DOI:
10.1039/C3RA44489A
(Paper)
RSC Adv., 2014,
4, 147-153
Synthesis, crystal structures and luminescent properties of CdII and ZnII complexes assembled by 4-aminophenylhydroxamic acid†
Received
20th August 2013
, Accepted 28th October 2013
First published on
4th November 2013
Abstract
Four complexes of composition [Cd(4-Apha)2]n (1), [Zn2(4-Apha)4(H2O)2]·2H2O (2), [Zn(4-Apha)(CH3COO)]n (3), and [Zn4(4-Apha)4(CH3COO)4(H2O)]n·2nCH3OH (4) (4-AphaH = 4-aminophenylhydroxamic acid) have been synthesized in different solvents and characterized by X-ray diffraction, IR spectroscopy, thermal analysis and elemental analysis. X-ray crystal analysis reveals that these complexes exhibit four varieties of structures. Complex 1 displays a 1-D chain structure, and complex 2 is binuclear. Complexes 3 and 4 possess different 3-D structures. Fluorescence emissions are observed for complexes 1, 2 and 4 in solid state.
Introduction
Hydroxamic acids (HAc) are important bioligands, broadly utilized in medicine and chemical biology.1 They function as potent and selective inhibitors of enzymes and have hypotensive, anti-cancer, anti-malarial and anti-fungal properties.2 They could coordinate to metals in monodentate, bidentate and tridentate fashion, as observed in some urease and metal-proteins.3 The powerful metal-chelating ability and rich coordination modes of hydroxamic acids have also been employed to construct a diverse host of fascinating coordination compounds including classical homometallic di- and trinuclear complexes,4 high-nuclear clusters,5 and transition- and lanthanide-transition- metallacrowns.6 It is found that the coordination behaviours of phenylhydroxamic acids can be tailored by the incorporation of various functional groups, e.g., –NMe2,5b –OH,7 –NH2,8etc., at ortho, meta and (or) para positions of benzene rings. However, to our surprise, hydroxymic acids have rarely been utilized as building blocks in creating 1-D, 2-D and 3-D coordination polymers,9 which might have potential applications in single-chain magnets, gas adsorption, and luminescence materials.10
We are interested in employing 4-aminophenylhydroxamic acid (4-AphaH) as a ligand to create the coordination polymers of CdII and ZnII. In 4-AphaH, the hydroxamic group can assemble several metals into a polynuclear moiety, and the extra amine group in para position may serve as the bridging ligand to link the polynuclear moiety with another metal, generating coordination polymers. Furthermore, the chemistry of diamagnetic ZnII and CdII coordination complexes in the forms of monomeric, multinuclear, and polymeric species have attracted much attention in recent years.11 Coordination polymers of CdII and ZnII have been widely investigated for photoluminescent properties and for potential applications as fluorescence-emitting materials, such as light-emitting diodes (LEDs).12 They have high thermal stability and the ability to affect the emission wavelength of the organic material by metal coordination. For applications in electroptical devices, ZnII complexes supported by 2-benzothiazol-2-yl-phenol13 or 1H-pyrrolo[2,3-b]pyridine ligands,14 are electroluminescent materials and have been applied in organic light-emitting diodes (OLEDs). With coordination polymeric structures,15 the inhibition of peptide deformylase of ZnII complexes in biological systems,16 the catalytic activity of CdII complexes in cyanosilylation reactions17 have also been studied, whereas the coordination polymers of ZnII/CdII of hydroxamic acids were rarely reported in the literature.16b,9b,18
The results of the present investigation gave four kinds of complexes: [Cd(4-Apha)2]n (1), [Zn2(4-Apha)4(H2O)2]·2H2O (2), [Zn(4-Apha)(CH3COO)]n (3), and [Zn4(4-Apha)4(CH3COO)4(H2O)]n·2nCH3OH (4) (4-AphaH = 4-aminophenylhydroxamic acid). Herein, we report the syntheses, structures, thermal stabilities and luminescent properties of these complexes.
Experimental section
General procedure
Materials and instruments.
The ligand 4-APhaH was prepared by the literature method.6j All reagents used in the synthesis were of analytical grade. The C, H and N microanalyses were carried out with a Carlo-Erba EA1110 CHNO-S elemental analyzer. FT-IR spectra were recorded from KBr pellets in the range of 400–4000 cm−1 on a Nicolet MagNa-IR 500 spectrometer. Powder X-ray diffraction (PXRD) was recorded on a Rigaku D/Max-2500 diffractiometer at 40 kV and 100 Ma with a Cu-target tube and a graphite monochromator. Thermal gravimetric analysis (TGA) was conducted on a NETZSCH STA 449F3 instrument in flowing N2 with a heating rate of 5 °C min−1. The solid-state luminescence emission/excitation spectra were recorded on a FLS920 fluorescence spectrophotometer equipped with a continuous Xe-900 xenon lamp and a μF900 microsecond flash lamp.
Syntheses of the complexes
[Cd(4-Apha)2]n (1).
A mixture of Cd(CH3COO)2·2H2O (0.0533 g, 0.20 mmol), 4-AphaH (0.0304 g, 0.20 mmol) and water (1.0 mL) was placed in a Pyrex-tube (8 mL). The tube was heated at 70 °C for 1 day under autogenous pressure. Cooling of the resultant solution to room temperature gave pink rod crystals. The crystals were collected by filtration, washed with water (2 mL) and dried in air. Yield: 0.021 g (50%, based on 4-AphaH). Anal. calc. for C14H14CdN4O4 (1): C, 40.55; H, 3.40; N, 13.51. Found: C, 40.42; H, 3.19; N, 13.32%. Selected IR data (KBr, cm−1): 3370(s), 3161(s), 1603(s), 1555(s), 1495(s), 1296(s), 1152(s), 1031(s), 906(s), 841(s), 751(s), 693(s), 628(s), 513(s).
