DOI:
10.1039/C4QO00251B
(Research Article)
Org. Chem. Front., 2014,
1, 1289-1294
1,2-Alkylarylation of activated alkenes with dual C–H bonds of arenes and alkyl halides toward polyhalo-substituted oxindoles†
Received
19th September 2014
, Accepted 6th November 2014
First published on 6th November 2014
Abstract
We describe here a new visible light facilitated radical strategy for 1,2-alkylarylation of activated alkenes with a C(sp2)–H bond of arenes and a C(sp3)–H bond of alkyl halides. This method achieves selective scission of the C(sp3)–H bond adjacent to halide atoms leading to a halo-substituted alkyl radical, and provides a new synthetic utilization of aryl halides toward polyhalo-substituted oxindoles in good to excellent yields. Moreover, the concise transformation of the products, polyhalo-substituted oxindoles, into vinyl halides and alkynyl halides was also illustrated.
Polyhalogenated hydrocarbons, a class of organic compounds with multiple substitutions of halogens, are of particular importance in organic synthesis and chemical industries (particular pharmaceuticals and materials), albeit arousing controversy for the effects of these compounds on the environment and on human and animal health.1 For example, the polychloromethyl unit is a structural feature presented in numerous bioactive natural products and pharmaceutical drugs wherein it usually provides the key component for the potent activity role in these compounds (Fig. 1).1–3 As a result, the introduction of polychloromethyl groups into known bioactive molecules remains an active area.2,3 Generally, the synthesis of polyhalogenated hydrocarbons from organohalides (often alkyl halides) is performed by thermo- or photo-initiated scission of the carbon–halogen bonds in organohalides leading to carbon-centered radicals, in which radical initiators, such as AIBN (azobis(isobutyronitrile)) and organotins (often Bu3SnH), are usually used to accomplish these transformations.4 However, methods for carbon-centered radical formation from organohalides by selectively splitting the carbon–hydrogen bond, not the carbon–halogen bond, are quite rare.5 The reason is that in organohalides the reactivity of the carbon–halogen bond is far higher than that of the carbon–hydrogen bond under thermo- or photo-initiation.
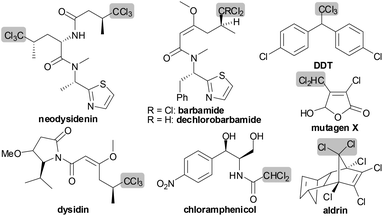 |
| Fig. 1 Important compounds with polychloro groups. | |
Recently, a new radical strategy for the oxidative cyclization of N-arylmethacrylamides with alkyl C(sp3)–H bonds to access functionalized oxindoles has been developed (Schemes 1a and 1b).6,7 We have first reported an Fe-catalyzed oxidative 1,2-alkylarylation of activated alkenes with an aryl C(sp2)–H bond and a C(sp3)–H bond adjacent to a heteroatom for building oxindoles using TBHP oxidant, which proceeds via a radical process (Scheme 1a).6a Subsequently, the Guo/Duan group,7a–d Liang group,7e Liu group7f and our group6b have independently developed other new radical cyclizations of N-arylmethacrylamides with alkyl C(sp3)–H bonds, including the C(sp3)–H bonds adjacent to an aryl,6b,7b,f a hydroxyl7a,e or dicarbonyl groups7c,d and the C(sp3)–H bonds in simple alkanes7f (Schemes 1a and 1b). However, these methods required unfriendly radical initiators including peroxides and K2S2O8 under harsh conditions (often at over 100 °C), thereby greatly restricting their applications in synthesis and industry.5b,6–8 Thus, a new mild radical strategy avoiding the use of unfriendly radical initiators for the cyclization of N-arylmethacrylamides with alkyl C(sp3)–H bonds is highly desirable. Very recently, we described a visible light catalysis strategy for the cyclization of N-arylmethacrylamides with alkyl C(sp3)–H bonds adjacent to a carbonyl group, which proceeds under mild conditions.6c Inspired by these results, we reasoned that the visible light catalysis strategy might be a better alternative for the cyclization of N-arylmethacrylamides with various alkyl C(sp3)–H bonds.9,10
