DOI:
10.1039/C4FO00649F
(Paper)
Food Funct., 2015,
6, 71-82
Optimization of in vitro inhibition of HT-29 colon cancer cell cultures by Solanum tuberosum L. extracts†
Received
21st July 2014
, Accepted 13th October 2014
First published on 15th October 2014
Abstract
Secondary metabolites in potato have been reported to possess bioactive properties, including growth inhibition of cancer cells. Because potatoes are widely consumed globally, potential health benefits may have broad application. Thus we investigated growth inhibition of HT-29 colon cancer cell cultures by extracts from 13 diverse genetic breeding clones. Extracts from three pigmented selections (CO97226-2R/R, CO97216-1P/P, CO04058-3RW/RW) inhibited growth of in vitro HT-29 cell cultures more effectively than other clones tested. While inhibition was highest from pigmented selections and pigmented tuber tissue sectors, not all pigmented breeding lines tested had appreciable inhibitory properties. Thus, inhibition was not uniquely linked to pigmentation. Immature tubers had the highest inhibitory properties, and in most cases mature tubers retained very low inhibition properties. Flowers and skins inhibited strongly at lower extract concentrations. An extract consisting of 7.2 mg mL−1 cell culture medium was the lowest effective concentration. While raw tuber extracts inhibited most effectively, a few clones at higher concentrations retained inhibition after cooking. Heated whole tubers retained higher inhibition than heated aqueous extracts. While all aqueous extracts from the two tuber selections (CO97216-1P/P and CO97226-2R/R) inhibited HT-29 cell cultures, inhibition was significantly enhanced in purple pigmented tubers of CO97216-1P/P prepared cryogenically as liquid nitrogen powders compared to extracts from freeze dried samples. Upregulation of caspase-3 protease activity, indicative of apoptosis, was highest among the most inhibitory clone samples. The unique sectorial red pigment expressing selection (CO04058-3RW/RW) provided a model system that isolated expression in pigmented sectors, and thus eliminated developmental, environmental and genetic confounding.
Introduction
Cancer is an epidemic affecting every region, race, and socioeconomic class across the globe.2 The American Cancer Society2 estimated that by 2030 about 21.4 million new cancer cases will arise globally and 13.2 million of these cases are expected to result in death. Colorectal carcinoma, cancer of the colon and rectum, is the third leading cause of cancer death in both men and women in the United States,3 the second leading cause of cancer death worldwide in women and the third leading cause of cancer death worldwide in men.2 The American Cancer Society estimates that approximately 136
830 new colorectal cancer diagnoses will arise in the US in 2014 and 50
310 people are expected to die of this disease.2 The Globocan Project reported that an estimated 1
361
000 new colorectal cancer cases arose in 2012 worldwide and that an estimated 694
000 individuals died of this potentially preventable disease.12
The WCRF/AICR has found an average preventability of colorectal cancer through a healthy diet, being physically active and maintaining a healthy weight of 45% in the United States, 43% in the United Kingdom, 37% in Brazil and 17% in China.40 The WCRF/AICR has also published the following cancer prevention recommendations: maintain a healthy body weight, be physically active in everyday life, limit consumption of energy dense foods, eat mostly foods of plant origin, limit consumption of red and processed meats, limit alcohol consumption, limit salt intake and sodium processed foods, and eliminate high doses of nutrient supplements.39 A meta-analysis of 19 prospective studies4 did not agree with the recommendation that fruit and vegetable consumption may be beneficial. Their study reported, “a weak but statistically significant nonlinear inverse association between fruit and vegetable intake and colorectal cancer risk”.4 However, many studies over several decades have implicated classes of secondary metabolites in addition to nutritional components found in potato that conferred inhibitory and antiproliferative activity,10,11,14 apoptotic induction,10,17,22,29,41 and capacity to upregulate detoxifying phase 2 enzymes.11 Potentially bioactive classes of metabolites in potato, found by others to induce inhibitory activity in cancer cells in vivo and/or in vitro, include dietary fiber,16 resistant starch,20 vitamin C,24,27 glycoalkaloids,1,13,14,17,22,41 pectin,9 flavonoids,8,15,19,21,29–32,35 and polyphenolic acids.11,25,37 Moreover, potato (S. tuberosum L.) is the third largest consumed staple food crop in the world and is the world's most important non-grain food crop.18 Accordingly, high prevalence of potato consumption worldwide, as well as previous research that suggested bioactivity and possible inhibitory properties against colorectal cancer, rationalized this research as worthy of further investigation.
