DOI:
10.1039/C4QO00143E
(Research Article)
Org. Chem. Front., 2014,
1, 777-781
Copper-mediated C(sp2)–H amination using TMSN3 as a nitrogen source: redox-neutral access to primary anilines†
Received
16th May 2014
, Accepted 23rd June 2014
First published on 24th June 2014
Abstract
A Cu-mediated direct conversion of aromatic C–H to C–NH2 assisted by a chelating group was developed. The reaction employed TMSN3 as a nitrogen source under redox-neutral conditions to provide a variety of N-heterocycle-substituted primary anilines. Neither external oxidant nor additional deprotection or reduction step was required in this process.
Aromatic amines (anilines) are essential intermediates in the manufacture of agrochemicals, pharmaceuticals, dyes, pigments, and polymers.1 Primary anilines are particularly useful, because they can serve as starting materials for various aniline derivatives, such as secondary or tertiary anilines, amides, imines, amidines, and carbamates. In addition, they are precursors of many other functional groups, including halides, CN, OH, SH, CF3, etc. via diazonium salts as intermediates.2 Common routes to primary anilines are reduction of aromatic nitro compounds3 and transition-metal-catalyzed amination of aryl halides with ammonia or its surrogates.4 Recent efforts have identified that aryl boronic acids5 or substituted cyclohexenone oximes6 could also be transformed to primary anilines under metal-free or transition-metal-catalyzed conditions. In recent years, substantial achievements have been made in transition-metal-catalyzed C–H functionalization reactions due to their advantages over traditional transformations based on pre-functionalized substrates in atom-economy and step-efficiency.7 Direct conversion of aromatic C–H to C–N bonds is one of the major topics in C–H functionalization due to the great importance of anilines in organic synthesis.8 Therefore, a range of Pd-, Rh-, Ru-, or Cu-catalyzed systems employing either non-activated amino sources under oxidative conditions, such as amines, amides,9 sodium azide/nitrite,10 or pre-activated amino sources under redox-neutral conditions, such as N-chloroamines,11N-hydroxycarbamates,12O-acylhydroxylamines,13 nitrosobenzenes,14 NFSI,15 sulfonyl/acyl azides,16 and aryl/alkyl azides17 have been developed (a, Scheme 1). The corresponding secondary/tertiary aniline derivatives, azides, etc. were produced. To gain access to primary anilines via the aforementioned C–H amination methods, an extra deprotection or reduction step is unavoidable. Therefore, the direct conversion of aromatic C–H bonds to C–NH2 is highly valuable and similar reports are scarce in the literature.18
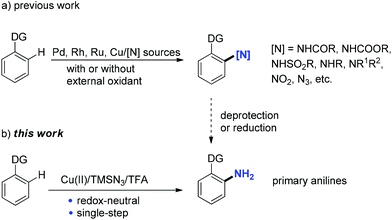 |
| Scheme 1 Chelation assisted C(sp2)–H amination. | |
Trimethylsilyl azide has been widely used in the synthesis of nitrogen-containing molecules,19 such as triazoles, tetrazoles, nitriles, azirines, and so on.20 Recently, the group of Jiao reported a copper-catalyzed C–H azidation of primary anilines with TMSN3 as an azide source directed by the free amino group.21 Monguchi and Sajiki developed a general route to primary anilines through Cu(0)-mediated reductive amination of aryl halides with TMSN3.22 Herein, we report an unprecedented copper(II)-mediated access to primary anilines using TMSN3 as an amino source in the presence of TFA via a chelation group assisted C–H activation in one pot. Neither external oxidant nor an additional deprotection or reduction step is required in this process (b, Scheme 1).