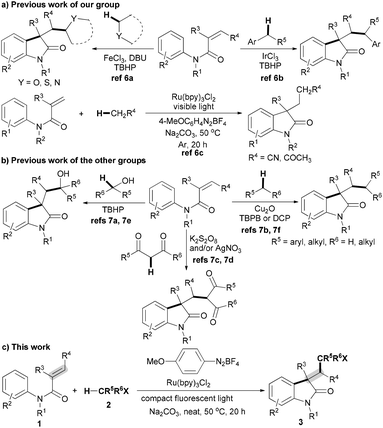 |
| Scheme 1 1,2-Alkylarylation of activated alkenes. | |
We began our investigation with the reaction between N-methyl-N-phenylmethacrylamide (1a) and dichloromethane (DCM, 2a) in the presence of visible light catalysts and 4-methoxybenzenediazonium tetrafluoroborate (Table 1). In the presence of 4-MeOC6H4N2BF4 and Na2CO3, the desired oxindole 3aa was obtained in 55% yield from substrate 1a with DCM 2a (entry 1). It has been reported that the visible light catalysis strategy could promoted the 4-MeOC6H4N2BF4-based reaction.5a,6c,10,11 As expected, treatment of substrate 1a with DCM 2a, Ru(bpy)3Cl2, 4-methoxybenzenediazonium tetrafluoroborate and 36 W compact fluorescent light increased the yield of oxindole 3aa from 55% to 96% yield (entry 2). The results showed that bases played an important role in the reaction, and Na2CO3 was the most effective (entries 2–5). While Na2CO3 as the base gave 96% yield of oxindole 3aa (entry 2), Et3N as the base decreased the yield to only 31% (entry 3), Cs2CO3 as the base to 37% (entry 4) and the absence of base to trace (entry 5). Among the reaction temperatures examined, it turned out that 50 °C was preferred for the reaction (entries 2 and 6–7). Gratifyingly, good yield was still achieved even at 2 mol% Ru(bpy)3Cl2 (entry 8). It is noteworthy that the reaction proceeds in MeCO2nBu smoothly, albeit with slightly lowering the yield (entry 9). Two other visible light photoredox catalysts, Ir(ppy)3 and Eosin Y, were found to effect the reaction (entries 1 vs. 10–11). These results also suggest that the role of both Ru and visible light is mainly to improve the reaction. Extensive screening revealed that the amount of 4-methoxyphenyldiazonium tetrafluoroborate has a fundamental influence on the reaction: the yield decreased from 96% (entry 2) to 59% at 1 equiv. diazonium salt (entry 12), and the absence of diazonium salt resulted in no detectable product 3aa (entry 13).
Table 1 Screening of optimal conditionsa
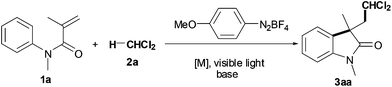
|
Entry |
[M] [mol%] |
Base |
T [°C] |
Isolated yield [%] |
Reaction conditions: 1a (0.3 mmol), 2a (15 mmol), [M], 4-MeOC6H4N2BF4 (2 equiv.) and base (2 equiv.) with 36 W compact fluorescent light for 20 h under argon atmosphere.
Without additional light.
2a (6 mmol) and MeCO2nBu (anhydrous, 0.5 mL).
4-MeOC6H4N2BF4 (1 equiv.).
Without 4-MeOC6H4N2BF4.
|
1b |
— |
Na2CO3 |
50 |
55 |
2 |
Ru(bpy)3Cl2 (5) |
Na2CO3 |
50 |
96 |
3 |
Ru(bpy)3Cl2 (5) |
Et3N |
50 |
31 |
4 |
Ru(bpy)3Cl2 (5) |
Cs2CO3 |
50 |
37 |
5 |
Ru(bpy)3Cl2 (5) |
— |
50 |
Trace |
6 |
Ru(bpy)3Cl2 (5) |
Na2CO3 |
25 |
8 |
7 |
Ru(bpy)3Cl2 (5) |
Na2CO3 |
80 |
83 |
8 |
Ru(bpy)3Cl2 (2) |
Na2CO3 |
50 |
80 |
9c |
Ru(bpy)3Cl2 (5) |
Na2CO3 |
50 |
75 |
10 |
Ir(ppy)3 (5) |
Na2CO3 |
50 |
95 |
11 |
Eosin Y (5) |
Na2CO3 |
50 |
68 |
12d |
Ru(bpy)3Cl2 (5) |
Na2CO3 |
50 |
59 |
13e |
Ru(bpy)3Cl2 (5) |
Na2CO3 |
50 |
0 |
Encouraged by these results, we next set out to examine the effect of diazonium salts (Scheme 2).11 The results demonstrated that three other aryldiazonium salts showed high reactivity, but they were less effective than 4-methoxybenzenediazonium tetrafluoroborate (Scheme 2vs. entry 2 in Table 1).