[Zn2(4-Apha)4(H2O)2]·2H2O (2).
A mixture of Zn(CH3COO)2·2H2O (0.0221 g, 0.10 mmol), 4-AphaH (0.0154 g, 0.10 mmol) and water (1.0 mL) was placed in a Pyrex-tube (8 mL). The tube was heated at 70 °C for 1 day under autogenous pressure. Cooling of the resultant solution to room temperature gave pink rod crystals. The crystals were collected by filtration, washed with water (2 mL) and dried in air. Yield: 0.013 g (63%, based on 4-AphaH). Anal. Calc. for C28H36N8O12Zn2 (2): C, 41.65; H, 4.49; N, 13.88. Found: C, 41.53; H, 4.39; N, 13.75%. Selected IR data (KBr, cm−1): 3425(m), 3380(m), 1609(s), 1553(s), 1494(m), 1357(s), 1301(m), 1151(s), 1043(m), 1028(m), 910(s), 842(s), 690(m), 628(s), 523(s).
[Zn(4-Apha)(CH3COO)]n (3).
Complex 3 was synthesized using the same method with that of complex 2, except for employing ethanol (or methanol) as the solvent. Needle-like crystals were obtained. Yield: 0.020 g (72%, based on 4-AphaH). Anal. calc. for C9H10N2O4Zn (3): C, 39.23; H, 3.66; N, 10.17. Found: C, 38.82; H, 3.81; N, 9.87%. Selected IR data (KBr, cm−1): 3421(w), 3370(s), 1607(s), 1556(s), 1493(s), 1430(s), 1293(s), 1152(s), 1037(s), 915(s), 841(s), 630(s).
Synthesis of [Zn4(4-Apha)4(CH3COO)4(H2O)]n·2nCH3OH (4).
Complex 4 was synthesized using the same method with that of complex 3, except that before the 4-AphaH ligand was add, water (0.1 mL) was added to wet Zn(CH3COO)2·2H2O, and methanol (0.9 mL) was then added to the reaction system. Pink block crystals were obtained. Yield: 0.011 g (38%, based on 4-AphaH). Anal. calc. for C38H50N8O19Zn4 (4): C, 38.54; H, 4.26; N, 9.46. Found: C, 38.34; H, 4.29; N, 9.32%. Selected IR data (KBr, cm−1): 3355(w), 3250(w), 1608(s), 1562(s), 1497(s), 1424(s), 1316(s), 1154(s), 1043(s), 918(s), 846(s), 663(s), 629(s).
The PXRD patterns of complexes 1–4 are presented in Fig. S5.† The diffraction peaks of both simulated and experimental patterns match well, indicating the phase purities of these complexes.
X-ray crystallography
Data were collected at room temperature on Bruker Smart ApexII diffractometer equipped with a graphite monochromator utilising Mo Kα radiation (λ = 0.71073 Å); the ω and φ scan technique was applied. The structures were solved by direct methods using SHELXS-97
19 and refined on F2 using full-matrix least-squares with SHELXL-97.20 Crystallographic data together with refinement details for the new complexes reported in this work are summarised in Table 1. See CIF files for further details.
Table 1 Crystal data and structure refinement information for complexes 1–4
|
1
|
2
|
3
|
4
|
Including solvate molecules.
Mo Kα radiation.
R
1 = Σ(|Fo| − |Fc|)/Σ(|Fo|) for observed reflections.
w = 1/[σ2(Fo2) + (αP)2 + bP] and P = [max(Fo2, 0) + 2Fc2]/3.
wR2 = {Σ[w(Fo2 − Fc2)2]/Σ[w(Fo2)2]}1/2 for all data.
|
Formulaa |
CdC14H14N4O4 |
Zn2C28H36N8O12 |
ZnC9H10N2O4 |
Zn4C38H50N8O19 |
M/g mol−1a |
414.70 |
807.39 |
275.56 |
1184.34 |
T/K |
223(2) |
296(2) |
296(2) |
290(2) |
λ
b/Å |
0.71073 |
0.71073 |
0.71073 |
0.71073 |
Crystal system |
Orthorhombic |
Monoclinic |
Tetragonal |
Monoclinic |
Space group |
Iba2 |
P21/n |
I41/a |
Cc
|
a/Å |
12.121(2) |
10.5931(6) |
19.7107(9) |
26.7007(19) |
b/Å |
17.543(4) |
7.6567(4) |
19.7107(9) |
9.7429(6) |
c/Å |
6.9785(14) |
19.8339(11) |
11.0593(10) |
18.8038(12) |
β/° |
90 |
93.2620(10) |
90 |
90.254(2) |
V/Å3 |
1483.9(5) |
1606.09(15) |
4296.7(5) |
4891.6(6) |
Z
|
4 |
2 |
16 |
4 |
ρ
c/g cm−3 |
1.856 |
1.670 |
1.704 |
1.608 |
μ/mm−1 |
1.498 |
1.570 |
2.286 |
2.018 |
F(000) |
824 |
832 |
2240 |
2424 |
θ range/° |
2.04–24.99 |
2.06–27.95 |
2.07 to 26.35 |
2.17–28.31 |
Measd/independent |
3194/1291 |
10 613/3828 |
26 488/2199 |
34 256/11 775 |
R
int reflections |
0.0243 |
0.0202 |
0.0233 |
0.0252 |
Obsd reflns [I > 2σ (I)] |
1291 |
3828 |
2199 |
11 775 |
GOF on F2 |
1.066 |
1.063 |
1.133 |
1.093 |
R
1
c
|
0.0183 |
0.0287 |
0.0340 |
0.0385 |
wR2d,e |
0.0458 |
0.0795 |
0.1082 |
0.1165 |
(Δρ)max,min/e Å−3 |
0.212, −0.215 |
0.814, −0.443 |
1.002, −0.288 |
0.944, −0.502 |
Results and discussion
Syntheses of the complexes
Complex 1 was prepared by heating a mixture of Cd(CH3COO)2·2H2O, 4-AphaH and water under soft-hydrothermal conditions at 70 °C. Pink rod crystals of [Cd(4-Apha)2]n (1) were generated after heating the mixture for 1 day. We also explored the reactions of Cd(CH3COO)2·2H2O and 4-AphaH in methanol and ethanol, respectively, and the same crystals were afforded.