We initially studied the reaction between 2-phenylpyridine 1a and TMSN32a in the presence of copper(I) thiophene-2-carboxylate (CuTc) (30 mol%) and TfOH (1.0 equiv.) in 1,2-dichlorobenzene at 120 °C for 24 h (entry 1, Table 1). To our delight, the desired primary aniline 3a was obtained in 24% yield together with 64% of 1a recovered. Extended reaction times resulted in partial decomposition of the aminated product. TFA was superior to other acidic additives, improving the yield of 3a to 32%. Cu(TFA)2 can promote the reaction more efficiently than CuTc (36%). Only a trace amount of 3a was detected in the absence of acid, suggesting the essential role of acid in this amination process (entries 2–5). In all of the cases, the starting material 1a cannot be fully consumed, presumably as a result of strong coordination of copper with the two nitrogen atoms in 3a. As expected, the yield of 3a was improved to 88% when the loading of Cu(TFA)2 was increased to 1.0 equivalent (entry 6). Other copper salts, including Cu(OAc)2, CuCl2, Cu(OTf)2, Cu(hfacac)2, CuI, and CuBr were also screened (see ESI†). It was intriguing that when Cu(TFA)2 was replaced by Cu(OAc)2, no 3a was detectable. When CuCl2 was applied, only mono- and dichlorinated products were formed. It is obvious that the counter anion of copper is vital for product formation. Screening of solvents resulted in lower yields or no product formation (entries 9–10, and ESI†). It should be noted that NaN3 can also be used as an amino source instead of TMSN3, furnishing 1a in 38% yield (entry 11). A control reaction using Pd(TFA)2 (20 mol%) as catalyst didn't give any of the desired product. When the reaction temperature was lowered to 90 °C, this C–H amination reaction was shut down completely.
Table 1 Optimization of the reaction conditionsa
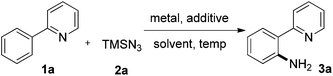
|
Entry |
Metal (equiv.) |
Additive (1.0 equiv.) |
Solvent |
Time (h) |
Yieldb (%) |
Conditions: 1a (0.20 mmol), 2a (0.4 mmol), metal salt, additive (1.0 equiv.), solvent (1.0 mL), Ar, sealed tube, 115 °C.
Yield of isolated 3a, DCB = 1,2-dichlorobenzene. CuTc = copper(I) thiophene-2-carboxylate.
Mono- and dichlorinated products were formed.
NaN3 (0.4 mmol) was used, 41% of 1a was recovered.
|
1 |
CuTc (0.3) |
TfOH |
DCB |
24 |
24 |
2 |
CuTc (0.3) |
TFA |
DCB |
24 |
32 |
3 |
CuTc (0.3) |
— |
DCB |
24 |
Trace |
4 |
CuTc (0.3) |
p-TsOH |
DCB |
24 |
18 |
5 |
Cu(TFA)2 (0.3) |
TFA |
DCB |
24 |
36 |
6
|
Cu(TFA)2 (1.0)
|
TFA
|
DCB
|
12
|
88
|
7 |
Cu(OAc)2 (1.0) |
TFA |
DCB |
24 |
N.R. |
8 |
CuCl2 (1.0) |
TFA |
DCB |
24 |
N.D.c |
9 |
Cu(TFA)2 (1.0) |
TFA |
Toluene |
12 |
47%. |
10 |
Cu(TFA)2 (1.0) |
TFA |
DMSO |
12 |
N.R. |
11 |
Cu(TFA)2 (1.0) |
TFA |
DCB |
24 |
38%d |
12 |
Pd(TFA)2 (0.2) |
TFA |
DCB |
12 |
N.R. |
Next, the scope of this C–H amination reaction was investigated under the optimal reaction conditions. 2-Phenylpyridines bearing an electron-donating Me (3b and 3c) or electron-withdrawing CF3 (3e) group in the pyridine moiety aminated smoothly. However, with a methyl group substituted at C6 of the pyridine the reaction deteriorated dramatically (34%, 3d) probably due to the steric hindrance around the pyridine nitrogen, proving its great importance in chelation with Cu. C–H amination also occurred efficiently on substrates with various substituents on different positions of the phenyl ring. Electron-donating OMe, OBn, and t-Bu (3f–h) as well as electron-withdrawing halogens (3k–l), ester (3m), ketone (3n), NO2 (3p), and CF3 (3q) groups on the para position were well tolerated, providing corresponding primary anilines in good to excellent yields. The nitrile group was less compatible with the acidic conditions, furnishing 3o in a lower yield of 44%. It is noteworthy that an alkene group in 3i also survived the reaction. These functional groups provided handles for further transformations on the scaffold. For meta-substituted substrates, amination took place at the less sterically hindered C–H bond exclusively (3r and 3s). Notably, a bipyridine substrate, 2-(pyridin-3-yl)pyridine, produced only one aminated product, 3-(pyridin-2-yl)pyridin-2-amine 3t, regioselectively in 76% yield. Besides pyridine, other heterocycles, such as pyrimidine, pyrazole, and isoquinoline, can also act as viable chelating groups in this amination reaction, providing the corresponding products 3u–w in moderate to good yields. Unlike other chelation assisted C(sp2)–H functionalization reactions where difunctionalization is generally an inevitable side-reaction, no diamination products were observed in any of the cases. Recently, Jiao reported a Pd-catalyzed tandem C–H azidation and N–N bond formation by reacting 2-arylpyridines with sodium azide in the presence of oxidants.23 However, neither 2-(2-azidophenyl)pyridines as reaction intermediates nor pyrido[1,2-b]indazoles were detected in the current Cu-mediated primary aniline synthesis (Table 2).
