rout, A. Banerjee, S. Nandi and B. K. Patel, Org. Lett., 2012, 14, 5294 CrossRef CAS PubMed;
(q) H. J. Li, P. H. Li, Q. Zhao and L. Wang, Chem. Commun., 2013, 49, 9170 RSC;
(r) Z. Y. Li, D. D. Li and G. W. Wang, J. Org. Chem., 2013, 78, 10414 CrossRef CAS PubMed;
(s) Y. Wu, B. Li, F. Mao, X. S. Li and F. Y. Kwong, Org. Lett., 2011, 13, 3258 CrossRef CAS PubMed;
(t) C. L. Li, L. Wang, P. H. Li and W. Zhou, Chem.–Eur. J., 2011, 17, 10208 CrossRef CAS PubMed;
(u) Y. Yuan, D. T. Chen and X. W. Wang, Adv. Synth. Catal., 2011, 353, 3373 CrossRef CAS;
(v) C. D. Pan, H. M. Jin, X. Liu, Y. X. Cheng and C. J. Zhu, Chem. Commun., 2013, 49, 2933 RSC;
(w) Q. P. Ding, H. F. Ji, C. Q. Ye, J. J. Wang, J. Y. Wang, L. Y. Zhou and Y. Y. Peng, Tetrahedron, 2013, 69, 8661 CrossRef CAS PubMed;
(x) Z. W. Yin and P. P. Sun, J. Org. Chem., 2012, 77, 11339 CrossRef CAS PubMed.
-
(a) X. Meng and S. Kim, Org. Lett., 2013, 15, 1910 CrossRef CAS PubMed;
(b) L. Y. Chan, S. Kim, T. Ryu and P. H. Lee, Chem. Commun., 2013, 49, 4682 RSC;
(c) L. Chan, L. Cheong and S. Kim, Org. Lett., 2013, 15, 2186 CrossRef CAS PubMed;
(d) J. Seo, Y. Park, I. Jeon, T. Ryu, S. Park and P. H. Lee, Org. Lett., 2013, 15, 3358 CrossRef CAS PubMed;
(e) B. C. Chary, S. Kim, Y. Park, J. Kim and P. H. Lee, Org. Lett., 2013, 15, 2692 CrossRef CAS PubMed;
(f) Y. Unoh, Y. Hashimoto, D. Takeda, K. Hirano, T. Satoh and M. Miura, Org. Lett., 2013, 15, 3258 CrossRef CAS PubMed;
(g) L. Y. Chan, X. J. Meng and S. Kim, J. Org. Chem., 2013, 78, 8826 CrossRef CAS PubMed;
(h) D. Zhao, C. Nimphius, M. Lindale and F. Glorius, Org. Lett., 2013, 15, 4504 CrossRef CAS PubMed;
(i) W. H. Jeon, T. S. Lee, E. J. Kim, B. Moon and J. Kang, Tetrahedron, 2013, 69, 5152 CrossRef CAS PubMed;
(j) M. Itoh, Y. Hashimoto, K. Hirano, T. Satoh and M. Miura, J. Org. Chem., 2013, 78, 8098 CrossRef CAS PubMed.
-
(a) H. L. Wang, R. B. Hu, H. Zhang, A. X. Zhou and S.-D. Yang, Org. Lett., 2013, 15, 5302 CrossRef CAS PubMed;
(b) H. Y. Zhang, H. M. Yi, G. W. Wang, B. Yang and S.-D. Yang, Org. Lett., 2013, 15, 6169 Search PubMed;
(c) R. B. Hu, H. Zhang, X. Y. Zhang and S. D. Yang, Chem. Commun., 2014, 50, 2193 RSC.
- X. Wang, Y. F. Chen, L. F. Niu and P. F. Xu, Org. Lett., 2009, 11, 3310 CrossRef CAS PubMed.
- A. E. Finholt, A. C. A. C. Bond Jr. and H. I. Schlesinger, J. Am. Chem. Soc., 1947, 69, 1199 CrossRef CAS.
- S. B. Liu, Y. Yu and L. S. Liebeskind, Org. Lett., 2007, 9, 1947 CrossRef CAS PubMed.
- S. T. Handy, J. Org. Chem., 2006, 71, 4659 CrossRef CAS PubMed.
Footnote |
† Electronic supplementary information (ESI) available. See DOI: 10.1039/c4qo00005f |
|
This journal is © the Partner Organisations 2014 |
Click here to see how this site uses Cookies. View our privacy policy here.