Treatment of Zn(CH3COO)2·2H2O with 4-AphaH in water gave a dinuclear complex [Zn2(4-Apha)4(H2O)2]·2H2O (2). When the reaction of Zn(CH3COO)2·2H2O and 4-AphaH was conducted in ethanol, a coordination polymer [Zn(4-Apha)(CH3COO)]n (3) was produced.
Intrigued by the observation that the complexes with the totally different compositions were afforded in the different solvents, we examined the reaction of Zn(CH3COO)2·2H2O and 4-AphaH in methanol. Complex [Zn(4-Apha)(CH3COO)]n (3) was generated. We were curious if another new complex could be given in the mixed solvent of water and methanol, we then investigated the reaction of Zn(CH3COO)2·2H2O and 4-AphaH in the mixture of water and methanol (v/v = 1
:
9). Gratifyingly, a coordination polymer [Zn4(4-Apha)4(CH3COO)4(H2O)]n·2nCH3OH (4) was prepared.
The successful generation of 2–4 indicates that the coordination complexes with different structures could be assembled via employing different solvents, demonstrating the synthetic novelty of this work.
Description of structures
Structure of [Cd(4-Apha)2]n (1).
The crystal structure analysis revealed that 1 crystallizes in the orthorhombic crystal system of the Iba2 space group. The crystal structure of 1 (Fig. 1a) consists of one CdII ion and two singly deprotonated 4-Apha− ligands. The adjacent CdII ions are bridged by the hydroximate oxygen atoms to form a one-dimensional linear chain. Each CdII ion is bound to six oxygen atoms (O1, μ2-O2, μ2-O2A, O1A, μ2-O2C and μ2-O2B) originated from four different 4-Apha− ligands in a slightly distorted octahedral geometry. Each 4-Apha− ligand chelates one CdII ion through a carbonyl oxygen atom and a μ2-hydroxamate oxygen atom (coordination mode A in Scheme 1), with Cd–O distances ranging from 2.221(2) to 2.341(3) Å. The Cd⋯Cd distance is 3.489 Å, and all bond lengths and angles of Cd–O are of typical values compared with other CdII complexes.11b,12 In addition, complex 1 features intermolecular hydrogen bonds (N–H⋯O, N–H⋯N) between NH2 groups as hydrogen donors and O or N atoms of HN–O(1) groups in the 4-Apha− ligands as hydrogen acceptors. The H2B to O2 distance of N2–H2B⋯O2 is 2.05 Å, and N2–H2B⋯O2 angle is 167.4° (symmetry operation: −x + 3/2, y + 1/2, z + 0); The H2B to N1 distance of N2–H2B⋯N1 is 2.58 Å, and N2–H2B⋯N1 angle is 149.1° (symmetry operation: −x + 3/2, y + 1/2, z + 0). These two kinds of hydrogen bonds link the 1-D chains to give a 3-D supramolecular structure (Fig. 1b).
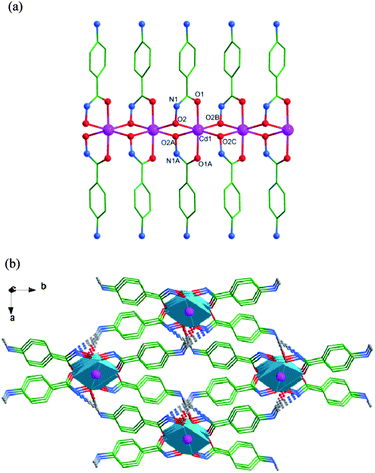 |
| Fig. 1 (a) The crystal structure of 1. Hydrogen atoms have been omitted for clarity. Symmetry transformations: A: −x + 1, y, z + 1/2; B: x, −y + 1, z + 1/2; C: −x + 1, −y + 1, z. (b) 3-D polyhedron framework of 1 created by intermolecular hydrogen bonds viewed along c-axis. Hydrogen atoms of the aromatic rings have been omitted for clarity. Color codes: pink, Cd; red, O; blue, N; green, C. | |
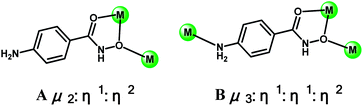 |
| Scheme 1 The coordination modes of Apha− in complexes 1 to 4. | |
Complex 1 is a member of a small family of chain complexes with hydroxamic acids as ligands.1,11b The chain configuration is a common topology in CdII compounds,12a but it is never observed in the coordination complexes of hydroxamic acids.
Structure of [Zn2(4-Apha)4(H2O)2]·2H2O (2).