Table 2 Scope of C–H aminationa,b
Reaction conditions: 1 (0.2 mmol), 2a (0.4 mmol), Cu(TFA)2 (1.0 equiv.), TFA (1.0 equiv.), 1, 2-dichlorobenzene (1.0 mL), Ar, sealed tube, 115 °C.
Yield of isolated 3.
|
|
These primary aniline products containing an ortho-N-heterocycle are useful precursors in the synthesis of bidentate nitrogen ligands24 as well as bioactive agents. For example, the N-Ts protected derivative of 3a was an effective bidentate ligand in fluorination chemistry.25 The binding affinity of this class of ligands is tunable, since analogues of 3a can be accessed feasibly by applying this amination method. Pyrazole and isoquinoline substituted primary anilines 3v and 3w are intermediates in the synthesis of apoptosis inducers26 and inhibitors of reverse transcriptase,27 respectively (Scheme 2). Primary aniline 3x, a key intermediate for the synthesis of inhibitors of phosphate transport protein,28 was prepared from 1x under the standard reaction conditions in 62% isolated yield. The previous method to introduce the free NH2 in 3x involves nitration and reduction steps, which is less atom-economic and step-efficient.
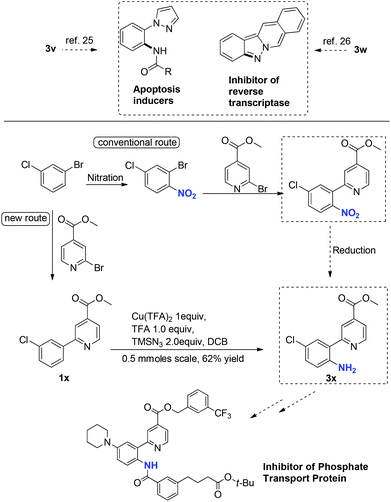 |
| Scheme 2 Application of the primary aniline products. | |
To gain insight into this C–H amination process, a competition reaction between equal amounts of 1a and deuterated 1a-d5 in one pot was carried out. The reaction was quenched at the midpoint and the product ratio between 3a and 3a-d4 was determined to be 2
:
1 by 1HNMR, suggesting that C–H bond cleavage was likely a rate-limiting step29 (Scheme 3). A possible intermediate, 2-(2-azidophenyl)pyridine 4, was synthesized and subjected to the reaction. Although the yield for the transformation of azide 4 to 3a was low under the standard conditions (32%), a reaction pathway involving azide 4 as an intermediate cannot be fully ruled out.
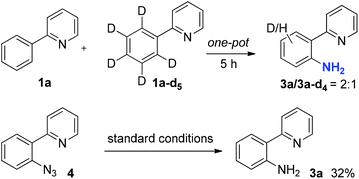 |
| Scheme 3 Mechanistic studies. | |
Although the exact mechanism of this free aniline-forming process is not clear at this stage, possible pathways are proposed in Scheme 4.30 In path A, the azido group may be transferred onto the aryl ring first via reductive elimination of a cyclometalated intermediate IA after C–H bond activation. Reduction of IB by Cu(0) in the presence of acid provides 3a. In path B, the coordinated Cu(I), generated by disproportionation of Cu(II), is oxidized to a high valent Cu(III) species31 with concurrent release of N2. After C–H bond activation and C–Cu bond formation, the cyclometalated Cu(III) intermediate IIB was formed, followed by reductive elimination. Alternatively, pathway C involving copper nitrenoid insertion into the C–Cu bond is also possible.32
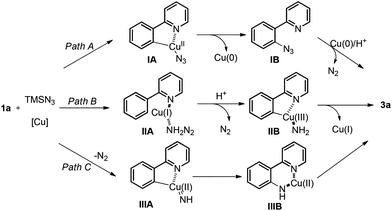 |
| Scheme 4 Plausible reaction pathways. | |
Conclusions
In summary, we have developed a copper-mediated process for the direct conversion of aromatic C–H to C–NH2 bonds. Several N-heterocycles can act as chelating groups to assist the key C–H activation step. The corresponding primary anilines containing an ortho N-heterocycle are obtained under redox neutral conditions without an extra deprotection or reduction step. A range of functional groups are tolerated. The versatility of primary anilines in chemical transformations makes this method particularly useful in diversifying N-heterocycle substituted arenes. The exploration of related transformations and further mechanistic studies are currently under way in our laboratories.