The main objective of this study was to test the hypothesis that extracts from 13 diverse S. tuberosum L. genotypes contain inhibitory properties against HT-29 colon cancer cell cultures in vitro. Additional goals were to seek insight into possible modes of inhibitory activity and to identify factors that may optimize inhibitory metabolites against HT-29 colon cancer cell cultures in vitro by exploring developmental stages, dosage concentrations, extraction from uncooked and cooked tubers, extraction from various S. tuberosum plant components, exposure of extracts and tubers to possible thermal degradation and sample preparation methodologies.
Experimental protocols
Experimental materials and treatments
Thirteen clones of S. tuberosum, grown under commercial conditions were obtained from the Colorado Potato Breeding Program, Colorado State University San Luis Valley Research Center, Colorado. Clones beginning with CO are in an experimental phase of release and are designated as selections. Clones with names have been released to the public and are designated as cultivars (Table 1). Selections ending in -P/PW, -R/RW and -RW/RW are selections containing pigmented and white sectors of tissue that are otherwise of the same genotype and not confounded by environmental and developmental differences (Fig. 1; Table 1). In all selections, the first letter before the slash is indicative of tuber skin color (P = purple, R = red, W = white, RU = russet) and the letters following the slash are indicative of tuber flesh colors (P = purple, R = red, W = white) (Table 1).
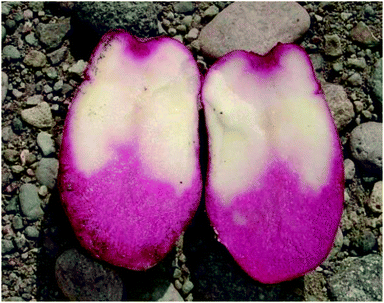 |
| Fig. 1 Sectorial expression of red pigments in CO04058-3RW/RW illustrating a model system that permits sampling of tissues from the same tuber that differ only in pigment expression and are thus not confounded by environmental factors or genetic segregation. | |
Table 1 Cultivars and clonal selections from the Colorado Breeding Program utilized in this research
Cultivars and clonal selections |
Female parent |
Male parent |
Skin |
Flesh |
CO95172-3RU |
Russet Nugget |
AC88165-3 |
Russet |
White |
CO97216-1P/P |
CO94163-1 |
CO94214-1 |
Purple |
Purple |
CO97216-3P/PW |
CO94163-1 |
CO94214-1 |
Purple |
Purple & White |
CO97226-2R/R |
Mountain Rose |
CO94214-1 |
Red |
Red |
CO97227-2P/PW |
Mountain Rose |
CO94215-1 |
Purple |
Purple & White |
CO04056-7P/PW |
CO97216-1P/P |
CO97227-2P/PW |
Purple |
Purple & White |
CO04058-3RW/RW |
CO97216-3P/PW |
CO97216-1P/P |
Red & White |
Red & White |
CO04061-1R/RW |
CO97222-1R/R |
CO97306-1R/R |
Red |
Red & White |
Colorado Rose |
NDTX9-1068-11R |
DT6063-1R |
Red |
White |
Mountain Rose |
All Red |
ND2109-7 |
Red |
Red |
Purple Majesty |
All Blue |
ND2008-2 |
Purple |
Purple |
Rio Grande Russet |
Butte |
A8469-5 |
Russet |
White |
Russet Nugget |
Krantz |
AND71609-1 |
Russet |
White |
Three to five biological replicates (always derived from separate plants and stored immediately following harvest at 4 °C in dark conditions for a short time prior to all preservation processes) from selected cultivars and selections at various extract concentrations were examined for inhibitory activity against HT-29 colon cancer cell cultures for one, two or three consecutive harvests (2008–2011) (Table S5†) as: (1) freeze dried raw potatoes (immature (Stage I, II), intermediate (Stage III, IV), and mature (Stage V) tuber developmental stages) (Table S6†); (2) freeze dried boiled potatoes (97 °C for 20 minutes), (immature (Stage I, II), intermediate (Stage III, IV), and mature (Stage V) tuber developmental stages) (Table S6†); (3) following different sample preparation processes (freeze dried vs. cryogenically ground) raw immature tubers stored for 6 months (Table S6†) prior to extraction in organic solvent; (4) freeze dried plant components (raw and immature potato peel, flesh and whole tubers; and potato flowers) (Table S6†); (5) heated aqueous extracts of freeze dried raw immature tubers compared to aqueous extracts from freeze dried heated immature whole tubers both stored for 6 months (Table S6†).
Extraction from freeze-dried tuber tissues
Three to five biological replicates from median slices of peel and flesh from whole raw tubers, boiled whole tubers, raw potato peels, raw potato flesh and flowers from each clone were freeze dried using a Virtis Genesis 1500 L cabinet style freeze drier. Freeze-dried samples were ground into a fine powder using a mortar and pestle and sifted through a fine sieve (100 mesh). Six hundred mg of sample was added to each 15 mL conical tube with 10 mL HPLC grade 80% acetone, rotated in a refrigerator for 1 hour at 4 °C and centrifuged for 15 minutes at 4 °C and 5000g. The supernatant was transferred to Eppendorf tubes in 1 mL aliquots and vacufuged to dryness at 45 °C in organic mode using a speed vacuum. Dried 60 mg mL−1 potato extracts were stored at −20 °C until analysis for microculture cell viability assays.