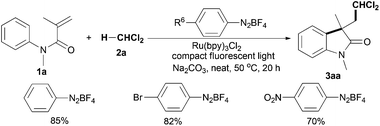 |
| Scheme 2 Screening of effect of diazonium salts. | |
With the optimal conditions in hand, we first employed N-arylacrylamides 1 to explore the scope of the above radical cyclization protocol in the presence of DCM 2a (Table 2). While analogous amides with N-Bn, N-(2-iodobenzyl) or N-Ph were found to be viable substrates for the reaction (products 3ba–3da), changing to N-Ac resulted in a lower reactivity (product 3ea). Gratifyingly, we found that a number of substituents, including alkyl, MeO, Cl, F, CF3, I and SMe groups, on the aromatic ring of the N-aryl moiety were tolerated well (products 3fa–ra), and the reactive order is as follow: para- > meta- > ortho-substituents. For example, para-Me-substituted substrate 1f afforded oxindole 3fa in 88% yield under the optimized conditions. Good yields were still achieved from substrates 1g–1i with other electron-rich groups, such as Et, n-Bu and MeO groups (products 3ga–ia). The reaction was not constrained by an electron-withdrawing CF3 group, giving product 3la in 80% yield. However, the yields of oxindoles 3ma–pa from the corresponding ortho-substituted substrates 1m–1p decreased to moderate. It was noted that meta-substituted substrates 1q or 1r gave a mixture of two regioselective oxindoles (products 3qa and 3ra). Most importantly, halo groups, I, Cl and F groups, could be tolerated, thereby facilitating additional modifications at the halogenated position (products 3ca, 3ja, 3ka, 3na, and 3oa).12 Screening disclosed that substrates 1s–1u bearing substituents, Ph, CH2OH or CH2OAc, at the 2 position of the acrylamide moiety were compatible with the optimal conditions (products 3sa–ua). Free CH2OH-substituted substrate 1t, for instance, was converted into product 3ta in 50% yield. However, substrate 1v with a hydrogen atom at the 2 position had no reactivity for the reaction (product 3va). Interestingly, N-methyl-N-phenylcinnamamide (1w), an internal alkene, could also be cyclized to oxindole 3wa in 53% yield.
Table 2 Screening scope of N-arylacrylamides (1)a
Reaction conditions: 1 (0.3 mmol), 2a (15 mmol), Ru(bpy)3Cl2 (5 mol%), 4-MeOC6H4N2BF4 (2 equiv.) and Na2CO3 (2 equiv.) with 36 W compact fluorescent light at 50 °C for 20 h under argon atmosphere.
A side de-I product 3aa was isolated in 36% yield.
|
|
As shown in Table 3, a variety alkyl halides 2 was next investigated in the presence of N-methyl-N-phenylmethacrylamide (1a), Ru(bpy)3Cl2, 4-methoxybenzenediazonium tetrafluoroborate, Na2CO3 and 36 W compact fluorescent light. Using 1,1-dichloroethane (2b), the C(sp3)–H bond adjacent to two chloride atoms was selectively cleaved, giving product 3ab in 93% yield. The results indicate that halo groups are necessary for selective scission of the C(sp3)–H bond. Indeed, both 1,1,2-trichloroethane (2c) and 1,1,2,2-tetrachloroethane (2d) were suitable substrates; moreover, the C(sp3)–H bond adjacent to dichloride atoms, not the C(sp3)–H bond adjacent to monochloride atoms, was selected to react with substrate 1a (products 3ac and 3ad). However, no reaction was observed using 1-chlorobutane (2e), a monochloro-substituted substrate (product 3ae). Gratifyingly, using 1,2,3-trichloropropane (2f) and 1,2-dichloroethane (2g) to build the corresponding products 3af and 3ag was successful in moderate yields. For chloroform (2h), good yield of the desired oxindole 3ah was also obtained under the optimal conditions.
Table 3 Screening scope of alkyl halides (2)a
Reaction conditions: 1a (0.3 mmol), 2 (15 mmol), Ru(bpy)3Cl2 (5 mol%), 4-MeOC6H4N2BF4 (2 equiv.) and Na2CO3 (2 equiv.) with 36 W compact fluorescent light at 50 °C for 20 h under argon atmosphere.
|
|
However, this reaction was dependent on the leaving nature of the halo group: both C–H bond and C–Br bond cleavage took place using alkyl bromides (products 3ai/3ai′ and 3ak/3ak′). For example, treatment of CH2Br2 (2i) with substrate 1a afforded a mixture of C–H bond cleavage and C–Br bond cleavage oxindoles 3ai and 3ai′ in 80% total yield with 1
:
1 ratio. For bromoform (2j), however, the ratio of C–H/C–Br cleavage is 2
:
1. Using bromodichloromethane (2k), however, the reactivity of the C–Br bond is higher than the C–H bond in view of the ratio of products 3ak and 3aa.