Acknowledgements
We are grateful for financial support of this work by National Science Foundation of China (21202167).
Notes and references
-
(a)
Amines: Synthesis, Properties and Applications, ed. S. A. Lawrence, Cambridge University Press, Cambridge, 2004 Search PubMed;
(b)
The Chemistry of Anilines, Parts 1 and 2, ed. Z. Rappoport, John Wiley & Sons, New York, 2007 Search PubMed;
(c)
U. Scholz and B. Schlummer, Arylamines, in Science of Synthesis, Georg Thieme Verlag, Stuttgart, 2007, vol. 31b, p. 1565 Search PubMed;
(d)
Amino Group Chemistry: From Synthesis to the Life Sciences, ed. A. Ricci, Wiley-VCH, Weinheim, 2008 Search PubMed.
-
(a) T. Sandmeyer, Ber. Dtsch. Chem. Ges., 1884, 17, 1633 CrossRef;
(b) H. H. Hodgson, Chem. Rev., 1947, 40, 251 CrossRef CAS;
(c) F. Mo, Y. Jiang, D. Qiu, Y. Zhang and J. Wang, Angew. Chem., Int. Ed., 2010, 49, 1846 CrossRef CAS PubMed;
(d) J. Dai, C. Fang, B. Xiao, J. Yi, J. Xu, Z. Liu, X. Lu, L. Liu and Y. Fu, J. Am. Chem. Soc., 2013, 135, 8436 CrossRef CAS PubMed;
(e) F. Mo, G. Dong, Y. Zhang and J. Wang, Org. Biomol. Chem., 2013, 11, 1582 RSC.
-
(a) H. U. Blaser, U. Siegrist, H. Steiner and M. Studer, Fine Chem. Heterog. Catal., 2001, 389 CAS;
(b)
T. Mallat, A. Baiker, W. Kleist and K. Koehler, Handbook of Heterogeneous Catalysis, 2nd edn, 2008, vol. 7, p. 3548 Search PubMed;
(c) H.-U. Blaser, H. Steiner and M. Studer, ChemCatChem, 2009, 1, 210 CrossRef CAS.
-
(a) Q. Shen and J. F. Hartwig, J. Am. Chem. Soc., 2006, 128, 10028 CrossRef CAS PubMed;
(b) N. Xia and M. Taillefer, Angew. Chem., Int. Ed., 2009, 48, 337 CrossRef CAS PubMed;
(c) Y. Aubin, C. Fischmeister, C. M. Thomas and J.-L. Renaud, Chem. Soc. Rev., 2010, 39, 4130 RSC;
(d) J. L. Klinkenberg and J. F. Hartwig, Angew. Chem., Int. Ed., 2011, 50, 86 CrossRef CAS PubMed.
-
(a) H. Rao, H. Fu, Y. Jiang and Y. Zhao, Angew. Chem., Int. Ed., 2009, 48, 1114 CrossRef CAS PubMed;
(b) C. Zhu, G. Li, D. H. Ess, J. R. Falck and L. Kürti, J. Am. Chem. Soc., 2012, 134, 18253 CrossRef CAS PubMed.
- W. P. Hong, A. V. Iosub and S. S. Stahl, J. Am. Chem. Soc., 2013, 135, 13664 CrossRef CAS PubMed.