Cryogenic extraction
Median slices (2–3 mm thick, approximately 3 g) from three to five raw whole tubers of each biological replicate of CO97226-2R/R and CO97216-1P/P clones were weighed and ground into a very fine powder using a mortar and pestle in liquid nitrogen. Immediately after grinding, 10 mL of HPLC grade 80% acetone was added and the contents transferred to a 15 mL conical tube, rotated in a refrigerator for 1 hour at 4 °C and centrifuged for 15 minutes at 4 °C and 5000g. The supernatant was transferred to Eppendorf tubes in 1 mL aliquots and vacufuged to dryness at 45 °C in organic mode using a speed vacuum. Dried 60 mg mL−1 potato extracts were stored at – 20 °C until analysis for microculture cell viability assays.
Heated extracts
Extracts (60 mg mL−1) from freeze dried powders of CO97226-2R/R and CO97216-1P/P were prepared as described. Dried 60 mg mL−1 potato extracts in 1.5 mL Eppendorf tubes stored at – 20 °C were reconstituted in 1 mL McCoys 5A, 1× medium supplemented with 10% fetal bovine serum under sterile conditions and subjected to six different temperature water baths (22, 37, 45, 55, 72 and 96 °C) for 20 minutes to give the “heated extract” samples. These heated extract samples were assayed for inhibitory activity against HT-29 colon cancer cells in culture immediately after heating and cooling to 25 °C using the microculture tetrazolium assay procedure described below.
Heated tubers
Three biological replicates from tubers of CO97226-2R/R and CO97216-1P/P were stored for 6 months at 4 °C in dark conditions and subjected to 6 different temperature water baths (24, 37, 45, 60, 72 and 96 °C) for 20 minutes. Internal tuber temperatures were recorded every 5 minutes with a copper-constantan thermocouple that was inserted into the center of an indicator tuber to ensure internal tuber temperature coincided with water bath temperature. Heated tubers were freeze-dried and 60 mg mL−1 potato extracts were prepared as above to give the “heated tuber” samples.
Microculture assay
Cell lines and cell cultures.
Human colorectal adenocarcinoma HT-29 cells were obtained from American Type Culture Collection (ATCC, Manassas, Virginia). First generation cells received from ATCC were thawed, grown in flasks to 80% confluence in McCoys 5A, 1× medium containing 10% fetal bovine at 37 °C in a humidified, 5% CO2 incubator chamber and cryogenically preserved in liquid nitrogen vapor at the USDA-ARS National Center for Genetic Resources Preservation (NCGRP, Fort Collins, Colorado) all according to ATCC culture method protocols. Cells used in the execution of all cell culture experiments were maintained according to ATCC protocol for no more than five generations before first generation cells were retrieved from cryopreservation storage.
Cell seeding.
All the following methods were conducted under sterile conditions using sterile materials and a sterile transfer hood. HT-29 colon cells were seeded into a 96-well plate at a rate of 5 × 104 cells in 50 μL McCoys 5A, 1× medium containing 10% fetal bovine per well and incubated at 37 °C in a humidified, 5% CO2 incubator chamber for 24 hours to allow cells to adhere to the plates. Trypan blue was used in a 1
:
1 ratio to distinguish viable cells from nonviable cells. A hemacytometer and inverted microscope were used to facilitate the cell count during this initial seeding step. No wells on the outer perimeter of the microplate were seeded with cells in order to minimize any variation that may occur in these wells.
Potato/plant component extract treatment.
Three to five biological replicates of 60 mg mL−1 whole tuber, potato peel, potato flesh and flower extracts from each clone obtained throughout several developmental stages in both raw and cooked forms were reconstituted in 1 mL of aqueous McCoys 5A, 1× medium containing 10% fetal bovine. Reconstituted 60 mg mL−1 whole tuber extracts were filter sterilized and 50 μL of 7.2, 14.4 and 28.8 mg mL−1 whole tuber extract diluted in McCoys 5A, 1× medium containing 10% fetal bovine was added in triplicate replication to individual wells of a 96 well microplate containing seeded cells to achieve a final treatment extract concentration of 3.6, 7.2 and 14.4 mg mL−1 extracts in cell culture medium. Reconstituted 60 mg mL−1 plant component (potato peel, potato flesh and flower) extracts were filter sterilized and 50 μL of plant component extract concentrations ranging from 0.9 to 28.8 mg mL−1 diluted in McCoys 5A, 1× medium containing 10% fetal bovine was added in triplicate replication to individual wells of a 96 well microplate containing seeded cells to achieve a final range of respective treatment extract concentrations of 0.5 to 14.4 mg mL−1 plant component extract in cell culture medium. All treated 96 well microplates were incubated for 24 hours at 37 °C in a humidified, 5% CO2 incubator chamber to allow for potential inhibitory interactions to occur.