X-ray single crystal diffraction analysis showed that 2 crystallizes in the monoclinic crystal system of P21/n space group. The crystal structure of 2 is composed of a binuclear [Zn2(4-Apha)4(H2O)2] unit and two solvent water molecules. Each ZnII ion is six coordinated by five oxygen atoms from three 4-Apha− ligands (one is in μ2:η1:η1 mode and the others are in μ3:η1:η1:η2 modes; Scheme 1) and one oxygen atom from a water molecule in a slightly distorted octahedron geometry (Fig. 2). The μ2-hydroxamate oxygen atoms (O2 and O2A) bridge two neighbouring ZnII ions to form a binuclear complex. The Zn–O distances range from 2.055(1) to 2.162(1) Å, the Zn1–O2–Zn1A angle is 96.9°, and the Zn1⋯Zn1A distance is 3.189 Å.
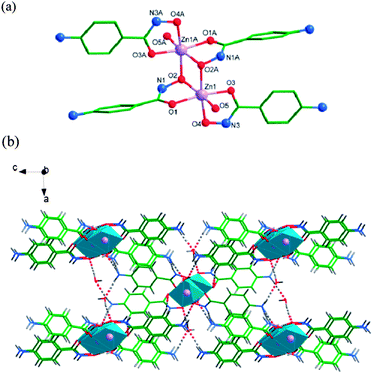 |
| Fig. 2 (a) The crystal structure of 2. Hydrogen atoms and solvent water molecules have been omitted for clarity. Symmetry transformations: A: −x, −y + 2, −z. (b) 3-D polyhedron framework of 2 created by intermolecular hydrogen bonds viewed along b-axis. Color codes: lavender, Zn; red, O; blue, N; green, C; gray, H. | |
The intermolecular hydrogen interactions between N1 atoms of the NH units as hydrogen donors and N2 atoms of NH2 groups as hydrogen acceptors of the 4-Apha− ligands (N1–H1⋯N2: the angle of N1–H1⋯N2 is 152.2°, the distance of H1⋯N2 is 2.27 Å, symmetry operation: −x + 1/2, y + 1/2, −z − 1/2) connect the binuclear complexes to generate a 2-D network structure in bc plane. The hydrogen bonds between N (HN and NH2) atoms of the 4-Apha− ligands as hydrogen donors and O atoms of water molecules as hydrogen acceptors connect the 2-D networks giving a 3-D supramolecular structure (Fig. 2b). Lattice water molecules are connected to the main structure by O–H⋯O hydrogen bonds. See Table S4† for the details.
Structure of [Zn(4-Apha)(CH3COO)]n (3).
X-ray crystallography revealed that 3 possesses a 3-D network structure. The asymmetric unit of 3 is composed of one ZnII ion, one 4-Apha− ligand and one acetate. As shown in Fig. 3a, each ZnII ion is six coordinate in slightly distorted octahedron geometry. The Zn1 centre is coordinated by two μ2-hydroxamate oxygen atoms (μ2-O5, μ2-O5A), one μ1-carbonyl oxygen atom (O1) and one amino nitrogen atom (N2) of three 4-Apha− ligands and two carboxylate oxygen atoms (O3, O4B) of two acetate ions. The neighbouring ZnII ions are doubly bridged by μ2-hydroxamate oxygen atoms and carboxylate groups to form left- and right-handed 1-D [ZnO(CH3COO)]n helices chain structures along c-axis (Fig. 3b). These left- and right-handed helices chains are connected by the 4-Apha− ligands along a-axis and b-axis to form a 3-D framework (Fig. 3c). The acetate shows the μ2:η1:η1 mode. In 3, the 4-Apha− ligands all adopt the μ3:η1:η1:η2 coordination mode.
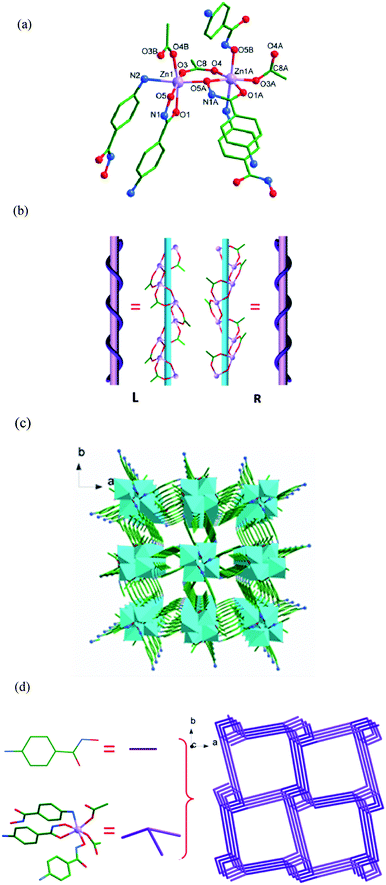 |
| Fig. 3 (a) The coordination environment of the Zn1 ion in 3. Hydrogen atoms and solvent water molecules have been omitted for clarity. Symmetry transformations: A: −y + 3/4, x + 1/4, z + 1/4; B: y − 1/4, −x + 3/4, z − 1/4. (b) The left- and right-handed 1-D [ZnO(CH3COO)]n helices chains of 3. (c) 3-D polyhedron framework structure of 3. (d) Schematic representation of the 3-connected topology with the topological notation 82.10 in the ab plane. Hydrogen atoms have been omitted for clarity. Color codes: lavender, Zn; red, O; blue, N; green, C. | |
To better understand the nature of the 3-D framework, a topological approach has been applied. Each Zn1 atom can be considered as a 3-connected node, and each 4-Apha− ligand serving as a linear linker connects two adjacent 3-connected nodes (Fig. 3d). The whole structure can be simplified to a topology with Schläfli symbol of 82.10.21
In 3, the N1–H1⋯O5 interaction between N1 atom of 4-Apha− ligand as the donor and O5 atom as the acceptor, with the N1–H1⋯O5 angle of 124.8°, the H1⋯O5 distance of 2.20 Å, symmetry operation y − 1/4, x + 3/4, z − 1/4 (Table S6†), occurs. A weak π⋯π interaction between the benzene rings of the 4-Apha− ligands has been found, with the π-centroid to π-centroid distance of 3.836(2) Å.
