DOI:
10.1039/C4QO00250D
(Research Article)
Org. Chem. Front., 2014,
1, 1285-1288
A room-temperature synthesis of 2,2′-bisoxazoles through palladium-catalyzed oxidative coupling of α-isocyanoacetamides†
Received
18th September 2014
, Accepted 4th November 2014
First published on 5th November 2014
Abstract
A palladium-catalyzed synthesis of symmetric and unsymmetric 2,2′-bisoxazoles starting from readily available α-isocyanoacetamides was developed. The reaction was performed at room temperature in air which acted as the sole oxidant of Pd(0). Mechanistic studies suggested that double isocyanide insertion into the Pd(II)–O bond was involved.
Acting as an isoelectronic equivalent of carbon monoxide, isocyanide has shown its great potential in palladium-catalyzed isocyanide insertion reactions.1 Imidoyl palladium(II) complex A was considered as a general intermediate in reaction with various nucleophiles followed by reductive elimination, generating amidines,2 amides,3 ketimines,4 imidates, thioimidates5 and aldehydes6 correspondingly (Scheme 1). Functionalized heterocycles could be generated by linking a nucleophile to substrates R–X/R–H ready for imidoyl palladium(II) intermediate formation upon oxidative addition or C–H bond activation and isocyanide insertion.7 Another strategy for heterocycle construction involving isocyanide insertion as a key step employs bisnucleophiles and isocyanides under oxidative conditions.8 For instance, Orru and co-workers reported an efficient synthesis of cyclic guanidine derivatives and related heterocycles via palladium-catalyzed isocyanide insertion with diamines or amino alcohols.8a Recently, our group developed a different strategy aimed at construction of heterocycles by linking a nucleophile to an isocyanide substrate. α-Isocyanoacetamides, in which the carbonyl oxygen in the amide moiety acted as an intramolecular nucleophile, reacted with aryl, vinyl, or alkynyl halides under palladium catalysis to provide C2-diversified oxazoles.9 During the optimization of reaction conditions, a symmetric 2,2′-bisoxazole byproduct was identified, albeit in very low yield (Scheme 1). In this novel process, two oxazole rings are formed in one pot through multiple bond formation including two C–O bonds and one C–C bond starting from acyclic substrates. This unprecedented and unexpected transformation intrigued us to investigate it in detail.
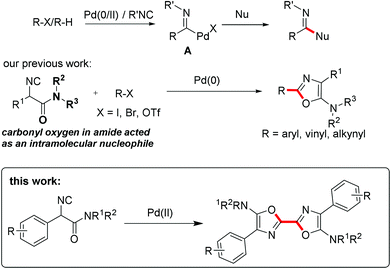 |
| Scheme 1 Palladium-catalyzed isocyanide insertion reactions. | |
C(sp2)–C(sp2) direct linked bisheterocycles are of vital importance in pharmaceuticals, natural products, and functional materials.10 Traditional approaches to these compounds are mostly based on heteroaryl (pseudo)halides and organometallic reagents.11 In recent years, more step-efficient and atom-economic strategies employing oxidative coupling of existing heterocyclic skeletons through C–H bond activation were developed.12 For instance, methods directed towards 2,2′-bisoxazoles were successfully developed by transition metal catalyzed coupling of dual C–H bonds.13 However, limitations of these methods, including high reaction temperatures, using a stoichiometric or excess amount of Cu/Ag-based oxidant, still exist. Herein, we report a novel palladium-catalyzed synthesis of symmetric and unsymmetric 2,2′-bisoxazoles by oxidative homo- and cross-coupling of readily available α-isocyanoacetamides.14 This reaction occurs smoothly at room temperature and uses air as the sole oxidant.