Control.
Nine individual wells containing seeded cells on each microplate were treated with 50 μL of McCoys 5A, 1× medium containing 10% fetal bovine serum and incubated for 24 hours at 37 °C in a humidified, 5% CO2 incubator chamber. Untreated cells were designated as controls for calculating percent growth inhibition of treated cells residing on the same microplate.
Cell viability assay.
Inhibitory activities in all cell culture assays were determined by the colorimetric MTS assay by Promega (CellTiter 96 Aqueous Non-Radioactive Cell Proliferation Assay) where dehydrogenase enzymes present in metabolically active cells bioreduce MTS into a colored formazan product soluble in tissue culture medium that is directly proportional to the number of viable cells in culture.28 Exactly 24 hours after cells were treated with potato/plant component extracts, 20 μL of the MTS/PMS solution prepared according to manufacturers protocol28 was added to each individual well and incubated for 1 hour at 37 °C in a humidified, 5% CO2 incubator chamber. The absorbance was read at 490 nm using a Spectromax 640 microplate spectrophotometer.
Quantification and statistical methods based on % growth inhibition.
Absorbance of the treated cells was compared to the absorbance of the untreated control cells from each individual plate and % growth inhibition was calculated using the following calculation
. Standard deviations and coefficients of variations were calculated among triplicate replications. Commonly, coefficients of variations were below 5%. Statistical analyses conducted on results expressed in terms of % growth inhibition for all potato extract treatments was based on three to five biological replicates from two to three harvest years dependent upon clone unless otherwise specified.
Ratio transformations of
were used in the statistical analysis to stabilize normal distributions and equal variance. Depending upon the clone, analyses of variance (ANOVA) was used to evaluate the main effects of year, cooking, sector-flesh color (purple vs. white or red vs. white), developmental stage, extract concentration, preparation process and plant component followed by the Tukey-Kramer test to assess separation of means using SAS® software version 9.2 software (SAS Institute, Cary, NC).42
Apoptosis
The Apoalert® Caspase-3 Colorimetric Assay Kit was utilized to detect caspase-3 protease activity.36 Caspase-3 catalyzes the hydrolysis of Ac-DEVD-p-nitroanilide (pNA) releasing the pNA chromophore (monitored at 405 nm) in mammalian cells that have been induced by apoptosis.36 Three biological replicate extracts at 7.2 mg mL−1 from three inhibitory clones, plus colchicine a known inducer of apoptosis of HT-29 colon cancer cells at 0.005 M concentration, were evaluated for caspase-3 protease activities to establish if an apoptosis signaling pathway was the source of cancer cell growth inhibition (Table S5,†Fig. 4).
All methods were conducted using sterile materials and under sterile conditions. HT-29 colon cells were seeded into a 6 well plate at a rate of 2.5 × 106 cells in 2 mL McCoys 5A, 1× medium containing 10% fetal bovine serum per well and incubated at 37 °C in a humidified, 5% CO2 incubator chamber for 24 hours to allow cells to adhere to the plates. Trypan blue was used in a 1
:
1 ratio to distinguish viable cells from nonviable cells and a hemacytometer and inverted microscope were used to facilitate the cell count during this initial seeding step.