- For recent reviews on C–H functionalization, see:
(a) T.-S. Mei, L. Kou, S. Ma, K. M. Engle and J.-Q. Yu, Synthesis, 2012, 1778 CAS;
(b) Z.-Z. Shi, C. Zhang, C.-H. Tang and N. Jiao, Chem. Soc. Rev., 2012, 41, 3381 RSC;
(c) T. A. Ramirez, B. G. Zhao and Y. A. Shi, Chem. Soc. Rev., 2012, 41, 931 RSC;
(d) S. R. Neufeldt and M. S. Sanford, Acc. Chem. Res., 2012, 45, 936 CrossRef CAS PubMed;
(e) L. McMurray, F. O'Hara and M. J. Gaunt, Chem. Soc. Rev., 2011, 40, 1885 RSC;
(f) C.-L. Sun, B.-F. Li and Z.-J. Shi, Chem. Rev., 2011, 111, 1293 CrossRef CAS PubMed;
(g) T. Newhouse and P. S. Baran, Angew. Chem., Int. Ed., 2011, 50, 3362 CrossRef CAS PubMed;
(h) J. Wencel-Delord, T. Droge, F. Liu and F. Glorius, Chem. Soc. Rev., 2011, 40, 4740 RSC.
-
(a) H. M. L. Davies and M. S. Long, Angew. Chem., Int. Ed., 2005, 44, 3518 CrossRef CAS PubMed;
(b) S. H. Cho, J. Y. Kim, J. Kwak and S. Chang, Chem. Soc. Rev., 2011, 40, 5068 RSC;
(c) W. C. P. Tsang, N. Zheng and S. L. Buchwald, J. Am. Chem. Soc., 2005, 127, 14560 CrossRef CAS PubMed;
(d) M. Wasa and J.-Q. Yu, J. Am. Chem. Soc., 2008, 130, 14058 CrossRef CAS PubMed;
(e) K.-H. Ng, A. S. C. Chan and W.-Y. Yo, J. Am. Chem. Soc., 2010, 132, 12862 CrossRef CAS PubMed;
(f) T. Hamada, X. Ye and S. S. Stahl, J. Am. Chem. Soc., 2008, 130, 833 CrossRef CAS PubMed;
(g) N. Matsuda, K. Hirano, T. Satoh and M. Miura, Org. Lett., 2011, 13, 2860 CrossRef CAS PubMed;
(h) G. Li, C. Jia and K. Sun, Org. Lett., 2013, 15, 5198 CrossRef CAS PubMed;
(i) M. Shang, S.-H. Zeng, S.-S. Sun, H.-X. Dai and J.-Q. Yu, Org. Lett., 2013, 15, 5286 CrossRef CAS PubMed;
(j) G. Brasche and S. L. Buchwald, Angew. Chem., Int. Ed., 2008, 47, 1932 CrossRef CAS PubMed.
- For examples of C–H amination reactions with amines and amides, see:
(a) X. Chen, X.-S. Hao, C. E. Goodhue and J.-Q. Yu, J. Am. Chem. Soc., 2006, 128, 6790 CrossRef CAS PubMed;
(b) B. Xiao, T.-J. Gong, J. Xu, Z.-J. Liu and L. Liu, J. Am. Chem. Soc., 2011, 133, 1466 CrossRef CAS PubMed;
(c) H.-Y. Thu, W.-Y. Yu and C.-M. Che, J. Am. Chem. Soc., 2006, 128, 9048 CrossRef CAS PubMed;
(d) S. H. Cho, J. Yoon and S. Chang, J. Am. Chem. Soc., 2011, 133, 5996 CrossRef CAS PubMed;
(e) Q. Shuai, G. Deng, Z. Chua, D. S. Bohle and C.-J. Li, Adv. Synth. Catal., 2010, 352, 632 CrossRef CAS;
(f) H. Zhao, M. Wang, W. Su and M. Hong, Adv. Synth. Catal., 2010, 352, 1301 CrossRef CAS;
(g) L. D. Tran, J. Roane and O. Daugulis, Angew. Chem., Int. Ed., 2013, 52, 6043 CrossRef CAS PubMed;
(h) T. Uemara, S. Imoto and N. Chatani, Chem. Lett., 2006, 35, 842 CrossRef.
-
(a) F. Xie, Z. Qi and X. Li, Angew. Chem., Int. Ed., 2013, 52, 11862 CrossRef CAS PubMed;
(b) Y. Kita, H. Tohma, M. Inagaki, K. Hatanaka and T. Yakura, Tetrahedron Lett., 1991, 32, 4321 CrossRef CAS;
(c) D. Lubriks, I. Soklovs and E. Suna, J. Am. Chem. Soc., 2012, 134, 15436 CrossRef CAS PubMed.