The reaction conditions were screened with 2-isocyano-2-phenyl-1-(piperidin-1-yl)ethanone 1a as a test substrate catalyzed by Pd(OAc)2 (10 mol%) in air at room temperature (Table 1). Among various solvents tested, the reaction performed best in MeCN in the presence of Cs2CO3 (1.1 equiv.) and PPh3 (20 mol%), delivering the desired symmetric 2,2′-bisoxazole 2a in 70% yield (entries 1–4). Further investigations including changing the reaction atmosphere from air to pure O2 or replacing the base from Cs2CO3 to LiOtBu gave lower yields of 2a (entries 5 and 7). In the absence of PPh3, the transformation was much less efficient (51% yield, entry 6). When a solution of 1a in MeCN (1.0 mL) was added slowly via a syringe pump over 0.5 h to a mixture containing Pd(OAc)2, PPh3, Cs2CO3 and 1 mL of MeCN, the yield of 2a increased slightly to 74% (entry 8).
Table 1 Optimization of reaction conditionsa
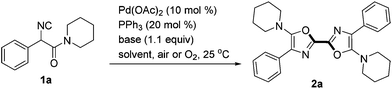
|
Entry |
Solvent |
Base |
Ligand |
Atmosphere |
Yieldb |
Reaction conditions: 1a (0.2 mmol), Pd(OAc)2 (10 mol%), base (0.22 mmol, 1.1 equiv.), PPh3 (20 mol%), solvent (2 mL), in air, 25 °C, 0.5 h.
Isolated yield.
2.0 h.
A solution of 1a in MeCN (1 mL) was added to the reaction mixture via a syringe pump within 0.5 h.
|
1 |
DMF |
Cs2CO3 |
PPh3 |
Air |
26% |
2 |
DCM |
Cs2CO3 |
PPh3 |
Air |
66% |
3 |
Dioxane |
Cs2CO3 |
PPh3 |
Air |
44% |
4 |
MeCN |
Cs2CO3 |
PPh3 |
Air |
70% |
5 |
MeCN |
Cs2CO3 |
PPh3 |
O2 |
44% |
6c |
MeCN |
Cs2CO3 |
— |
Air |
51% |
7 |
MeCN |
LiOtBu |
PPh3 |
Air |
50% |
8d |
MeCN |
Cs2CO3 |
PPh3 |
Air |
74% |
With the optimized reaction conditions in hand, the scope of α-isocyanoacetamides was then screened (Scheme 2). Besides piperidinyl amide, a cyclic morpholino analogue of 1a also generated the corresponding product 2b smoothly in 70% yield. Other α-isocyanoacetamides derived from acyclic secondary amines including N,N-dimethylamine (1c), N-methyl-N-ethylamine (1d), N,N-diethylamine (1e) and N-methylallylamine (1f) all homo-coupled efficiently to produce the corresponding symmetric 2,2′-bisoxazoles (2c–2f) in good yields. It is noteworthy that the terminal alkene in 2f survived the reaction well. Methyl and chloro substituted 2,2′-bisoxazoles 2g and 2h were obtained in 56% and 72% yields respectively. Unfortunately, isocyanoacetamide bearing a benzyl group rather than an aryl one at the α-position was not a suitable substrate in this transformation (1i).
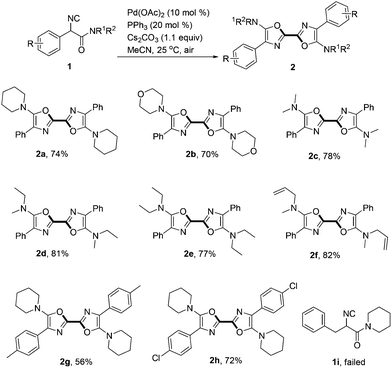 |
| Scheme 2 Scope of symmetric 2,2′-bisoxazoles. Reaction conditions: a solution of 1a (0.20 mmol) in MeCN (1 mL) was added to the reaction mixture containing Pd(OAc)2 (0.02 mmol, 10 mol%), PPh3 (0.04 mmol, 20 mol%), Cs2CO3 (0.22 mmol, 1.1 equiv.) and MeCN (1 mL) via a syringe pump within 0.5 h at 25 °C in air. | |
When two different α-isocyanoacetamides were present, an unsymmetric 2,2′-bisoxazole product derived from cross-coupling together with two homo-coupling products was obtained (Scheme 3). For example, addition of a solution of 1a (0.2 mmol, 1 equiv.) and 1b (3 equiv.) in 4 mL of MeCN to an open reaction tube containing the catalyst, ligand, base and CH3CN (1 mL) via a syringe pump in 1 h generated an unsymmetric 2,2′-bisoxazole product 3a in synthetically useful yield (51%) after careful chromatographic isolation. Symmetric 2,2′-bisoxazoles 2a and 2b generated from homo-coupling were also obtained in 13% and 69% yields, respectively. The selectivity for cross-coupling was better in a reaction of 1c and 1b, generating unsymmetric product 3b in 63% yield. Unsymmetric 2,2′-bisoxazole 3c containing an aromatic chloride functionality was also isolated in 57% yield. The current strategy provides an efficient approach to both symmetric and unsymmetric 2,2′-bisoxazoles in one step starting from simple acyclic α-isocyanoacetamides. It is notable that two heterocyclic rings are constructed simultaneously at ambient temperature in open air. Three chemical bonds including two C–O bonds and one C–C bond are formed with 100% atom-economy during this process.