Three biological replicates (separate plants) of immaturely harvested stage II raw CO97216-1P/P, CO97226-2R/R and CO0458-3RW/RW red and white sector 60 mg mL−1 whole tuber potato extracts were reconstituted in 1 mL of aqueous McCoys 5A, 1× medium containing 10% fetal bovine serum, filter sterilized, and 2 mL of 14.4 mg mL−1 tuber extract diluted in McCoys 5A, 1× medium containing 10% fetal bovine was added in triplicate replication to individual wells of a 6 well microplate to achieve a final treatment extract concentration of 7.2 mg mL−1 cell culture medium. All treated microplates were incubated for 24 hours at 37 °C in a humidified, 5% CO2 incubator chamber to allow apoptotic induction to occur. Two controls were utilized during this assay. A negative control reaction was executed on three wells containing seeded HT-29 colon cancer cells left untreated and/or uninduced. A positive control was executed on seeded HT-29 cells where 2 mL of 0.01 M colchicine solubilized in McCoys 5A, 1× medium containing 10% fetal bovine was added in triplicate replication to individual wells of a 6 well microplate to achieve a final treatment concentration of 0.005 M colchicine, a known apoptosis inducer of HT-29 colon cancer cells in culture. All medium was removed exactly 24 hours after cells were treated with potato extracts and controls and 0.5 mL of Hyclone 0.25% Trypsin EDTA, 1× was added to each individual well and incubated for 10 minutes in a humidified, 5% CO2 incubator chamber to allow enzymes to cleave adherent HT-29 cells from each well. A hemacytometer and inverted microscope were utilized to count 2 × 106 cells from each well that were transferred to individual sterile 15 mL conical tubes and centrifuged in a microcentrifuge at 400g for 5 minutes. The assay was conducted according to the manufacturer's protocol.36
Caspase-3 activity was calculated based on a pNA standard curve expressed as nmole pNA. Two-way analysis of variance (ANOVA) was used to evaluate the main effects of treatment and incubation time followed by the Tukey-Kramer test to assess separation of means using SAS® version 9.2 software (SAS Institute, Cary, NC).42 Statistical analyses conducted on results expressed in terms of nmole pNA for all treatments was based on three biological replicates of each potato extract treatment and triplicate replication of positive and negative controls where the Apoalert Caspase-3 Colorimetric assay was conducted only once.
Results
Seven variables impacted and/or optimized inhibitory responses including: germplasm source (cultivars and selections with pigmented versus white tissue from whole tubers and from pigmented sectors); stage of tuber development (immature tubers versus intermediate and mature tubers); extract concentration (mg mL−1 cell culture medium); extraction from uncooked or cooked tuber tissues; plant component (whole tuber, peel only, flesh only, flowers); heated aqueous extracts from raw immature tubers compared to aqueous extracts from immature heated tubers; and extract preparation process (freeze dried vs. cryogenic grinding).
Cultivars and clonal selections.
Only three of the 13 diverse germplasm sources tested, CO97226-2R/R, CO97216-1P/P, and the red sectors of CO04058-3RW/RW (Fig. 2, 3), had high inhibitory properties, in this case defined as >45% growth inhibition of HT-29 cell cultures.
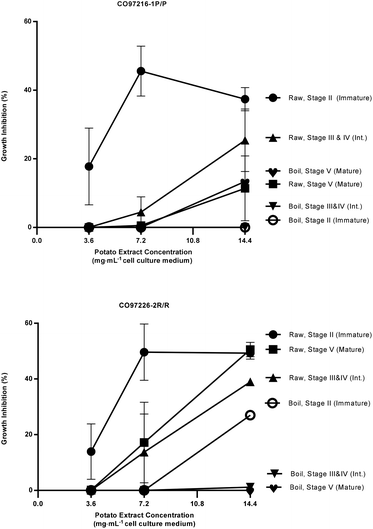 |
| Fig. 2 Percent growth inhibition of HT-29 colon cancer cell cultures by extracts of raw and boiled tubers from CO97216-1P/P and CO97226-2R/R, collected at immature, intermediate and mature developmental stages. Data are means from one, two or three years, 2008 to 2010, error bars are ±SEM. See Table S2 and S3† for exact percent growth inhibitory values and significant differences based on Tukey's HSD, P = 0.05. | |
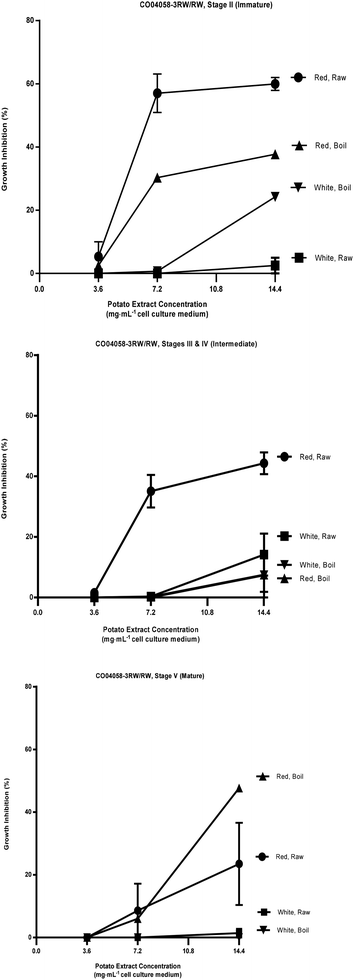 |
| Fig. 3 Percent growth inhibition of HT-29 cell cultures by extracts of raw and boiled tubers from CO04058-3RW/RW, collected at immature, intermediate and mature developmental stages from red and white sectors. Data are means from one, two or three years, 2008 to 2010, error bars are ±SEM. See Table S1 and S4† for exact percent growth inhibitory values and significant differences based on Tukey's HSD, P = 0.05. | |
The most effective inhibition was detected from stage II immature raw extracts at 7.2 and 14.4 mg mL−1 from CO97226-2R/R (50 and 49% over three years, Fig. 2), from CO97216-1P/P (46 and 37% over three years, Fig. 2) and from red-fleshed sectors CO04058-3RW/RW (57 and 60% over two years, Fig. 3). Inhibitory activity (46%) was also detected in raw extracts from stage V mature CO970226-2R/R tubers at a potato extract concentration of 7.2 mg mL−1 cell culture medium in 2010, and in all three production years (51%) at 14.4 mg mL−1 cell culture medium (Fig. 2). Inhibition of 48% was also detected in extracts from raw red sectors of CO04058-3RW/RW at intermediate (stage IV) maturity, and from boiled samples of red tissues of CO04058-3RW/RW grown in 2010 at 14.4 mg mL−1 cell culture medium from stage V mature tubers (Fig. 3).