-
(a) T. Kanano, K. Hirano, T. Satoh and M. Miura, J. Am. Chem. Soc., 2010, 132, 6900 CrossRef PubMed;
(b) K.-H. Ng, Z. Zhou and W.-Y. Yu, Org. Lett., 2012, 14, 272 CrossRef CAS PubMed;
(c) X.-Y. Liu, P. Gao, Y.-W. Shen and Y.-M. Liang, Org. Lett., 2011, 13, 4196 CrossRef CAS PubMed;
(d) C. Grohmann, H. Wang and F. Glorius, Org. Lett., 2012, 14, 656 CrossRef CAS PubMed.
- B. Zhou, J. Du, Y. Yang, H. Feng and Y. Li, Org. Lett., 2014, 16, 592 CrossRef CAS PubMed.
-
(a) E. J. Yoo, S. Ma, T.-S. Mei, K. S. L. Chan and J.-Q. Yu, J. Am. Chem. Soc., 2011, 133, 7652 CrossRef CAS PubMed;
(b) S. Yu, B. Wan and X. Li, Org. Lett., 2013, 15, 3706 CrossRef CAS PubMed.
- B. Zhou, J. Du, Y. Yang, H. Feng and Y. Li, Org. Lett., 2013, 15, 6302 CrossRef CAS PubMed.
-
(a) K. Sun, Y. Li, T. Xiong, J. Zhang and Q. Zhang, J. Am. Chem. Soc., 2011, 133, 1694 CrossRef CAS PubMed;
(b) Z. Ni, Q. Zhang, T. Xiong, Y. Zheng, Y. Li, H. Zhang, J. Zhang and Q. Liu, Angew. Chem., Int. Ed., 2012, 51, 1244 CrossRef CAS PubMed;
(c) Á. Iglesias, R. Árno Prolvarez, Á. R. de Lera and K. Mañiz, Angew. Chem., Int. Ed., 2012, 51, 2225 CrossRef PubMed.
-
(a) J.-Y. Kim, S.-H. Park, J. Ryu, S. H. Cho, S. H. Kim and S. Chang, J. Am. Chem. Soc., 2012, 134, 9110 CrossRef CAS PubMed;
(b) M. R. Yadav, R. K. Rit and A. K. Sahoo, Org. Lett., 2013, 15, 1638 CrossRef CAS PubMed;
(c) V. S. Thirunavukkarasu, K. Raghuvanshi and L. Ackermann, Org. Lett., 2013, 15, 3286 CrossRef CAS PubMed;
(d) M. Bhanuchandra, R. Yadav, R. K. Rit, M. R. Kuram and A. K. Sahoo, Chem. Commun., 2013, 49, 5225 RSC;
(e) J. Kim, J. Kim and S. Chang, Chem. – Eur. J., 2013, 19, 7328 CrossRef CAS PubMed;
(f) J. Ryu, J. Kwak, K. Shin, D. Lee and S. Chang, J. Am. Chem. Soc., 2013, 135, 12861 CrossRef CAS PubMed;
(g) K. Shin, J. Ryu and S. Chang, Org. Lett., 2014, 16, 2022 CrossRef CAS PubMed;
(h) J. Kim and S. Chang, Angew. Chem., Int. Ed., 2014, 53, 2203 CrossRef CAS PubMed;
(i) T. Kang, Y. Kim, D. Kee, Z. Wang and S. Chang, J. Am. Chem. Soc., 2014, 136, 4141 CrossRef CAS PubMed.
-
(a) K. Shim, Y. Baek and S. Chang, Angew. Chem., Int. Ed., 2013, 52, 8031 CrossRef PubMed;
(b) J. Ryu, K. Shin, S. H. Park, J. Y. Kim and S. Chang, Angew. Chem., Int. Ed., 2012, 51, 9904 CrossRef CAS PubMed;
(c) D. Lee, K. Kim and S. Chang, J. Org. Chem., 2013, 78, 11102 CrossRef CAS PubMed.