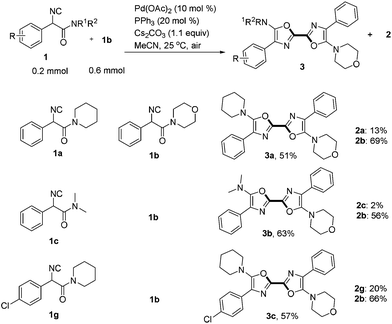 |
| Scheme 3 Scope of unsymmetric 2,2′-bisoxazoles. Reaction conditions: a solution of 1 (0.20 mmol) and 1b (0.6 mmol) in MeCN (4 mL) was added to the reaction mixture containing Pd(OAc)2 (0.02 mmol, 10 mol%), PPh3 (0.04 mmol, 20 mol%), Cs2CO3 (0.22 mmol, 1.1 equiv.) and MeCN (1 mL) via a syringe pump within 1 h at 25 °C in air. Isolated yields of 2b are based on 1b. Other yields are based on another reactant 1. | |
This reaction was scalable, as exemplified by sub-gram preparation of 2a with equal efficiency (a, Scheme 4). Further diversification of the obtained oxazole product 2h was also performed. Transforming the chloride moiety to boronic acid ester through palladium catalysis was realized in 89% yield. The product 4 containing two aromatic boronic acid ester moieties is expected to be a useful precursor for more complicated symmetric 2,2′-bisoxazole synthesis (b).15 Suzuki coupling of 2h with phenyl boronic acid also performed smoothly, giving highly conjugated product 5 in high yield (c).16
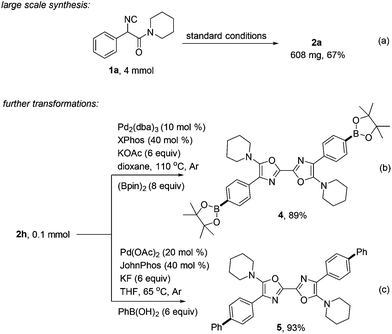 |
| Scheme 4 Large scale synthesis and further transformations. | |
To verify the reaction pathway, C2 unsubstituted oxazole 6 was treated under the standard aerobic conditions. Most of the starting material 6 was recovered with no homo-coupling product 2a being detected, which suggested that 6 was an unlikely reaction intermediate. Although the role of triphenyl phosphine was not fully understood, it may facilitate the process of reductive elimination and stabilize the Pd(0) species before being oxidized to Pd(II) by O2 in air.
A plausible reaction mechanism was proposed in
Scheme 5. Coordination of the carbonyl oxygen in α-isocyanoacetamide
1 with Pd(OAc)
2 affords intermediate
I. Deprotonation and the subsequent isocyanide insertion to the Pd–O bond forms the first oxazole ring in intermediate
III. Repeating the same process furnishes the key bisoxazole ligated palladium(
II) intermediate
VI. Reductive elimination releases the homo-coupling product
2 and the Pd
(0) species which is reoxidized to Pd
(II) by O
2 in air. It is also possible that isocyanide insertion to the Pd–O bond in Pd(OAc)
2 takes place before its coordination with the carbonyl oxygen.