To understand the current radical reaction, the mechanisms outlined in Scheme 3 are proposed on the basis of the present results13 and the literature reports.5–11 Initially, ˙CHCl2 radical may be generated via two pathways: (1) single-electron oxidation of DCM 2a by Ru(bpy)33+˙ gives a carbocation A, followed by deprotonation leading to the ˙CHCl2 radical (pathway I), and/or (2) hydrogen abstraction of DCM 2a by a phenyl radical which was generated from the diazonium salt forms the ˙CHCl2 radical (pathway II).6c,10,11 Subsequently, addition of ˙CHCl2 radical to alkene produces radical intermediate B, followed by intramolecular cyclization of radical intermediate B with an arene which gives rise to radical intermediate C. Hydrogen atom abstraction of radical intermediate C by Ru(bpy)32+˙ takes place to yield product 3aa and Ru(bpy)33+. Notably, this photoinduced mechanism is supported by the quantum yield (Φx = 0.056).13,14
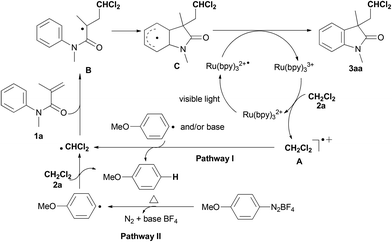 |
| Scheme 3 Possible mechanisms. | |
On the basis of the results in Table 1, the ˙CHCl2 radical can also be directly formed from the reaction between CH2Cl2 (2a) and 4-methoxyphenyl radical in the presence of a base under heating conditions. Thus, there are two key roles for the base in the present reaction: initiation of the radical reaction and neutralization of the in situ formed BF4−.
Vinyl halides or alkynyl halides are important intermediates in organic synthesis. Having established a hydrogen abstraction–cyclization tandem method for the preparation of polyhalo-substituted oxindoles 3, we finally explored their concise transformation into vinyl halides or alkynyl halides (Scheme 4).15 After a brief screening of bases, tBuOK was employed for the dehalogenation of dichloro-substituted oxindoles 3aa, 3ha, 3ia, 3ka and 3la, exclusively providing the corresponding (Z)-vinyl chlorides 4 in moderate yields (eqn (1)). Interestingly, dehalogenation of trichloro-substituted oxindole 3ah resulted in alkynyl chloride 4ah in good yield (eqn (2)). Using a mixture of bromo-substituted oxindoles 3aj and 3aj′, (Z)-vinyl bromide 4aj was formed alone in 61% yield (eqn (3)).
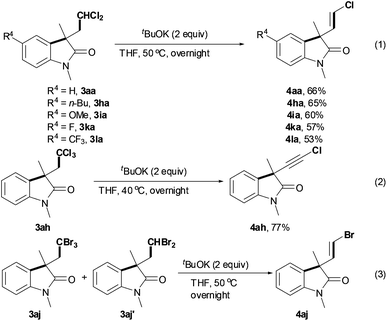 |
| Scheme 4 Utilizations of products 3. | |
In summary, selective scission of the C–H bond adjacent to halide atoms in alkyl halides under visible light photoredox catalysts-facilitated conditions has been illustrated for the synthesis of polyhalo-substituted oxindoles. Importantly, the polyhalo-substituted oxindoles could be further converted into (Z)-vinyl halides or alkynyl halides simply by treatment with tBuOK.
This research was supported by the Hunan Provincial Natural Science Foundation of China (no. 13JJ2018), Natural Science Foundation of China (no. 21172060), and Specialized Research Fund for the Doctoral Program of Higher Education (no. 20120161110041).
Notes and references
-
(a) D. J. Faulkner, Nat. Prod. Rep., 1997, 14, 259 RSC;
(b)
S. L. Neidleman and J. Geigert, Biohalogenation: Principles, Basic Roles and Applications, Wiley, New York, 1986 Search PubMed;
(c) G. W. Gribble, J. Chem. Educ., 2004, 81, 1441 CrossRef CAS;
(d) C. N. Albers, P. E. Hansen and O. S. Jacobsen, Sci. Total Environ., 2010, 408, 6223 CrossRef CAS PubMed;
(e)
N. Clarke, M. Gryndler, H.-H. Liste, R. Schroll, P. Schröder and M. Matucha, in Organic Xenobiotics and Plants: From Mode 17 of Action to Ecophysiology, ed. P. Schröer and C. D. Collins, ed. Springer, Heidelberg, 2011 Search PubMed.