In contrast, little or no inhibition was detected from extracts taken from white tissue sectors of CO04058-3RW/RW (Fig. 3). Accordingly, a significant increase in inhibition was observed in red tissue sectors when compared to white tissue sectors of boiled stage V mature CO04058-3RW/RW tubers at a potato extract concentration of 14.4 mg mL−1 cell culture medium and of raw stage II immature tubers at both potato extract concentrations of 7.2 and 14.4 mg mL−1 cell culture medium (Fig. 3).
No significant inhibition was detected at any concentration or developmental stage from extracts of the other russet skinned or white fleshed selections or cultivars. However, not all pigmented selections and cultivars inhibited appreciably. For example, extracts from red-fleshed Mountain Rose, purple-fleshed Purple Majesty and red sectors of CO04061-1R/RW did not inhibit to any extent (data not shown). Thus pigmented characteristics alone did not account for the inhibitory properties.
Developmental stage.
With minor exceptions, the most effective inhibition was detected from immature tubers. Inhibition lessened with increasing maturity and except for the three strongly inhibitory clones, generally declined to trivial levels at normal stage V harvest maturity (Fig. 2, 3).
Extract concentration.
While potato extract concentrations of 3.6, 7.2 and 14.4 mg mL−1 cell culture medium were most effective, and some interactions were evident, 7.2 mg mL−1 cell culture medium appeared to be the lowest potato extract concentration that provided high levels of inhibition (Fig. 2, 3). Accordingly, it seems most prudent to emphasize comparative responses at a potato extract concentration of 7.2 mg mL−1 cell culture medium.
Extraction from uncooked and cooked tubers.
Samples prepared from uncooked tubers were overall more effective inhibitors than boiled samples. Boiling tubers resulted in a significant decrease in inhibitory activity in CO97216-1P/P and CO97226-2R/R immature tubers obtained during stage II at a potato extract concentration of 7.2 mg mL−1 cell culture medium (Fig. 2). Boiling also decreased inhibition by CO97226-2RR mature tubers during harvest stage V at both potato extract concentrations of 7.2 and 14.4 mg mL−1. A few notable exceptions were detected where boiled tubers retained some inhibitory activity after heating. For example, boiled stage II tubers of CO97226-2R/R harvested in 2010 inhibited 27% of HT-29 cells in culture at a potato extract concentration of 14.4 mg mL−1 cell culture medium. Boiled red sectors of CO04058-3RW/RW harvested during immature stage II in 2010 inhibited 30 and 37%, from both potato extract concentrations of 7.2 and 14.4 mg mL−1 cell culture medium, while mature boiled red sectors during stage V harvest inhibited 48% at a potato extract concentration of 14.4 mg mL−1 cell culture medium (Fig. 3). These exceptions are in contrast to data from the other clones tested, that did not inhibit HT-29 cell cultures after cooking (data not shown).
Plant components.
Flowers, potato peels, potato flesh and whole tuber samples obtained during an immature developmental stage in 2011 all inhibited HT-29 colon cancer cell cultures in most cultivars and selections. Inhibitory activity from flower and peel samples was high compared to flesh and whole tuber samples tested in 2011 (Fig. 4). Aqueous extracts from russet peels obtained from immature Russet Nugget tubers inhibited HT-29 cell cultures by 82, 70 and 50% at peel extract concentrations of 3.6, 7.2 and 14.4 mg mL−1 cell culture medium, whereas immature flesh alone and immature whole tubers did not inhibit, 0% (Fig. 4). Aqueous extracts from peels obtained from immature Mountain Rose tubers also inhibited cell cultures by 53, 72, and 62% at a peel extract concentrations of 1.8, 3.6 and 7.2 mg mL−1 cell culture medium while immature whole tubers did not inhibit, 0% (Fig. 4). Aqueous extracts from peels of immature Purple Majesty tubers inhibited 56 and 63% at peel extract concentrations of 1.8 and 3.6 mg mL−1 cell culture medium respectively, while immature flesh alone and immature whole tubers had weak to nil inhibition (Fig. 4).