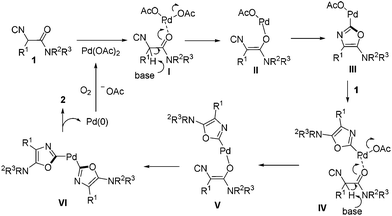 |
| Scheme 5 Proposed mechanism. | |
In summary, we have developed a novel palladium-catalyzed synthesis of symmetric and unsymmetric 2,2′-bisoxazoles starting from readily available acyclic α-isocyanoacetamides. Double isocyanide insertion was believed as a key step in this transformation. The reaction was performed at room temperature in air which acted as the sole oxidant of Pd(0). The resulting symmetric or unsymmetric products were highly π-conjugated, showing their great potential in functional material synthesis.
This work was supported by National Science Foundation of China (21202167).
Notes and references
- For reviews, see:
(a) T. Vlaar, B. W. Maes, E. Ruijter and R. V. A. Orru, Angew. Chem., Int. Ed., 2013, 52, 7084 CrossRef CAS PubMed;
(b) S. Lang, Chem. Soc. Rev., 2013, 42, 4867 RSC;
(c) G. Qiu, Q. Ding and J. Wu, Chem. Soc. Rev., 2013, 42, 5257 RSC.
- C. G. Saluste, R. J. Whitby and M. Furber, Angew. Chem., Int. Ed., 2000, 39, 4156 CrossRef CAS.
-
(a) H. Jiang, B. Liu, Y. Li, A. Wang and H. Huang, Org. Lett., 2011, 13, 1028 CrossRef CAS PubMed;
(b) J. Peng, L. Liu, Z. Hu, J. Huang and Q. Zhu, Chem. Commun., 2012, 48, 3772 RSC.
- M. Tobisu, S. Imoto, S. Ito and N. Chatani, J. Org. Chem., 2010, 75, 4835 CrossRef CAS PubMed.
- C. G. Saluste, R. J. Whitby and M. Furber, Tetrahedron Lett., 2011, 42, 6191 CrossRef.
- X. Jiang, J. M. Wang, Y. Zhang, Z. Chen, Y. M. Zhu and S.-J. Ji, Org. Lett., 2014, 16, 3492 CrossRef CAS PubMed.
-
(a) Y. Wang, H. Wang, J. Peng and Q. Zhu, Org. Lett., 2011, 13, 4604 CrossRef CAS PubMed;
(b) Y. Wang and Q. Zhu, Adv. Synth. Catal., 2012, 354, 1902 CrossRef CAS;
(c) G. van Baelen, S. Kuijer, L. Rýćek, S. Sergeyev, E. Janssen, F. J. J. de Kanter, B. U. W. Maes, E. Ruijter and R. V. A. Orru, Chem. – Eur. J., 2011, 17, 15039 CrossRef CAS PubMed;
(d) T. Vlaar, E. Ruijter, A. Znabet, E. Jansson, F. J. J. de Kanter, B. U. W. Maes and R. V. A. Orru, Org. Lett., 2011, 13, 6496 CrossRef CAS PubMed;
(e) G. Qiu, G. Liu, S. Pu and J. Wu, Chem. Commun., 2012, 44, 2903 RSC;
(f) G. Qiu, Y. Lu and J. Wu, Org. Biomol. Chem., 2013, 11, 798 RSC;
(g) G. Qiu, Y. He and J. Wu, Chem. Commun., 2012, 48, 3836 RSC;
(h) Y. Li, J. Zhao, H. Chen, B. Liu and H. Jiang, Chem. Commun., 2012, 48, 3545 RSC;
(i) B. Liu, Y. Li, H. Jiang, M. Yin and H. Huang, Adv. Synth. Catal., 2012, 354, 2288 CrossRef CAS;
(j) B. Liu, Y. Li, M. Wu and H. Jiang, Chem. Commun., 2012, 48, 11446 RSC;
(k) V. Tyagi, S. Khan, A. Giri, H. M. Gauniyal, B. Sridhar and P. M. S. Chauhan, Org. Lett., 2012, 14, 3126 CrossRef CAS PubMed;