-
(a) X. Fu, M. L. G. Ferreira, F. J. Schmitz and M. Kelly-Borges, J. Nat. Prod., 1998, 61, 1226 CrossRef CAS PubMed;
(b) P. N. Sitachitta, J. Rossi, M. A. Roberts, W. H. Gerwick, M. D. Fletcher and C. L. Willis, J. Am. Chem. Soc., 1998, 120, 7131 CrossRef;
(c) J. B. MacMillan, E. K. Trousdale and T. F. Molinski, Org. Lett., 2000, 2, 2721 CrossRef CAS;
(d) M. D. Unson, C. B. Rose, D. J. Faulkner, L. S. Brinen, J. R. Steiner and J. Clardy, J. Org. Chem., 1993, 58, 6336 CrossRef CAS;
(e) J. O. Orjala and W. H. Gerwick, J. Nat. Prod., 1996, 59, 427 CrossRef CAS PubMed;
(f) D. P. Galoni, E. W. Barr, C. T. Walsh, J. M. Bollinger Jr. and C. Krebs, Nat. Chem. Biol., 2007, 3, 113 CrossRef PubMed;
(g) G. W. Gribble, Chemosphere, 2003, 52, 289 CrossRef CAS;
(h) C. Wagner, M. El Omari and G. M. König, J. Nat. Prod., 2009, 72, 540 CrossRef CAS PubMed;
(i) M. Rich, R. Ritterhoff and R. Hoffmann, Ann. Intern. Med., 1950, 33, 1459 CrossRef CAS PubMed;
(j) S. J. Singer, F. Spengler, J. T. Chavez and T. Kusmierek, Carcinogenesis, 1987, 8, 745 CrossRef PubMed;
(k) K. C. Cheng, D. S. Preston, M. K. Cahill, B. Dosanjh and L. A. Singer, Proc. Natl. Acad. Sci. U. S. A., 1991, 88, 9974 CrossRef CAS;
(l) L. M. Werbel, E. F. Elslager, C. Hess and M. P. Hutt, J. Med. Chem., 1987, 30, 1943 CrossRef CAS.
- For selected reviews and papers:
(a) F. I. Luknitskii, Chem. Rev., 1975, 75, 259 CrossRef CAS;
(b) A. Butler and J. V. Walker, Chem. Rev., 1993, 93, 1937 CrossRef CAS;
(c) A. Butler, Curr. Opin. Chem. Biol., 1998, 2, 279 CrossRef CAS;
(d) P. Verhaeghe, P. Rathelot, A. Gellis, S. Rault and P. Vanelle, Tetrahedron, 2006, 62, 8173 CrossRef CAS PubMed;
(e) A. Guirado, B. Martiz and R. Andreu, Tetrahedron Lett., 2004, 45, 8523 CrossRef CAS PubMed;
(f) A. Guirado, B. Martiz, R. Andreu and D. Bautista, Tetrahedron, 2009, 65, 5958 CrossRef CAS PubMed;
(g) A. Guirado, B. Martiz, R. Andreu and D. Bautista, Tetrahedron, 2011, 67, 5811 CrossRef CAS PubMed;
(h) H.-F. Wang, P. Li, H.-F. Cui, X.-W. Wang, J.-K. Zhang, W. Liu and G. Zhao, Tetrahedron, 2011, 67, 1774 CrossRef CAS PubMed;
(i) P. Verhaeghe, N. Azas, S. Hutter, C. Castera-Ducros, M. Laget, A. Dumètre, M. Gasquet, J.-P. Reboul, S. Rault, P. Rathelot and P. Vanelle, Bioorg. Med. Chem., 2009, 17, 4313 CrossRef CAS PubMed;
(j) S. E. Brantley and T. F. Molinski, Org. Lett., 1999, 1, 2165 CrossRef CAS;
(k) H. Morimoto, G. Lu, N. Aoyama, S. Matsunaga and M. Shibasaki, J. Am. Chem. Soc., 2007, 129, 9588 CrossRef CAS PubMed;
(l) S. Beaumont, E. A. Ilardi, L. R. Monroe and A. Zakarian, J. Am. Chem. Soc., 2010, 132, 1482 CrossRef CAS PubMed.
- For selected reviews, see:
(a)
D. P. Curran, in Comprehensive Organic Synthesis, ed. B. M. Trost, I. Fleming and M. F. Semmelhack, Pergamon, Oxford, 1991, vol. 4, pp. 715–779 Search PubMed;
(b) I. Ryu, N. Sonoda and D. P. Curran, Chem. Rev., 1996, 96, 172 CrossRef PubMed;
(c)
Radicals in Organic Synthesis, ed. P. Renaud and M. P. Sibi, Wiley-VCH, Weinheim, 1st edn, 2001 Search PubMed;
(d)
Encyclopedia of Radicals in Chemistry, Biology and Materials, ed. C. Chatgilialoglu and A. Studer, Wiley, Hoboken, 2012 Search PubMed.