Structure of [Zn4(4-Apha)4(CH3COO)4(H2O)]n·2nCH3OH (4).
The crystal structure of 4 exhibits a 3-D framework structure, which is constructed from the 4-Apha− ligands, ZnII ions and coordination water molecules. The asymmetric unit (Fig. 4) contains four independent ZnII ions, four 4-Apha− ligands, and a coordinated water molecule. Each Zn ion is in a slightly distorted octahedral geometry. As shown in Fig. 4a, Zn1 ion is coordinated by two μ2-hydroxamate oxygen atoms (μ2-O2, μ2-O14A) from two 4-Apha− ligands, two carboxylate oxygen atoms (O3, O16A), one nitrogen atom (N2B) from another 4-Apha− ligand and one water molecule (O17). The Zn1 ion bridges both Zn2 and Zn4A ions by μ2-hydroxamate oxygen atoms and carboxylate groups. The Zn2 ion is coordinated by six oxygen atoms (μ2-O2, O1, O5, μ2-O6) arising from two 4-Apha− ligands and two acetates (O4, O7), with the Zn1–O distance ranging from 2.020(3) to 2.204(4) Å. Zn3 ion is coordinated by two μ2-hydroxamate oxygen atoms (μ2-O6, μ2-O10) of two 4-Apha− ligands, two amino nitrogen atoms (N4, N6) of another two 4-Apha− ligands and two carboxylate oxygen atoms (O8, O11) (Fig. 4b). The Zn3 ion bridges both Zn2 and Zn4 ions by μ2-hydroxamate oxygen atoms and carboxylate groups. The Zn4 ion is coordinated by six oxygen atoms (μ2-O10, O9, O13, μ2-O14) originated from two 4-Apha− ligands and two carboxylate oxygen atoms (O12, O15), with the Zn4–O distances ranging from 2.054(4) to 2.177(4) Å. These four ZnII ions are bridged by μ2-hydroxamate oxygen atoms and carboxylate groups to form a [ZnO(CH3COO)]4n 1-D chain structure, which is different from that of 3. The chains are connected by the 4-Apha− ligands in six different directions to give a 3-D network (Fig. 4c).
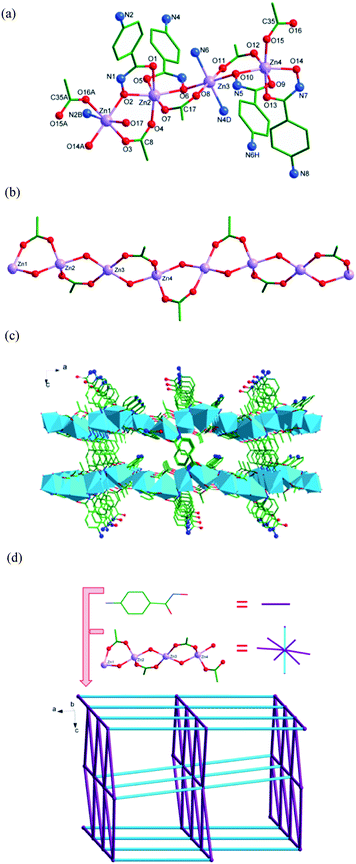 |
| Fig. 4 (a) The coordination environments of the ZnII ions in 4. Symmetry transformations: A: x − 1/2, y + 1/2, z; B: x, y + 1, z; D: x, −y + 2, z − 1/2; H: x, −y + 1, z − 1/2. (b) The structure of [ZnO(CH3COO)]4n 1-D chain. (c) The polyhedron framework structure of 4. (d) Schematic representation of the 8-connected topology with the topological notation 36·414·57·6 in the ac plane. Hydrogen atoms and solvent methanol molecules have been omitted for clarity. Color codes: lavender, Zn; red, O; blue, N; green, C. | |
To better understand the nature of the 3-D framework, a topological approach has been applied. The tetranuclear [ZnO(CH3COO)]4 unit (Zn1, Zn2, Zn3 and Zn4 as the node centres) can be considered as a 8-connected node, and each 4-Apha− serving as a linear linker connects two adjacent [ZnO(CH3COO)]4 units (Fig. 4d). The whole structure can be simplified to a topology with Schläfli symbol of 36·414·57·6.21
Both intermolecular and intramolecular hydrogen interactions between N atoms (NH and NH2) of 4-Apha− as hydrogen donors and O atoms of 4-Apha− (or acetic acid, or coordinated water molecular) as the acceptors have been found in 4. Interactions between O17 atom of coordination water molecule as the donor and N8 (or O7) atom of the 4-Apha− ligand has been found (O17–H17A⋯N8: the O17–H17A⋯N8 angle is 165.4°, the H17A-N8 distance is 2.03 Å, and the symmetry operation −1/2 + x, −1/2 + y, z; O17H–17B⋯O7: the angle of O17H–17B⋯O7 is 102.0°, the distance of 17B⋯O7 is 2.49 Å, and no symmetry operation). These hydrogen bonds enhance the stability of the crystal structure. The lattice methanol molecules connect the main structure by O19–H19⋯N6 and O18–H18⋯O5 hydrogen interactions (O19–H19⋯N6: the distance of H19⋯N6 is 2.51 Å, and the angle of O19–H19⋯N6 is 123.3°; O18–H18⋯O5: the angle of O18–H18⋯O5 is 164.2°, the distance of H18⋯O5 is 2.05 Å. both of them have no symmetry operation). See Table S8† for the details.
