DOI:
10.1039/C4CC08343A
(Communication)
Chem. Commun., 2015,
51, 133-136
Intramolecular annulation of aromatic rings with N-sulfonyl 1,2,3-triazoles: divergent synthesis of 3-methylene-2,3-dihydrobenzofurans and 3-methylene-2,3-dihydroindoles†
Received
23rd October 2014
, Accepted 4th November 2014
First published on 4th November 2014
Abstract
The controllable synthesis of 3-methylene-2,3-dihydrobenzofurans 2 and 3-methylene-2,3-dihydroindoles 5 has been developed through Rh-catalyzed intramolecular annulation of aromatic rings with azavinyl carbenes.
Benzofuran and indole containing molecules are very important heterocycles which can serve as versatile building blocks and are found in a number of natural products with a wide range of biological activities.1,2 Not surprisingly, numerous efforts have been made to synthesize such compounds.3,4 On the other hand, less attention has been paid to the chemoselective synthesis of 3-methylene-2,3-dihydrobenzofurans and 3-methylene-2,3-dihydroindoles, which are also very important due to the fact that the exocyclic double bonds can be easily transformed to other useful functional groups for rapid construction of molecular complexity. The challenge relies on the 1,3-H shift driven by the aromatization process. Therefore, many reported synthetic methods showed no chemoselectivity in the synthesis of such exo-methylene heterocyclic compounds, or suffered from very limited substrate scopes.5 Only one selective synthesis of both 3-methylene-2,3-dihydrobenzofurans and 3-methylene-2,3-dihydroindoles was accomplished by means of the cycloisomerization of dienes in the presence of Ru complex and trimethylsilyl vinyl ether.6 Therefore, developing new methodologies to chemoselectively construct such compounds is highly desirable and urgent.
Recently, the Rh(II) catalyzed ring-opening reactions of N-sulfonyl 1,2,3-triazoles7 have emerged as a powerful tool for many useful transformations, including annulation with unsaturated compounds,8 X–H bond insertion or functionalization (X = heteroatoms or carbon),9 ring expansion or carbene induced other transformations.10 Previously, we also developed the intramolecular annulation of carbonyl-triazoles for the facile construction of 8-aza bridged benzodioxepines through Rh azavinyl carbene intermediates (Scheme 1a).11 Herein, we wish to report the substrate-dependent divergent synthesis of 3-methylene-2,3-dihydrobenzofurans and 3-methylene-2,3-dihydroindoles (Scheme 1b).
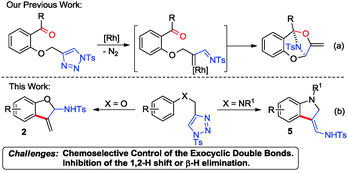 |
| Scheme 1 Intramolecular annulations of triazoles. | |
Our initial work started with the annulation reaction of 4-(phenyloxymethyl)-1-tosyl-1,2,3-triazole 1a, which was readily available from Cu(I)-catalyzed azide–alkyne cycloaddition (CuAAC),12 in the presence of Rh2(Piv)4 catalyst. To our delight and surprise, an unprecedented 3-methylene-2,3-dihydrobenzofuran 2a was obtained and unequivocally confirmed by X-ray diffraction.13 Moreover, when nitrogen tethered substrates 4 were employed as the substrate, the reaction gave indole derivatives (see Table 3).
After evaluation of the catalysts, solvent effect, temperature and reaction time, we found that 2a could be formed in 95% yield in DCE upon heating at 90 °C for 3 h (see ESI,† Table S1).