-
(a) Y. Liu, J.-L. Zhang, R.-J. Song and J.-H. Li, Eur. J. Org. Chem., 2014, 1177 CrossRef CAS. During our preparation of this paper, a paper has been reported on the oxidative radical cyclization of N-arylacrylamides with only CH2Cl2 or CCl4 toward chloro-containing oxindoles using FeCl2 catalyst, Ph2IOTf oxidant and Et3N base at 100 °C:
(b) M.-Z. Lu and T.-P. Loh, Org. Lett., 2014, 16, 4698 CrossRef CAS PubMed.
-
(a) W.-T. Wei, M.-B. Zhou, J.-H. Fan, W. Liu, R.-J. Song, Y. Liu, M. Hu, P. Xie and J.-H. Li, Angew. Chem., Int. Ed., 2013, 52, 3638 CrossRef CAS PubMed;
(b) M.-B. Zhou, C.-Y. Wang, R.-J. Song, Y. Liu, W.-T. Wei and J.-H. Li, Chem. Commun., 2013, 49, 10817 RSC;
(c) J.-L. Zhang, Y. Liu, R.-J. Song, G.-F. Jiang and J.-H. Li, Synlett, 2014, 1031 Search PubMed.
-
(a) Y. Meng, L.-N. Guo, H. Wang and X.-H. Duan, Chem. Commun., 2013, 49, 7540 RSC;
(b) S.-L. Zhou, L.-N. Guo, H. Wang and X.-H. Duan, Chem. – Eur. J., 2013, 19, 12970 CrossRef CAS PubMed;
(c) H. Wang, L.-N. Guo and X.-H. Duan, Chem. Commun., 2013, 49, 10370 RSC;
(d) H. Wang, L.-N. Guo and X.-H. Duan, Org. Lett., 2013, 15, 5254 CrossRef CAS PubMed;
(e) Z.-Z. Zhou, H.-L. Hua, J.-Y. Luo, Z.-S. Chen, P.-X. Zhou, X.-Y. Liu and Y.-M. Liang, Tetrahedron, 2013, 69, 10030 CrossRef CAS PubMed;
(f) Z. Li, Y. Zhang, L. Zhang and Z.-Q. Liu, Org. Lett., 2014, 16, 382 CrossRef CAS PubMed.
- For papers on other oxidative cyclizations of N-arylacrylamides:
(a) S. Jaegli, J. Dufour, H.-L. Wei, T. Piou, X.-H. Duan, J.-P. Vors, L. Neuville and J. Zhu, Org. Lett., 2010, 12, 4498 CrossRef CAS PubMed;
(b) T. Wu, X. Mu and G.-S. Liu, Angew. Chem., Int. Ed., 2011, 50, 12578 CrossRef CAS PubMed;
(c) T. Wu, H. Zhang and G.-S. Liu, Tetrahedron, 2012, 68, 5229 CrossRef CAS PubMed;
(d) H. Zhang, P. Chen and G.-S. Liu, Synlett, 2012, 2749 CAS;
(e) M.-B. Zhou, R.-J. Song, X.-H. Ouyang, Y. Liu, W.-T. Wei, G.-B. Deng and J.-H. Li, Chem. Sci., 2013, 4, 2690 RSC;
(f) Q. Zhang, F. Yang and Y.-J. Wu, Tetrahedron, 2013, 69, 4908 CrossRef CAS PubMed;
(g) Y.-M. Li, M. Sun, H.-L. Wang, Q.-P. Tian and S.-D. Yang, Angew. Chem., Int. Ed., 2013, 52, 3972 CrossRef CAS PubMed;
(h) F. Jia, K.-S. Liu, H. Xi, S.-L. Lu and Z.-P. Li, Tetrahedron Lett., 2013, 54, 6337 CrossRef CAS PubMed;
(i) X.-H. Wei, Y.-M. Li, A.-X. Zhou, T.-T. Yang and S.-D. Yang, Org. Lett., 2013, 15, 4158 CrossRef CAS PubMed;
(j) K. Matcha, R. Narayan and A. P. Antonchick, Angew. Chem., Int. Ed., 2013, 52, 7985 CrossRef CAS PubMed;
(k) H. Wang, L.-N. Guo and X.-H. Duan, Adv. Synth. Catal., 2013, 355, 2222 CrossRef CAS;