(l) X.-D. Fei, Z.-Y. Ge, T. Tang, Y.-M. Zhu and S.-J. Ji, J. Org. Chem., 2012, 77, 10321 CrossRef CAS PubMed;
(m) Y. Ito, I. Ito, T. Hirao and T. Saegusa, Synth. Commun., 1974, 4, 97 CrossRef CAS;
(n) N. Thirupathi, M. H. Babu, V. Dwivedi, R. Kant and M. S. Reddy, Org. Lett., 2014, 16, 2908 CrossRef CAS PubMed;
(o) X. Jiang, T. Tang, J.-M. Wang, Z. Chen, Y.-M. Zhu and S.-J. Ji, J. Org. Chem., 2014, 79, 5082 CrossRef CAS PubMed;
(p) R. Mancuso, I. Ziccarelli, D. Armentano, N. Marino, S. V. Giofrè and B. Gabriele, J. Org. Chem., 2014, 79, 3506 CrossRef CAS PubMed.
-
(a) T. Vlaar, R. C. Cioc, P. Mampuys, B. U. W. Maes, R. V. A. Orru and E. Ruijter, Angew. Chem., Int. Ed., 2012, 51, 13058 CrossRef CAS PubMed;
(b) B. Liu, M. Yin, H. Gao, W. Wu and H. Jiang, J. Org. Chem., 2013, 78, 3009 CrossRef CAS PubMed;
(c) T. Vlaar, R. V. A. Orru, B. U. W. Maes and E. Ruijter, J. Org. Chem., 2013, 78, 10469 CrossRef CAS PubMed;
(d) T. Fang, Q. Tan, Z. Ding, B. Liu and B. Xu, Org. Lett., 2014, 16, 2342 CrossRef CAS PubMed;
(e) T.-H. Zhu, S.-Y. Wang, G.-N. Wang and S.-J. Ji, Chem. – Eur. J., 2013, 19, 5850 CrossRef CAS PubMed.
- J. Wang, S. Luo, J. Huang, T. Mao and Q. Zhu, Chem. – Eur. J., 2014, 20, 11220 CrossRef CAS PubMed.
-
(a)
Polycyclic Aromatic Hydrocarbons, ed. R. G. Harvey, Wiley-VCH, New York, 1996 Search PubMed;
(b) R. Capdeville, E. Buchdunger, J. Zimmermann and A. Matter, Nat. Rev. Drug Discovery, 2002, 1, 493 CrossRef CAS PubMed;
(c)
I. Cepanec, Synthesis of Biaryls, Elsevier, Amsterdam, 2004 Search PubMed;
(d)
V. Balzani, A. Credi and M. Venturi, Molecular Devices and Machines, Wiley-VCH, Weinheim, 2008 Search PubMed;
(e) A. M. Norberg, L. Sanchez and R. E. Maleczka, Jr., Curr. Opin. Drug Discovery Dev., 2008, 11, 853 CAS;
(f) Recent developments: S. Hiraoka, Y. Hisanaga, M. Shiro and M. Shionoya, Angew. Chem., Int. Ed., 2010, 49, 1669 CrossRef CAS PubMed.
-
(a) J. K. Stille, Angew. Chem., Int. Ed., 1986, 25, 508 CrossRef;
(b) N. Miyaura and A. Suzuki, Chem. Rev., 1995, 95, 2457 CrossRef CAS;
(c)
Metal-catalyzed cross-coupling reactions, ed. F. Diederich and P. J. Stang, Wiley-VCH, New York, 1998 Search PubMed;
(d) S. P. Stanforth, Tetrahedron, 1998, 54, 263 CrossRef CAS;
(e) J. Hassan, M. Sévignon, C. Gozzi, E. Schulz and M. Lemaire, Chem. Rev., 2002, 102, 1359 CrossRef CAS PubMed;
(f) M. Schnürch, R. Flasik, A. F. Khan, M. Spina, M. D. Mihovilovic and P. Stanetty, Eur. J. Org. Chem., 2006, 3283 CrossRef;
(g) S. Yanagisawa, T. Sudo, R. Noyori and K. Itami, J. Am. Chem. Soc., 2006, 128, 11748 CrossRef CAS PubMed;
(h) K. Billingsley and S. L. Buchwald, J. Am. Chem. Soc., 2007, 129, 3358 CrossRef CAS PubMed.