Complexes 3 and 4 join a small family of 3-D frameworks with hydroxamic acids as ligands.1,9b However, the ZnII complexes with 3-D structures have never been observed in the coordination complexes of hydroxamic acids.
Luminescence properties
The luminescent properties of the 4-AphaH ligand and complexes 1 to 4 were investigated in the solid state at room temperature (Fig. 5 and S6†). Excited at 354 nm, the 4-AphaH ligand shows weak photoluminescence emission at 425 nm. Upon excitation at 368 nm, complex 1 exhibits a red-shift of 43 nm compared with the 4-AphaH ligand, with the maximum peaks at 468 nm. However, excited at 370 nm, complex 2 shows red-shift of 39 nm compared with the 4-AphaH ligand, with the maximum peaks at 464 nm. No luminescent property was observed for complex 3. Upon excitation at 364 nm, complex 4 exhibits a red-shift of 31 nm compare with the 4-AphaH ligand, with the maximum peaks at 456 nm. Compared with 4-AphaH, the red-shifted emission of 1, 2 and 4 may be ascribed as the intraligand (π–π*) fluorescent emission because similar emissions were observed for the 4-AphaH ligand at 428 nm.12,22 The pronounced fluorescence emissions of these complexes indicate their potential applications for photoactive materials.
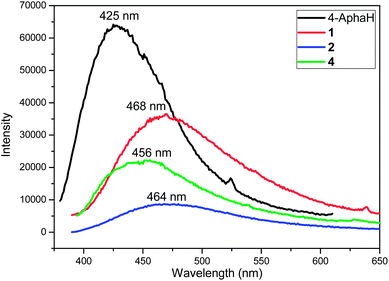 |
| Fig. 5 Room temperature emission spectra for 4-AphaH, 1, 2 and 4 in the solid state (emission slit 1 nm). | |
Thermal analysis
The thermal stability of 1–4 was investigated using thermogravimetric analysis. As can be seen from Fig. S7–10,† the first weight loss of 2 took place between 92.0 °C and 115.4 °C (9.09%), which corresponds to the loss of all lattice and coordinated water molecules (calculated: 8.92%). Above 182.4 °C, the structure began to collapse and decompose. The first weight loss of 4 took place between 57.8 °C and 97.5 °C (6.89%), which corresponds to the loss of all lattice methanol molecules and coordinated water molecules (calculated 6.92%). Above 172.9 °C, the structure began to collapse and decompose. Complexes 1 and 3 were collapsed and decomposed from 213.5 °C and 196.3 °C, respectively.
Conclusions
In summary, the CdII and ZnII coordination complexes based on 4-aminophenylhydroxamic acid (4-AphaH) were reported. One 1-D CdII chain complex, one dinuclear ZnII compound, and two ZnII coordination polymers with different 3-D network structures have been prepared and characterized. The solvents played vital roles in the control of the dimensionality of 2–4, as well as the crystal packing structures of 2 and 4. The strategy of rational assembly of the structures via choosing different solvents provides an efficient approach to ZnII coordination polymers. The luminescent properties of the free ligand, 1, 2 and 4 have been determined; the pronounced fluorescence emissions of 1, 2 and 4 reveal their potential applications for photoactive materials.
Acknowledgements
The authors appreciate the financial support from Natural Science Foundation of China (21272167 and 21201127), A Project Funded by the Priority Academic Program Development of Jiangsu Higher Education Institution, Graduate Education Innovation Project in Jiangsu Province (CXZZ12_0808), and Qinghai Science & Technology Department of China (2011-G-208 and 2011-Z-722).
References
- R. Codd, Coord. Chem. Rev., 2008, 252, 1387 CrossRef CAS PubMed.
-
(a) C. J. Marmion, D. Griffith and K. B. Nolan, Eur. J. Inorg. Chem., 2004, 15, 3003 CrossRef;
(b) C. J. Marmion, T. Murphy, J. R. Docherty and K. B. Nolan, Chem. Commun., 2000, 1153 RSC;
(c) M. J. Miller, Chem. Rev., 1989, 89, 1563 CrossRef CAS.
-
(a) M. A. Pearson, L. O. Nickel, R. P. Hausinger and P. A. Karplus, Biochemistry, 1997, 36, 8164 CrossRef CAS PubMed;
(b) A. J. Stemmler, J. W. Kampf, M. L. Kirk and V. L. Pecoraro, J. Am. Chem. Soc., 1995, 117, 6368 CrossRef CAS;
(c) S. Ciurli, S. Benini, W. R. Rypniewski, K. S. Wilson, S. Miletti and S. Mangani, Coord. Chem. Rev., 1999, 331, 190 Search PubMed.
-
(a) I. M. Rio-Echevarria, F. J. White, E. K. Brechin, P. A. Tasker and S. G. Harris, Chem. Commun., 2008, 4570 RSC;
(b) Z. Tomkowicz, S. Ostrovsky, H. Müller-Bunz, A. J. Hussein Eltmimi, M. Rams, D. A. Brown and W. Haase, Inorg. Chem., 2008, 47, 6956 CrossRef CAS PubMed.