With the optimized reaction conditions in hand, we next investigated the generality of this method with respect to various 4-(aryloxymethyl)-1-tosyl-1,2,3-triazoles 1 (Table 1). As for substrates 1b–1d with electron-withdrawing Cl and Br atoms on the benzene ring, the corresponding products 2b–2d were isolated in 43–72% yield (Table 1, entries 1–3). On the other hand, upon variation of the substituents by different electron-donating groups, the reactions also proceeded smoothly to give the corresponding 2-aminobenzofuran derivatives 2e–2j in moderate to excellent yield (65–99%) (Table 1, entries 4–9). However, when a strongly electron-withdrawing CN group was introduced at the para position of the phenyl ring, the reaction became sluggish to give complex product mixtures, and no desired product 2k was observed (Table 1, entry 10). Next, several substrates with 4-naphthyloxymethyl substituted 1l–1o were investigated under the standard reaction conditions, delivering the corresponding 3-methylenenaphthofuran derivatives 2l–2o in 72–85% yield (Table 1, entries 11–14). For 7-hydroxycoumarin derived N-sulfonyl triazole 1p, the reaction also proceeded smoothly to give the desired product 2p in 61% yield (Table 1, entry 15).
Table 1 Scope of the reaction for the synthesis of 2a

|
0.2 mmol scale. Reaction conditions: under Ar, triazole, Rh2(Piv)4 and DCE (2.0 mL) were stirred in a sealed tube at 90 °C for 3 h.
Isolated yields.
The reaction temperature was decreased to 80 °C to avoid the formation of 1,3-H shift product 3.
|
|
During the reaction scope investigation, we found that in some cases (see Table 1, substrates 1i and 1l–1o), the temperature has to be decreased to 80 °C to get pure product 2, and higher temperature (90 °C) resulted in the 1,3-H shift relevant 3, which could not be isolated from 2. The structure was also confirmed by X-ray diffraction of 3e.13 We were pleased to find that products 3a–3e could be exclusively formed at 120 °C in moderate to good yield (50–88%) (Table 2, entries 1–5). However, for most of 4-aryloxymethyl-1,2,3-triazoles, conducting the reaction at 120 °C led to the formation of both 2 and 3 together with increased β-H elimination byproduct acrolein imines, suggesting that temperature significantly affects the reaction pathways. Fortunately, by carefully tuning the temperature, the reactions of 1g and 1i proceeded smoothly at 120 °C and 90 °C, furnishing the corresponding products 3f and 3g in 64% and 71% yield, respectively (Table 2, entry 6).
Table 2 Rearrangement of 1 at high temperaturea

|
0.2 mmol scale. Procedure: under Ar, triazole, Rh2(Piv)4 and DCE (2.0 mL) were stirred in a sealed tube at 120 °C for 3 h.
Isolated yields.
Starting material is 1q.
The reaction temperature was 90 °C.
|
|
Next, we also turned our effort to study the reaction of N-tethered substrate 4. However, upon treatment of N-tosyl tethered substrate 4a with Rh2(Piv)4 at 90 °C in DCE for 3 h, product 5a14 was obtained in 55% yield, together with the formation of indolyl imine product 6a′ (β-H elimination) and acrolein imine 7a (carbene induced 1,2-H shift) in 30% and 11% yield, respectively (Scheme 2). The identification of 6a′13 and 7a13 was established by single-crystal X-ray analysis. It is reported that LiCl could suppress β-H elimination by coordination with metal catalyst.15 Therefore, 5 eq. of LiCl was used in the reaction. By conducting the reaction at 60 °C, we were glad to find that 5a was exclusively formed in 82% yield (Scheme 2).
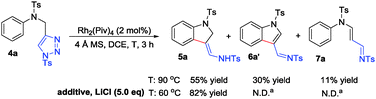 |
| Scheme 2 Inhibition of β-H elimination/1,2-H shift. a N.D.: not determined. | |
As we successfully solved the problem of β-H elimination and 1,2-H shift, various N-sulfonyl tethered triazoles were synthesized to test the generality of this reaction (Table 3). All substrates employed were suitable for this cyclization reaction, giving the corresponding products 5 in 41–71% yield (Table 3, entries 1–6). Interestingly, when substrate 4h with 3,5-dimethoxy substituents was treated under the standard reaction conditions, indolyl methanamine product 6a was obtained in 85% yield after aromatization (Table 3, entry 7). It is worth mentioning that gram scale reaction of 4b also gives 5b in 65% yield (608 mg) (Table 3, entry 1).
Table 3 Scope of the reaction for the synthesis of 5a
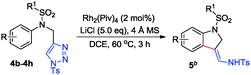
|
0.2 mmol scale.