-
(a) C. Grohmann, H. Wang and F. Glorious, Org. Lett., 2013, 15, 3014 CrossRef CAS PubMed;
(b) T. Morofuji, A. Shimizu and J. Yoshida, J. Am. Chem. Soc., 2013, 135, 5000 CrossRef CAS PubMed.
-
(a) S. Cenini, E. Gallo, A. Caselli, F. Ragaini, S. Fantauzi and C. Piangiolino, Coord. Chem. Rev., 2006, 250, 1234 CrossRef CAS PubMed;
(b) S. Bräse, C. Gil, K. Knepper and V. Zimmerman, Angew. Chem., Int. Ed., 2005, 44, 5188 CrossRef PubMed;
(c) S. Lang and J. A. Murphy, Chem. Soc. Rev., 2006, 35, 146 RSC;
(d) T. G. Driver, Org. Biomol. Chem., 2010, 8, 3831 RSC.
-
(a) C. Qin, P. Feng, Y. Ou, T. Shen, T. Wang and N. Jiao, Angew. Chem., Int. Ed., 2013, 52, 7850 CrossRef CAS PubMed;
(b) F. Chen, Y. Cui and N. Jiao, Angew. Chem., Int. Ed., 2011, 50, 7850 CrossRef PubMed;
(c) C. Qin, W. Zhou, F. Chen and Y. Ou, Angew. Chem., Int. Ed., 2011, 50, 12595 CrossRef CAS PubMed;
(d) T. Shen, T. Wang, C. Qin and N. Jiao, Angew. Chem., Int. Ed., 2013, 52, 6677 CrossRef CAS PubMed;
(e) W. Zhou, J. Xu, L. Zhang and N. Jiao, Org. Lett., 2010, 12, 2888 CrossRef CAS PubMed;
(f) C. Qin and N. Jiao, J. Am. Chem. Soc., 2010, 132, 2888 Search PubMed;
(g) T. Wang and N. Jiao, J. Am. Chem. Soc., 2013, 135, 11692 CrossRef CAS PubMed;
(h) H. Yanai and T. Taguchi, Tetrahedron Lett., 2005, 46, 8639 CrossRef CAS PubMed;
(i) T. Jin, S. Kamijo and Y. Yamamoto, Tetrahedron Lett., 2004, 45, 9435 CrossRef CAS PubMed;
(j) B. G. Reddy, K. P. Madhusudanan and Y. D. Vankar, J. Org. Chem., 2004, 69, 2630 CrossRef CAS PubMed;
(k) T. M. V. D. Pinho e Melo, C. S. J. Lopes, A. L. Cardoso and A. M. d'A. Rocha Gonsalves, Tetrahedron, 2001, 57, 6203 CrossRef CAS;
(l) D. Amantini, R. Beleggia, F. Fringuelli, F. Pizzo and L. Vaccaro, J. Org. Chem., 2004, 69, 2896 CrossRef CAS PubMed;
(m) M. J. Schulz, S. J. Coats and D. J. Hlasta, Org. Lett., 2004, 6, 3265 CrossRef CAS PubMed;
(n) S. Kamijo, T. Jin, Z. Huo and Y. Yamamoto, J. Org. Chem., 2004, 69, 2386 CrossRef CAS PubMed;
(o) M. Omura, K. Iwanami and T. Oriyama, Chem. Lett., 2007, 532 CrossRef CAS;
(p) G. D'Ambrosio, F. Fringuelli, F. Pizzo and L. Vaccaro, Green Chem., 2005, 7, 874 RSC;
(q) D. Amantini, F. Fringuelli, O. Piermatti, F. Pizzo, E. Zunino and L. Vaccaro, J. Org. Chem., 2005, 70, 6526 CrossRef CAS PubMed.
- C. Tang and N. Jiao, J. Am. Chem. Soc., 2012, 134, 18924 CrossRef CAS PubMed.
- Y. Monguchi, T. Maejima, S. Mori, T. Moegawa and H. Sajiki, Chem. – Eur. J., 2010, 16, 7372 CrossRef CAS PubMed.
- Q.-Z. Zheng, P. Feng, Y.-F. Liang and N. Jiao, Org. Lett., 2013, 15, 4262 CrossRef CAS PubMed.