-
(a) Y. Cao, Y. Chen, L. Li, D. Gao, W. Liu, H. Hu, W. Li and Y. Li, Dalton Trans., 2013, 42, 10912 RSC;
(b) D. Gaynor, Z. A. Starikova, S. Ostrovsky, W. Haase and K. B. Nolan, Chem. Commun., 2002, 506 RSC;
(c) J. A. Johnson, J. W. Kampf and V. L. Pecoraro, Angew. Chem., Int. Ed., 2003, 42, 546 CrossRef CAS PubMed;
(d) C. McDonald, T. Whyte, S. M. Taylor, S. Sanz, E. K. Brechin, D. Gaynor and L. F. Jones, CrystEngComm, 2013, 15, 6672 RSC.
-
(a) C. M. Zaleski, E. C. Depperman, J. W. Kampf, M. L. Kirk and V. L. Pecoraro, Angew. Chem., Int. Ed., 2004, 43, 3912 CrossRef CAS PubMed;
(b) J. Jankolovits, C. M. Andolina, J. W. Kampf, K. N. Raymond and V. L. Pecoraro, Angew. Chem., Int. Ed., 2011, 50, 9660 CrossRef CAS PubMed;
(c) T. T. Boron, J. W. Kampf and V. L. Pecorao, Inorg. Chem., 2010, 49, 9104 CrossRef CAS PubMed;
(d) C. M. Zaleski, J. W. Kampf, T. Mallah, M. L. Kirk and V. L. Pecoraro, Inorg. Chem., 2007, 46, 1954 CrossRef CAS PubMed;
(e) A. D. Cutland, R. G. Malkani, J. W. Kampf and V. L. Pecoraro, Angew. Chem., Int. Ed., 2000, 39, 2689 CrossRef CAS;
(f) A. D. Cutland, J. A. Halfen, J. W. Kampf and V. L. Pecoraro, J. Am. Chem. Soc., 2001, 123, 6211 CrossRef CAS;
(g) C. S. Lim, J. W. Kampf and V. L. Pecoraro, Inorg. Chem., 2009, 48, 5224 CrossRef CAS PubMed;
(h) A. D. Cutland-Van Noord, J. W. Kampf and V. L. Pecoraro, Angew. Chem., Int. Ed., 2002, 41, 4668 CrossRef PubMed;
(i) C. M. Zaleski, A. D. Cutland-Van Noord, J. W. Kampf and V. L. Pecoraro, Cryst. Growth Des., 2007, 7, 1098 CrossRef CAS;
(j) A. J. Stemmler, J. W. Kampf, M. L. Kirk, B. H. Atasi and V. L. Pecoraro, Inorg. Chem., 1999, 38, 2807 CrossRef CAS PubMed;
(k) M. Tegoni, M. Furlotti, M. Tropiano, C. S. Lim and V. L. Pecoraro, Inorg. Chem., 2010, 49, 5190 CrossRef CAS PubMed;
(l) C. S. Lim, J. Jankolovits, P. Zhao, J. W. Kampf and V. L. Pecoraro, Inorg. Chem., 2011, 50, 4832 CrossRef CAS PubMed;
(m) C. M. Zaleski, C. S. Lim, A. D. C. V. Noord, J. W. Kampf and V. L. Pecoraro, Inorg. Chem., 2011, 50, 7707 CrossRef CAS PubMed;
(n) J. T. Grant, J. Jankolovits and V. L. Pecoraro, Inorg. Chem., 2012, 51, 8034 CrossRef CAS PubMed;
(o) J. Jankolovits, C. S. Lim, G. Mezei, J. W. Kampf and V. L. Pecoraro, Inorg. Chem., 2012, 51, 4527 CrossRef CAS PubMed;
(p) C. S. Lim, M. Tegoni, T. Jakusch, J. W. Kampf and V. L. Pecoraro, Inorg. Chem., 2012, 51, 11533 CrossRef CAS PubMed;
(q) J. Jankolovits, J. W. Kampf and V. L. Pecoraro, Inorg. Chem., 2013, 52, 5063 CrossRef CAS PubMed;
(r) J. Jankolovits, J. W. Kampf and V. L. Pecoraro, Polyhedron, 2013, 52, 491 CrossRef CAS PubMed;
(s) J. Jankolovits, A. D. C. Van-Noord, J. W. Kampf and V. L. Pecoraro, Dalton Trans., 2013, 42, 9803 RSC;
(t) B. Ruggiero, M. Vivarelli, A. Gianviti, E. Benetti, L. Peruzzi, G. Barbano, F. Corona, G. Ventura, C. Pecoraro, L. Murer, G. M. Ghiggeri, M. Pennesi, A. Edefonti, R. Coppo and F. Emma, Nephrol., Dial., Transplant., 2013, 28, 1487 CrossRef CAS PubMed.
- M. Gajewska, K. V. Luzyanin, M. F. C. Guedes da Silva, Q. S. Li, J. R. Cui and A. J. L. Pombeiro, Eur. J. Inorg. Chem., 2009, 3765 CrossRef CAS.
-
(a) D. Gaynor, Z. A. Starikova, W. Haase and K. B. Nolan, J. Chem. Soc., Dalton Trans., 2001, 1578 RSC;
(b) A. B. M. Alagha, D. Gaynor, H. Muller-Bunz, K. B. Nolan and L. Parthasarathi, Acta Crystallogr., Sect. E: Struct. Rep. Online, 2010, 66, m853 CAS.