Isolated yields.
2 mmol scale; 992 mg of 4i and 608 mg of 5b were obtained.
|
|
For N-alkyl group tethered triazoles, the reactions gave 3-indolylimines 6′ and 3-indolylmethanamines 6 together, and finally, the mixture could be converted to 3-indolylmethanamines 6 after a one-pot reduction (Scheme 3) (for the substrate scope study, see Table S2 in the ESI†).16
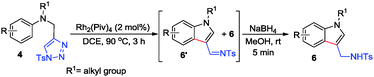 |
| Scheme 3 One-pot synthesis of 6. | |
Several control experiments were conducted and carbene induced 1,2-H shift or 1,2-phenyl migration was observed (for details, see Scheme S1 in the ESI†). The deuterium labeling isotopic experiments revealed that the alkyl methylene carbon migrates to the terminal alkene in the final products (for details, see Scheme S2 in ESI†).
The deuterium kinetic isotope effects were also investigated. The obtained intra- and intermolecular kH/kD values were 0.89 and 0.77 (for details, see ESI†), respectively, suggesting that C–H functionalization is a Friedel–Crafts type reaction.17 This result is also consistent with the electronic effect observed in the substrate scope study.
In view of the control experiments and kinetic studies, an explanation of the reaction sequence is depicted in Scheme 4. First, in the presence of Rh(II) catalyst, an azavinyl carbene intermediate A is formed after denitrogenation, followed by a Friedel–Crafts type nucleophilic attack of the aryl ring on the Rh azavinyl carbenoid to give a zwitterionic intermediate B. The elimination of the Rh(II) catalyst together with the cleavage of the C–O bond delivers an acrolein imine C and its resonance structure C′. Finally, product 2 is formed via 1,2-addition, which is more favored than 1,4-addition probably due to the fact that the intramolecular H bonding can pull the imine group more closely to the oxygen atom. Upon heating, the thermodynamically more stable product 3 is formed after 1,3-H shift.
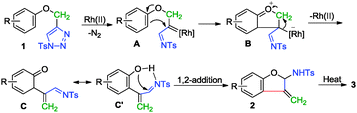 |
| Scheme 4 Proposed catalytic cycle for the synthesis of 2 and 3. | |
A plausible mechanism for the synthesis of 5 is also outlined in Scheme 5. First, in the presence of Rh(II) complex, compound 4 generates an azavinyl carbene intermediate D, and then the Rh imino carbenoid accepts a nucleophilic attack from the aryl ring to give zwitterionic intermediate E. After aromatization and protonation, product 5 is formed. In the case of substrate 4h with two strongly electron-donating groups on the benzene ring, 6b is obtained after the 1,3-H shift.
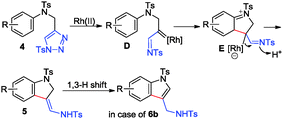 |
| Scheme 5 Proposed catalytic cycle for the formation of 5. | |
According to the DFT calculation, the 1,3-H shift process of 5a needs a relatively high energy barrier of 67.1 kcal mol−1, indicating that this 1,3-H shift process is hard to take place under normal reaction conditions, which may account for why only product 5a is obtained (for details, see Fig. S1 in ESI†).
The gram scale reaction of 1a was conducted and 2a was obtained in 90% yield (for details, see Scheme S3 in the ESI†). It was also found that 2a could be readily converted to the corresponding ketone and indoline after ozonization and hydrogenation (for details, see Scheme S4 in the ESI†). Other aromatic systems, such as indoles and pyroles, will be investigated and reported in due course.