(l) S.-L. Zhou, L.-N. Guo, S. Wang and X.-H. Duan, Chem. Commun., 2014, 50, 3589 RSC;
(m) Q. Dai, J. Yu, Y. Jiang, S. Guo, H. Yang and J. Cheng, Chem. Commun., 2014, 50, 3865 RSC;
(n) J.-H. Fan, M.-B. Zhou, Y. Liu, W.-T. Wei, X.-H. Ouyang, R.-J. Song and J.-H. Li, Synlett, 2014, 657 CAS;
(o) X.-H. Ouyang, R.-J. Song and J.-H. Li, Eur. J. Org. Chem., 2014, 3395 CrossRef CAS;
(p) X. Li, X. Xu, P. Hu, X. Xiao and C. Zhou, J. Org. Chem., 2013, 78, 7343 CrossRef CAS PubMed;
(q) G. Wang, S. Wang, J. Wang, S.-Y. Chen and X.-Q. Yu, Tetrahedron, 2014, 70, 3466 CrossRef CAS PubMed;
(r) Y.-M. Li, X.-H. Wei, X.-A. Li and S.-D. Yang, Chem. Commun., 2013, 49, 11701 RSC;
(s) Y.-M. Li, Y. Shen, K.-J. Chang and S.-D. Yang, Tetrahedron Lett., 2014, 55, 2119 CrossRef CAS PubMed;
(t) T. Shen, Y. Yuan and N. Jiao, Chem. Commun., 2014, 50, 554 RSC;
(u) W. Fu, F. Xu, Y. Fu, C. Xu, S. Li and D. Zou, Eur. J. Org. Chem., 2014, 709 CrossRef CAS;
(v) J. Liu, S. Zhuang, Q. Gui, X. Chen, Z. Yang and Z. Tan, Eur. J. Org. Chem., 2014, 3196 CrossRef CAS;
(w) F. Yin and X.-S. Wang, Org. Lett., 2014, 16, 1128 CrossRef CAS PubMed;
(x) B. Zhou, W. Hou, Y. Yang, H. Feng and Y. Li, Org. Lett., 2014, 16, 1322 CrossRef CAS PubMed;
(y) W. Wei, J. Wen, D. Yang, X. Liu, M. Guo, R. Dong and H. Wang, J. Org. Chem., 2014, 79, 4225 CrossRef CAS PubMed.
- For papers on the cyclization of N-arylacrylamides using visible light photoredox catalysis:
(a) P. Xu, J. Xie, Q. Xue, C. Pan, Y. Cheng and C. Zhu, Chem. – Eur. J., 2013, 19, 14039 CrossRef CAS PubMed;
(b) J. Xie, P. Xu, H. Li, Q. Xue, H. Jin, Y. Cheng and C. Zhu, Chem. Commun., 2013, 49, 5672 RSC;
(c) W. Fu, F. Xu, Y. Fu, M. Zhu, J. Yu, C. Xu and D. Zou, J. Org. Chem., 2013, 78, 12202 CrossRef CAS PubMed;
(d) X.-J. Tang, C. S. Thomoson and W. R. Dolbier Jr., Org. Lett., 2014, 16, 4594 CrossRef CAS PubMed.
- For selected reviews on visible light photoredox catalysis, see:
(a) P. Melchiorre, Angew. Chem., Int. Ed., 2009, 48, 1360 CrossRef CAS PubMed;
(b) K. Zeitler, Angew. Chem., Int. Ed., 2009, 48, 9785 CrossRef CAS PubMed;
(c) T. P. Yoon, M. A. Ischay and J. Du, Nat. Chem., 2010, 2, 527 CrossRef CAS PubMed;
(d) J. M. R. Narayanam and C. R. J. Stephenson, Chem. Soc. Rev., 2011, 40, 102 RSC;
(e) F. Teplý, Collect. Czech. Chem. Commun., 2011, 76, 859 CrossRef;
(f) J. Xuan and W.-J. Xiao, Angew. Chem., Int. Ed., 2012, 51, 6828 CrossRef CAS PubMed;
(g) D. P. Hari and B. König, Angew. Chem., Int. Ed., 2013, 52, 4734 CrossRef CAS PubMed.