-
(a) A. M. W. Cargill Thompson, S. R. Batten, J. C. Jeffery, L. H. Rees and M. D. Ward, Aust. J. Chem., 1997, 50, 109 CrossRef;
(b) C. A. Otter, S. M. Couchman, J. C. Jeffery, K. L. V. Mann, E. Psillakis and M. D. Ward, Inorg. Chim. Acta, 1998, 278, 1499 CrossRef.
-
(a) T. Furuya and T. Ritter, J. Am. Chem. Soc., 2008, 130, 10060 CrossRef CAS PubMed;
(b) T. Furuya, D. Bebnitez, E. Tkatchouk, A. E. Strom, P. Tang, W. A. Goddard III and T. Ritter, J. Am. Chem. Soc., 2010, 132, 3793 CrossRef CAS PubMed;
(c) L. Eunsung, J. M. Hooker and T. Ritter, J. Am. Chem. Soc., 2012, 134, 17456 CrossRef PubMed.
- G. Timári, T. Soós, G. Hajós, A. Messmer, J. Nacsa and J. Molnár, Bioorg. Med. Chem. Lett., 1996, 6, 1288 CrossRef.
- W. Kemnitzer, N. Sirisoma, S. Jiang, S. Kasibhatala, C. Crogan-Grcudy, B. Tseng, J. Drewe, J. Drewe and S. X. Cai, Bioorg. Med. Chem. Lett., 2010, 20, 2831 CrossRef PubMed.
-
N. Bell, C. Carreras, H.-T. Chang, D. Charmot, T. Chen, J. W. Jacobs, E. Labonte, M. R. Leadbetter, J. G. Lewis, M. Navre and N. U. S. Reich, Patent043267, April, 26 , 2012 Search PubMed.
- E. M. Simmons and J. F. Hartwig, Angew. Chem., Int. Ed., 2012, 51, 3066 CrossRef CAS PubMed.
-
(a) R. T. Gephart III and T. H. Warren, Organometallics, 2012, 31, 7728 CrossRef;
(b) Y. M. Badiel, A. Krishnaswamy, M. M. Melzer and T. H. Warren, J. Am. Chem. Soc., 2006, 128, 15056 CrossRef PubMed;
(c) Y. M. Badiei, A. Dinescu, X. Dai, R. M. Palomino, F. W. Heinenmann, T. R. Cundari and T. H. Warren, Angew. Chem., Int. Ed., 2008, 47, 9961 CrossRef CAS PubMed;
(d) S. Wiese, Y. M. Badiei, R. T. Gephart, S. Mossin, M. S. Varonka, M. M. Melzer, K. Meyer, T. R. Cundari and T. H. Warren, Angew. Chem., Int. Ed., 2010, 49, 8850 CrossRef CAS PubMed;
(e) T. Maejima, M. Ueda, J. Nakano, Y. Sawama, Y. Monguchi and H. Sajiki, J. Org. Chem., 2013, 78, 8980 CrossRef CAS PubMed.
-
(a) A. M. Suess, M. Z. Ertem, C. J. Cramer and S. S. Stahl, J. Am. Chem. Soc., 2013, 135, 9797 CrossRef CAS PubMed;
(b) L. M. Huffman and S. S. Stahl, Dalton Trans., 2011, 40, 8959 RSC;
(c) L. M. Huffman, A. Casitas, M. Font, M. Canta, M. Costas, X. Ribas and S. S. Stahl, Chem. – Eur. J., 2011, 17, 10643 CrossRef CAS;
(d) A. E. King, L. M. Huffman, A. Casitas, M. Costas, X. Ribas and S. S. Stahl, J. Am. Chem. Soc., 2010, 132, 12068 CrossRef CAS PubMed;
(e) L. M. Huffman and S. S. Stahl, J. Am. Chem. Soc., 2008, 130, 9196 CrossRef CAS PubMed.
-
(a) H. Han, S. B. Park, S. K. Kim and S. Chang, J. Org. Chem., 2008, 73, 2862 CrossRef CAS PubMed;
(b) K. M. Gillespie, E. J. Crust, R. J. Deeth and P. Scott, Chem. Commun., 2001, 785 RSC;
(c) P. Brandt, M. J. Södergren, P. G. Andersson and P.-O. Norrby, J. Am. Chem. Soc., 2000, 122, 8013 CrossRef CAS.
Footnote |
† Electronic supplementary information (ESI) available. See DOI: 10.1039/c4qo00143e |
|
This journal is © the Partner Organisations 2014 |