Furthermore, upon treatment of 2a with 2 equiv. of m-nitrobenzaldehyde in the presence of BF3·Et2O (1.1 equiv.), a 3,3′-spirobi[benzofuran]2-one derived imine 818 was isolated in 52% yield (Scheme 6) and its structure was confirmed by X-ray diffraction.13 Notably, the 3,3′-spirobi[benzofuran]-2-one structure is found to exist in a natural product family of phenolics (Yuccaol A–E and Larixinol), which are extracted from Yucca schidigera Roezl and have been used in folk medicine, food and pharmaceutical industries.19
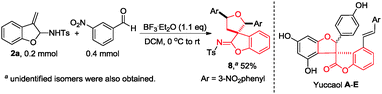 |
| Scheme 6 Further derivatization of 2a to synthesize imine 8. | |
In conclusion, the controllable and chemoselective synthesis of 3-methylene-2,3-dihydrobenzofurans and 3-methylene-2,3-dihydroindoles has been established through two types of annulations of aromatic rings with N-sulfonyl 1,2,3-triazoles. For benzofuran synthesis, temperature plays an important role in controlling the exocyclic double bond and intramolecular hydrogen bonding facilitates the 1,2-addition. On the other hand, in indole synthesis, the big challenges are the β-H elimination and 1,2-H shift, which are successfully solved by the combination of additive (LiCl) and temperature, and this finding may be helpful for those reactions in which the triazole ring has adjacent beta-protons. Several derivatizations of 2a were conducted to access biologically and medicinally valuable molecules, such as the analog of phenolics. Further investigations to examine the mechanistic details more extensively are currently underway in our laboratory.
We are grateful for the financial support from the National Basic Research Program of China (973)-2015CB856603, the Shanghai Municipal Committee of Science and Technology (11JC1402600), and the National Natural Science Foundation of China (20472096, 21372241, 21361140350, 20672127, 21102166, 21121062, 21302203 and 20732008).
Notes and references
-
(a) Y. Liu, M. Kubo and Y. Fukuyama, J. Nat. Prod., 2012, 75, 2152 CrossRef CAS PubMed;
(b) T. Nagahara, Y. Yokoyama, K. Inamura, S. Katakura, S. Komoriya, H. Yamaguchi, T. Hara, D. Argentieri and W. Hagenman, J. Med. Chem., 1994, 37, 1200 CrossRef CAS;
(c) Z. Yang, H. B. Liu, C. M. Lee, H. M. Chang and H. N. C. Wong, J. Org. Chem., 1992, 57, 7248 CrossRef CAS.
-
(a)
R. J. Sundberg, The Chemistry of Indoles, Academic Press, New York, 1970 Search PubMed;
(b)
R. J. Sundberg, Indoles, Academic Press, San Diego, 1996 Search PubMed;
(c)
R. H. Thomson, The Chemistry of Natural Products, Blackie and Son, Glasgow, 1985 Search PubMed;
(d) F.-E. Chen and J. Huang, Chem. Rev., 2005, 105, 4671 CrossRef CAS PubMed.
- For selected examples, see:
(a) A. Fürstner and P. W. Davies, J. Am. Chem. Soc., 2005, 127, 15024 CrossRef PubMed;
(b) I. Nakamura, Y. Mizushima and Y. Yamamoto, J. Am. Chem. Soc., 2005, 127, 15022 CrossRef CAS PubMed;
(c) D.-H. Lee, K.-H. Kwon and C. S. Yi, J. Am. Chem. Soc., 2012, 134, 7325 CrossRef CAS PubMed;
(d) T. J. Maimone and S. L. Buchwald, J. Am. Chem. Soc., 2012, 134, 7325 CrossRef PubMed.
- For selected examples, see:
(a) Y. Wei, I. Deb and N. Yoshikai, J. Am. Chem. Soc., 2012, 134, 9098 CrossRef CAS PubMed;
(b) S. G. Newman and M. Lautens, J. Am. Chem. Soc., 2010, 132, 11416 CrossRef CAS PubMed;
(c) S. Wagaw, B. H. Yang and S. L. Buchwald, J. Am. Chem. Soc., 1998, 120, 6621 CrossRef CAS;
(d) M. C. Willis, G. N. Brace and I. P. Holmes, Angew. Chem., Int. Ed., 2005, 44, 403 CrossRef CAS PubMed;
(e) B. J. Stokes, H. Dong, B. E. Leslie, A. L. Pumphrey and T. G. Driver, J. Am. Chem. Soc., 2007, 129, 7500 CrossRef CAS PubMed.