- For representative papers on the use of aryldiazonium salts in organic synthesis including the Sandmeyer reaction:
(a) T. Sandmeyer, Ber. Dtsch. Chem. Ges., 1884, 17, 1633 CrossRef;
(b) T. Sandmeyer, Ber. Dtsch. Chem. Ges., 1884, 17, 2650 CrossRef;
(c) H. H. Hodgson, Chem. Rev., 1947, 40, 251 CrossRef CAS; the Pschorr reaction:
(d) R. Pschorr, Ber. Dtsch. Chem. Ges., 1896, 29, 496 CrossRef CAS;
(e) P. H. Leake, Chem. Rev., 1956, 56, 27 CrossRef CAS; visible light photoredox catalysis:
(f) H. Cano-Yelo and A. Deronzier, J. Chem. Soc., Perkin Trans. 2, 1984, 1093 RSC;
(g) H. Cano-Yelo and A. Deronzier, J. Chem. Soc., Faraday Trans. 1, 1984, 3011 RSC;
(h) H. Cano-Yelo and A. Deronzier, Tetrahedron Lett., 1984, 25, 5517 CrossRef CAS;
(i) D. P. Hari, P. Schroll and B. König, J. Am. Chem. Soc., 2012, 134, 2958 CrossRef CAS PubMed;
(j) P. Schroll, D. P. Hari and B. König, ChemistryOpen, 2012, 1, 130 CrossRef CAS PubMed;
(k) T. Hering, D. P. Hari and B. König, J. Org. Chem., 2012, 77, 10347 CrossRef CAS PubMed;
(l) T. Xiao, X. Dong, Y. Tang and L. Zhou, Adv. Synth. Catal., 2012, 354, 3195 CrossRef CAS.
- For reviews:
(a) B. S. Jensen, CNS Drug Rev., 2002, 8, 353 CrossRef CAS PubMed;
(b) C. Marti and E. M. Carreira, Eur. J. Org. Chem., 2003, 2209 CrossRef CAS;
(c) H. Lin and S. J. Danishefsky, Angew. Chem., Int. Ed., 2003, 42, 36 CrossRef CAS;
(d) C. V. Galliford and K. A. Scheidt, Angew. Chem., Int. Ed., 2007, 46, 8748 CrossRef CAS PubMed;
(e) A. Millemaggi and R. J. K. Taylor, Eur. J. Org. Chem., 2010, 4527 CrossRef CAS;
(f) F. Zhou, Y.-L. Liu and J. Zhou, Adv. Synth. Catal., 2010, 352, 1381 CrossRef CAS;
(g) S. R. S. Rudrangi, V. K. Bontha, V. R. Manda and S. Bethi, Asian J. Res. Chem., 2011, 4, 335 Search PubMed. For selected papers:
(h) G. Deak, M. Doda, L. Gyorgy, L. Hazai and L. Sterk, J. Med. Chem., 1977, 20, 1384 CrossRef CAS;
(i) A. Numata, P. Yang, C. Takahashi, R. Fujiki, M. Nabae and E. Fujita, Chem. Pharm. Bull., 1989, 37, 648 CrossRef CAS;
(j) S. Hibino and T. Choshi, Nat. Prod. Rep., 2001, 18, 66 RSC;
(k) A. S. Ratnayake, W. Y. Yoshida, S. L. Mooberry and T. K. Hemscheidt, J. Org. Chem., 2001, 66, 8717 CrossRef CAS PubMed;
(l) M. Kuriyama, S. Tanigawa, Y. Kubo, Y. Demizu and O. Onomura, Tetrahedron: Asymmetry, 2010, 21, 1370 CrossRef CAS PubMed.
- The detailed data, including kinetic isotope effect experiments (Scheme S1†) and the quantum yield (Fig. S1†), are summarized in the ESI†.
-
(a) H. Gorner and H. J. Khun, J. Chem. Soc., Perkin Trans. 2, 1999, 2671 RSC;
(b) A. P. Pelliccioli and J. Wirz, Photochem. Photobiol. Sci., 2002, 1, 441 RSC.
-
(a) C. Hanekamp and P. A. A. Klusener, Synth. Commun., 1989, 19, 2677 CrossRef;
(b) Z.-Q. Wang, Y. Lei, M.-B. Zhou, G.-X. Chen, R.-J. Song, Y.-X. Xie and J.-H. Li, Org. Lett., 2011, 13, 14 CrossRef CAS PubMed;
(c) R. Bejot, S. Tisserand, L. M. Reddy, D. K. Barma, R. Baati, J. R. Falck and C. Mioskowski, Angew. Chem., Int. Ed., 2005, 44, 2008 CrossRef CAS PubMed.
Footnote |
† Electronic supplementary information (ESI) available. See DOI: 10.1039/c4qo00251b |
|
This journal is © the Partner Organisations 2014 |
Click here to see how this site uses Cookies. View our privacy policy here.