- For selected examples, see:
(a) S.-L. You and J.-B. Xia, Top. Curr. Chem., 2010, 292, 165 CrossRef CAS;
(b) T. W. Lyons and M. S. Sanford, Chem. Rev., 2010, 110, 1147 CrossRef CAS PubMed;
(c) T. Newhouse and P. S. Baran, Angew. Chem., Int. Ed., 2011, 50, 3362 CrossRef CAS PubMed;
(d) C. S. Yeung and V. M. Dong, Chem. Rev., 2011, 111, 1215 CrossRef CAS PubMed;
(e) L. McMurray, F. O. Hara and M.
J. Gaunt, Chem. Soc. Rev., 2011, 40, 1885 RSC;
(f) X. Bugaut and F. Glorius, Angew. Chem., Int. Ed., 2011, 50, 7479 CrossRef CAS PubMed;
(g) Z. Wang, K. Li, D. Zhao, J. Lan and J. You, Angew. Chem., Int. Ed., 2011, 50, 5365 CrossRef CAS PubMed;
(h) J. Dong, Z. Long, F. Song, N. Wu, Q. Guo, J. Lan and J. You, Angew. Chem., Int. Ed., 2013, 52, 580 CrossRef CAS PubMed;
(i) S. Fan, Z. Chen and X. Zhang, Org. Lett., 2012, 14, 4950 CrossRef CAS PubMed;
(j) Z. Mao, Z. Wang, Z. Xu, F. Huang, Z. Yu and R. Wang, Org. Lett., 2012, 14, 3854 CrossRef CAS PubMed;
(k) N.-N. Li, Y.-L. Zhang, S. Mao, Y.-R. Gao, D.-D. Guo and Y.-Q. Wang, Org. Lett., 2014, 16, 2732 CrossRef CAS PubMed.
- For selected examples, see:
(a) W. Han, P. Mayer and A. R. Ofial, Angew. Chem., Int. Ed., 2011, 50, 2178 CrossRef CAS PubMed;
(b) J. Dong, Y. Huang, X. Qin, Y. Cheng, J. Hao, D. Wan, W. Li, X. Liu and J. You, Chem. – Eur. J., 2012, 18, 6158 CrossRef CAS PubMed;
(c) M. Zhu, K. Fujita and R. Yamaguchi, Chem. Commun., 2011, 47, 12876 RSC;
(d) Y. Li, J. Jin, W. Qian and W. Bao, Org. Biomol. Chem., 2010, 8, 326 RSC.
-
(a) A. V. Gulevich, A. G. Zhdanko, R. V. A. Orru and V. G. Nenajdenko, Chem. Rev., 2010, 110, 5235 CrossRef CAS PubMed;
(b) A. Fayol, C. Housseman, X. Sun, P. Janvier, H. Bienaymé and J. Zhu, Synthesis, 2005, 161 CAS;
(c) C. Housseman and J. Zhu, Synlett, 2006, 1777 CAS.
- K. L. Billingsley, T. E. Barder and S. L. Buchwald, Angew. Chem., Int. Ed., 2007, 46, 5359 CrossRef CAS PubMed.
- J. P. Wolfe, R. A. Singer, B. H. Yang and S. L. Buchwald, J. Am. Chem. Soc., 1999, 121, 9550 CrossRef CAS.
Footnote |
† Electronic supplementary information (ESI) available. See DOI: 10.1039/c4qo00250d |
|
This journal is © the Partner Organisations 2014 |
Click here to see how this site uses Cookies. View our privacy policy here.