- For selected examples, see:
(a) Y. Wang and A. Zhang, Tetrahedron, 2009, 65, 6986 CrossRef CAS PubMed;
(b) K. Sasaki, Y. Kondo and K. Maruoka, Angew. Chem., Int. Ed., 2001, 40, 411 CrossRef CAS;
(c) A.-L. Girard, R. Lhermet, C. Fressigné, M. Durandetti and J. Maddaluno, Eur. J. Org. Chem., 2012, 2895 CrossRef CAS;
(d) M. Durandetti, L. Hardou, R. Lhermet, M. Rouen and J. Maddaluno, Chem. – Eur. J., 2011, 17, 12773 CrossRef CAS PubMed;
(e) S. N. Osipov, N. M. Kobel'kova, A. F. Kolomiets, K. Pumpor, B. Koksch and K. Burger, Synlett, 2001, 1287 CrossRef CAS PubMed;
(f) Q. Liu, B. Han, W. Zhang, L. Yang, Z.-L. Liu and W. Yu, Synlett, 2005, 2248 CrossRef CAS;
(g) B. Burns, R. Grigg, V. Sridharan, P. Stevenson, S. Sukirthalingam and T. Worakun, Tetrahedron Lett., 1989, 30, 1135 CrossRef CAS.
-
(a) Y. Terada, M. Arisawa and A. Nishida, Angew. Chem., Int. Ed., 2004, 43, 4063 CrossRef CAS PubMed;
(b) M. Arisawa, Y. Terada, K. Takahashi, M. Nakagawa and A. Nishida, J. Org. Chem., 2006, 71, 4255 CrossRef CAS PubMed.
- For leading reviews, see:
(a) B. Chattopadhyay and V. Gevorgyan, Angew. Chem., Int. Ed., 2012, 51, 862 CrossRef CAS PubMed;
(b) A. V. Gulevich and V. Gevorgyan, Angew. Chem., Int. Ed., 2013, 52, 1371 CrossRef CAS PubMed;
(c) H. M. L. Davies and J. S. Alford, Chem. Soc. Rev., 2014, 5151 RSC . For pioneering examples, see: ;
(d) T. Horneff, S. Chuprakov, N. Chernyak, V. Gevorgyan and V. V. Fokin, J. Am. Chem. Soc., 2008, 130, 14972 CrossRef CAS PubMed;
(e) T. Miura, M. Yamauchi and M. Murakami, Chem. Commun., 2009, 1470 RSC;
(f) J. S. Alford and H. M. L. Davies, Org. Lett., 2012, 14, 6020 CrossRef CAS PubMed.
-
(a) S. Chuprakov, S. W. Kwok, L. Zhang, L. Lercher and V. V. Fokin, J. Am. Chem. Soc., 2009, 131, 18034 CrossRef CAS PubMed;
(b) N. P. Grimster, L. Zhang and V. V. Fokin, J. Am. Chem. Soc., 2010, 132, 2510 CrossRef CAS PubMed;
(c) J. C. Culhane and V. V. Fokin, Org. Lett., 2011, 13, 4578 CrossRef CAS PubMed;
(d) M. Zibinsky and V. V. Fokin, Org. Lett., 2011, 13, 4870 CrossRef CAS PubMed;
(e) B. T. Parr and H. M. L. Davies, Angew. Chem., Int. Ed., 2013, 52, 10044 CrossRef CAS PubMed;
(f) J. S. Alford, J. E. Spangler and H. M. L. Davies, J. Am. Chem. Soc., 2013, 135, 11712 CrossRef CAS PubMed;
(g) S. W. Kwok, L. Zhang, N. P. Grimster and V. V. Fokin, Angew. Chem., Int. Ed., 2014, 53, 3452 CrossRef CAS PubMed;
(h) H. Shang, Y. Wang, Y. Tian, J. Feng and Y. Tang, Angew. Chem., Int. Ed., 2014, 53, 5662 CrossRef CAS PubMed;