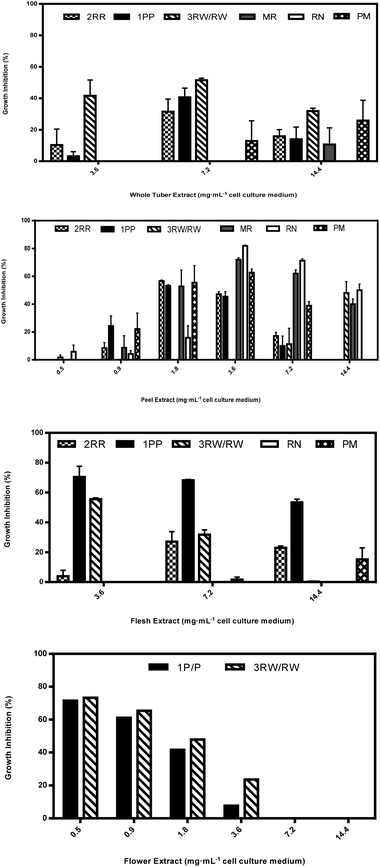 |
| Fig. 4 Percent growth inhibition of HT-29 colon cancer cells after 24 hours by plant component extracts (mg mL−1 cell culture medium) of peel, whole tuber, flesh, and flower obtained from immature developmental stage II tubers during 2011. Data are means ± standard errors of three biological replicates for one harvest year (2011). Legend: 2RR, CO97226-2R/R; 1PP, CO97216-1P/P; 3RW/RW, CO04058-3RW/RW; MR, Mountain Rose; RN, Russet Nugget; PM, Purple Majesty. | |
Aqueous extracts from peels from immature CO97226-2R/R tubers inhibited HT-29 cell cultures 57 and 47% at peel extract concentrations as low as 1.8 and 3.6 mg mL−1, but inhibitory activity in both immature flesh and whole tubers was detected only at 7.2 mg mL−1 cell culture medium (Fig. 4A–C).
Aqueous extracts from flesh obtained from immature CO97216-1P/P tubers inhibited HT-29 cell cultures 70, 68 and 54% at flesh extract concentrations of 3.6, 7.2 and 14.4 mg mL−1 cell culture medium (Fig. 4). Immature flesh extracts were also more effective inhibitors than flower extracts at 3.6, 7.2 and 14.4 mg mL−1 cell culture medium, immature peel extracts at 7.2 and 14.4 mg mL−1 cell culture medium and immature whole tuber extracts at 3.6 and 14.4 mg mL−1 cell culture medium (Fig. 4). On the other hand, immature peel extracts had weak to nil inhibitory activity at extract concentrations of 7.2 and 14.4 mg mL−1 cell culture medium, but displayed inhibitory activity of 53 and 46% at lower extract concentrations of 1.8 and 3.6 mg mL−1 cell culture medium (Fig. 4). Flower extracts inhibited HT-29 cell cultures as high as 72 and 61% at low extract concentrations of 0.5 and 0.9 mg mL−1 cell culture medium (Fig. 4), and flower extracts were also more effective inhibitors than immature peel extracts at 0.5 mg mL−1 cell culture medium. By comparison, immature whole tubers inhibited 41% at 7.2 mg mL−1 cell culture medium (Fig. 4).
Aqueous extracts from immature red flesh of CO04058-3RW/RW were superior to peels at an extract concentration of 3.6 mg mL−1 cell culture medium, inhibiting HT-29 cell cultures 56% (Fig. 4). Immature peels were superior to immature red flesh extracts inhibiting cell cultures 48% but only when extract concentrations were as high as 14.4 mg mL−1 cell culture medium (Fig. 4). Red sectors from immature whole tubers inhibited cell cultures 52% and were more effective than immature peel extracts at an extract concentration of 7.2 mg mL−1 cell culture medium (Fig. 4). Flower extracts inhibited HT-29 cell cultures 73, 65 and 48% at extract concentrations as low as 0.5, 0.9 and 1.8 mg mL−1 cell culture medium and were also more effective inhibitors of cell cultures than immature peel extracts at 0.5 and 0.9 mg mL−1 cell culture medium (Fig. 4).
Effects of freeze dried tuber extracts on caspase-3 protease.