-
(a) X. M. Shang, J. Z. Wu and Q. S. Li, Eur. J. Inorg. Chem., 2006, 4143 CrossRef CAS;
(b) C. Mulcahy, F. M. Dolgushin, K. A. Krot, D. Griffith and C. J. Marmion, Dalton Trans., 2005, 1993 RSC.
-
R. E. P. Winpenny, in Comprehensive Coordination Chemistry II, ed. J. A. McCleverty and T. J. Meyer, Elsevier, Amsterdam, 2004, vol. 7, pp. 125–175 Search PubMed.
-
(a) Z. Chen, D. Luo, M. Kang and Z. Lin, Inorg. Chem., 2011, 50, 4674 CrossRef CAS PubMed;
(b) S. Q. Bai, E. Q. Gao, Z. He, C. J. Fang and C. H. Yan, CrystEngComm, 2004, 6, 606 RSC;
(c) J. A. Gould, J. T. A. Jones, J. Bacsa, Y. Z. Khimyak and M. J. Rosseinsky, Chem. Commun., 2010, 2793 RSC.
-
(a) S. L. Zheng and X. M. Chen, Aust. J. Chem., 2004, 57, 703 CrossRef CAS;
(b) X. Li, R. Cao, W. Bi, D. Yuan and D. Sun, Eur. J. Inorg. Chem., 2005, 3156 CrossRef CAS;
(c) Y. Bai, J. L. Wang, D. B. Dang, M. M. Li and J. Y. Niu, CrystEngComm, 2012, 14, 1575 RSC.
- G. Yu, S. Yin, Y. Liu, Z. Shuai and D. Zhu, J. Am. Chem. Soc., 2003, 125, 14816 CrossRef CAS PubMed.
-
(a) C. F. Lee, K. F. Chin, S. M. Peng and C. M. Che, J. Chem. Soc., Dalton Trans., 1993, 467 RSC;
(b) Y. Ma, H. Y. Chao, Y. Wu, S. T. Lee, W. Y. Yu and C. M. Che, Chem. Commun., 1998, 22, 2491 RSC;
(c) Y. Ma, T. Lai and Y. Wu, Adv. Mater., 2000, 12, 433 CrossRef CAS.
-
(a) A. Erxleben, Coord. Chem. Rev., 2003, 246, 203 CrossRef CAS;
(b) P. Jiang and Z. J. Guo, Coord. Chem. Rev., 2004, 248, 205 CrossRef CAS PubMed;
(c) S. N. Wang, Coord. Chem. Rev., 2001, 215, 79 CrossRef CAS.
-
(a) D. A. Brown, W. Errington, N. J. Fitzpatrick, W. K. Glass, T. J. Kemp, H. Nimir and Á. T. Ryan, Chem. Commun., 2002, 1210 RSC;
(b) D. A. Brown, N. J. Fitzpatrick, H. Müller-Bunz and Á. T. Ryan, Inorg. Chem., 2006, 45, 4497 CrossRef CAS PubMed;
(c) T. Tekeste and H. Vahrenkamp, Inorg. Chim. Acta, 2007, 360, 1523 CrossRef CAS PubMed.
-
(a) X. Meng, Y. Song, H. Hou, H. Han, B. Xiao, Y. Fan and Y. Zhu, Inorg. Chem., 2004, 43, 3528 CrossRef CAS PubMed;
(b) S. Biswas, M. Maes, A. Dhakshinamoorthy, M. Feyand, D. E. De Vos, H. Garcia and N. Stock, J. Mater. Chem., 2012, 22, 10200 RSC.
- M. Alexiu, C. Dendrinou-Samara, C. P. Raptopoulou, A. Terzis and D. P. Kessissoglou, Inorg. Chem., 2002, 41, 4732 CrossRef PubMed.
-
G. M. Sheldrick, SHELXS–97, Program for Crystal Structure Solution, University of Göttingen, Germany, 1997 Search PubMed.
-
G. M. Sheldrick, SHELXL–97, Program for the Refinement of Crystal Structures from Diffraction Data, University of Göttingen, Germany, 1997 Search PubMed.
- V. A. Blatov, Nanocluster analysis of intermetallic structures with the program package TOPOS, Struct. Chem., 2012, 23, 955 CrossRef CAS PubMed.
-
(a) J. H. Yang, W. Li, S. L. Zheng, Z. L. Huang and X. M. Chen, Aust. J. Chem., 2003, 56, 1175 CrossRef CAS;
(b) S. L. Zheng, J. P. Zhang, X. M. Chen, Z. L. Huang, Z. Y. Lin and W. T. Wong, Chem.–Eur. J., 2003, 9, 3888 CrossRef CAS PubMed;
(c) S. L. Zheng, J. H. Yang, X. L. Yu, X. M. Chen and W. T. Wong, Inorg. Chem., 2004, 43, 830 CrossRef CAS PubMed;
(d) L. L. Wen, D. B. Dang, C. Y. Duan, Y. Z. Li, Z. F. Tian and Q. J. Meng, Inorg. Chem., 2005, 44, 7161 CrossRef CAS PubMed.
Footnote |
† Electronic supplementary information (ESI) available: Tables of selected bond lengths and angles, and additional figures of these compounds. CCDC 882293, 948225, 948226, and 948228 for complexes 1 to 4, respectively. For crystallographic data in CIF or other electronic format see DOI: 10.1039/c3ra44489a |
|
This journal is © The Royal Society of Chemistry 2014 |
Click here to see how this site uses Cookies. View our privacy policy here.