(i) M. Zibinsky and V. V. Fokin, Angew. Chem., Int. Ed., 2013, 52, 1507 CrossRef CAS PubMed;
(j) T. Miura, T. Tanaka, K. Hiraga, S. G. Stewart and M. Murakami, J. Am. Chem. Soc., 2013, 135, 13652 CrossRef CAS PubMed;
(k) B. Chattopadhyay and V. Gevorgyan, Org. Lett., 2011, 13, 3746 CrossRef CAS PubMed;
(l) Y. Shi and V. Gevorgyan, Org. Lett., 2013, 15, 5394 CrossRef CAS PubMed;
(m) E. E. Schultz and R. Sarpong, J. Am. Chem. Soc., 2013, 135, 4696 CrossRef CAS PubMed;
(n) S. Chuprakov, S. W. Kwok and V. V. Fokin, J. Am. Chem. Soc., 2013, 135, 4652 CrossRef CAS PubMed;
(o) J. E. Spangler and H. M. L. Davies, J. Am. Chem. Soc., 2013, 135, 6802 CrossRef CAS PubMed;
(p) B. T. Parr, S. A. Green and H. M. L. Davies, J. Am. Chem. Soc., 2013, 135, 4716 CrossRef CAS PubMed;
(q) Y. Xing, G. Sheng, J. Wang, P. Lu and Y. Wang, Org. Lett., 2014, 16, 1244 CrossRef CAS PubMed;
(r) E. E. Schultz, V. N. G. Lindsay and R. Sarpong, Angew. Chem., Int. Ed., 2014, 53, 9904 CrossRef CAS PubMed;
(s) J. S. Alford and H. M. L. Davies, J. Am. Chem. Soc., 2014, 136, 10266 CrossRef CAS PubMed;
(t) X. Ma, S. Pan, H. wang and W. Chen, Org. Lett., 2014, 16, 4554 CrossRef CAS PubMed;
(u) D. J. Lee, H. S. Han, J. Shin and E. J. Yoo, J. Am. Chem. Soc., 2014, 136, 11606 CrossRef CAS PubMed;
(v) S. Rajasekar and P. Anbarasan, J. Org. Chem., 2014, 79, 8428 CrossRef CAS PubMed;
(w) R.-Q. Ran, J. He, S.-D. Xiu, K.-B. Wang and C.-Y. Li, Org. Lett., 2014, 16, 3704 CrossRef CAS PubMed;
(x) F. Medina, C. Besnard and J. Lacour, Org. Lett., 2014, 16, 3232 CrossRef CAS PubMed;
(y) C.-E. Kim, S. Park, D. Eom, B. Seo and P. H. Lee, Org. Lett., 2014, 16, 1900 CrossRef CAS PubMed.
-
(a) T. Miura, T. Biyajima, T. Fujii and M. Murakami, J. Am. Chem. Soc., 2012, 134, 194 CrossRef CAS PubMed;
(b) T. Miura, T. Tanaka, T. Biyajima, A. Yada and M. Murakami, Angew. Chem., Int. Ed., 2013, 52, 3883 CrossRef CAS PubMed;
(c) T. Miura, T. Tanaka, A. Yada and M. Murakami, Chem. Lett., 2013, 42, 1308 CrossRef CAS;
(d) S. Chuprakov, B. T. Worrell, N. Selander, R. K. Sit and V. V. Fokin, J. Am. Chem. Soc., 2014, 136, 195 CrossRef CAS PubMed;
(e) S. Chuprakov, J. A. Malik, M. Zibinsky and V. V. Fokin, J. Am. Chem. Soc., 2011, 133, 10352 CrossRef CAS PubMed;
(f) J.-M. Yang, C.-Z. Zhu, X.-Y. Tang and M. Shi, Angew. Chem., Int. Ed., 2014, 53, 5142 CAS;
(g) D. Yadagiri and P. Anbarasan, Org. Lett., 2014, 16, 2510 CrossRef CAS PubMed.
-
(a) N. Selander, B. T. Worrell, S. Chuprakov, S. Velaparthi and V. V Fokin, J. Am. Chem. Soc., 2012, 134, 14670 CrossRef CAS PubMed;
(b) N. Selander and V. V. Fokin, J. Am. Chem. Soc., 2012, 134, 2477 CrossRef CAS PubMed;
(c) T. Miura, Y. Funakoshi, M. Morimoto, T. Biyajima and M. Murakami, J. Am. Chem. Soc., 2012, 134, 17440 CrossRef CAS PubMed;
(d) R. Liu, M. Zhang, G. Winston-McPherson and W. Tang, Chem. Commun., 2013, 49, 4376 RSC;
(e) K. Chen, Z.-Z. Zhu, Y.-S. Zhang, X.-Y. Tang and M. Shi, Angew. Chem., Int. Ed., 2014, 53, 6645 CrossRef CAS PubMed;
(f) T. Miura, Y. Funakoshi, T. Tanaka and M. Murakami, Org. Lett., 2014, 16, 2760 CrossRef CAS PubMed;
(g) T. Miura, T. Nakamuro, K. Hiraga and M. Murakami, Chem. Commun., 2014, 50, 10474 RSC;
(h) A. Boyer, Org. Lett., 2014, 16, 1660 CrossRef CAS PubMed.
- This work has been submitted to Chem. Commun. (manuscript ID: CC-COM-10-2014-008339).
- For selected recent review and example:
(a) J. E. Hein and V. V. Fokin, Chem. Soc. Rev., 2010, 39, 1302 RSC;
(b) B. T. Worrell, J. A. Malik and V. V. Fokin, Science, 2013, 340, 457 CrossRef CAS PubMed.
- The crystal data of 2a, 3e, 6a′, 7a and 8 have been deposited in CCDC with number 966827, 969393, 969179, 1012006 and 989483. For details, see ESI†.
- The configuration of the double bond in product 5 is determined by NOE spectroscopy of 5d. See ESI†.
-
(a) X. Lu, Top. Catal., 2005, 35, 73 CrossRef;
(b) Z. Wang and X. Lu, J. Org. Chem., 1996, 61, 2254 CrossRef CAS;
(c) Z. Wang, Z. Zhang and X. Lu, Organometallics, 2000, 19, 775 CrossRef CAS.
- The results are similar as a previously reported work, see: B. Rajagopal, C.-H. Chou, C.-C. Chung and P.-C. Lin, Org. Lett., 2014, 16, 3752 CrossRef CAS PubMed.
-
(a) Most of the Friedel–Crafts reactions exhibit inverse isotope effects (kH/kD < 1, ∼0.8–0.9), see:L. Melander, Isotope Effect on Reaction Rates, Ronald Press, New York, 1960, pp. 107–123 Search PubMed;
(b)
E. V. Anslyn and D. A. Dougherty, Modern Physical Organic Chemistry, University Science Books, 2006, p. 428 Search PubMed.
- Proposed mechanism for the formation of 8 is presented in the ESI†.
-
(a) S. Piacente, C. Pizza and W. Oleszek, Phytochem. Rev., 2005, 4, 177 CrossRef CAS PubMed;
(b) C. Balestrieri, F. Felice, S. Piacente, C. Pizza, P. Montoro, W. Oleszek, V. Visciano and M. L. Balestrieri, Biochem. Pharmacol., 2006, 71, 1479 CrossRef CAS PubMed;
(c) S. Marzocco, S. Piacente, C. Pizza, W. Oleszek, A. Stochmal, A. Pinto, R. Sorrentino and G. Autore, Life Sci., 2004, 75, 1491 CrossRef CAS PubMed;
(d) E. M. Wenzig, W. Oleszek, A. Stochmal, O. Kunert and R. Bauer, J. Agric. Food Chem., 2008, 56, 8885 CrossRef CAS PubMed.
Footnote |
† Electronic supplementary information (ESI) available: Experimental procedures, characterization data of new compounds, and CCDC 966827, 969393, 969179, 1012006 and 989483. For ESI and crystallographic data in CIF or other electronic format see DOI: 10.1039/c4cc08343a |
|
This journal is © The Royal Society of Chemistry 2015 |
Click here to see how this site uses Cookies. View our privacy policy here.