Colchicine, a known inducer of apoptosis, produced the highest level of caspase-3 activities with an average of 22.5 nmole pNA (Fig. 5). Aqueous extracts from red tissue sectors of CO04058-3RW, that strongly inhibited HT-29 cancer cell cultures on average 57% over two years, produced the second highest level of caspase-3 activities with an average of 12.9 nmole pNA (Fig. 5). Conversely, aqueous extracts from white tissue sectors from this sectorial expressing selection, that was a weak inhibitor of HT-29 cancer cell cultures, produced statistically the same amount of caspase-3 activity as the untreated control averaging 6.3 nmole pNA (Fig. 5). CO97216-1P/P and CO97226-2R/R aqueous extracts, that were both strong inhibitors of HT-29 cancer cell cultures averaging 46 and 50% over three years, were statistically classified the same as the weak inhibiting aqueous extracts from white sectors of CO04058-3RW/RW producing 9.5 and 11.4 nmoles pNA (Fig. 5).
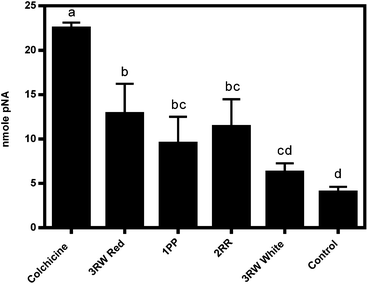 |
| Fig. 5 Caspase-3 protease activity (nmole pNA chromophore) measured after 24 hours of HT-29 colon cancer cell cultures treated by aqueous extracts (7.2 mg mL−1 cell culture medium) of three strong inhibitory clones (CO97226-2R/R, CO97216-1P/P, CO04058-3RW/RW red sector) and one weak inhibitory clone (CO04058-3RW/RW white sector) obtained from immature developmental stage II in addition to a negative control (untreated cells) and positive control (0.005 M colchicine). Data are means ± standard errors of three biological replicates obtained for one harvest year (2010) where the experiment was conducted only once. Letters denote statistically significant differences in caspase-3 protease activities. Legend: 2RR, CO97226-2R/R; 1PP, CO97216-1P/P; 3RW Red, CO04058-3RW/RW red sector; 3RW White, CO04058-3RW/RW white sector. | |
Thermal stability of inhibitory properties in heated tubers and heated extracts both stored for six months.
Immature tubers stored at 4 °C for six months retained their valuable inhibitory properties under normal potato storage conditions (Table S5†). Differences in temperature sensitivity were detected at 7.2 mg mL−1 cell culture medium (Fig. 6A–B). Higher inhibitory activity was found in extracts from heated tubers than in heated extracts at all temperatures in CO97216-1P/P and at 45, 55–60 and 72 °C in CO97226-2R/R primarily at 7.2 mg mL−1 (Fig. 6A–B). Heated extracts of CO97216-1P/P and CO97226-2R/R had weak inhibitory (<45%) activity upon exposure to six different temperatures (Fig. 6A–B).
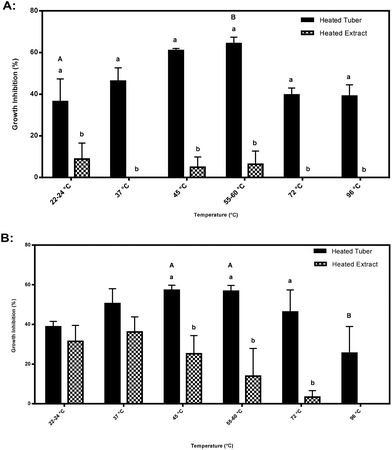 |
| Fig. 6 (A–B) Percent growth inhibition of HT-29 colon cancer cells after 24 hours, comparing heated extracts to heated whole tubers (7.2 mg mL−1 cell culture medium) from: (A) immature CO97216-1P/P tubers, and (B) immature CO97226-2R/R tubers. Data are means of all replicates (%). Three biological replicates were tested. Percent growth inhibition data represented in this figure were ratio transformed to conduct Tukey's HSD analysis. Lower case letters denote statistically significant differences between heated tubers and heated extract treatments at an extract concentration of 7.2 mg mL−1 cell culture medium. Upper case letters denote statistically significant differences between temperatures between heated tubers at a specific extract concentration based on Tukey-Kramer's HSD. | |
Significant differences were also found when comparing inhibitory activity amongst heated tubers subjected to different temperatures. Inhibitory activity at an extract concentration of 7.2 mg mL−1 cell culture medium by CO97216-1P/P heated tubers increased from 37% at 22–24 °C to 64% at 55–60 °C (Fig. 6A). Inhibitory activity at an extract concentration of 7.2 mg mL−1 cell culture medium by CO97226-2R/R heated tubers decreased by half from 57% at 45 and 55–60 °C to 26% inhibitory activity at 96 °C (Fig. 6B).
Cryogenic extraction vs. freeze drying in tubers stored for six months.
Immature tubers stored at 4 °C for six months retained their inhibitory properties under normal potato storage conditions (Table S5†). All aqueous extracts from the two tuber selections inhibited HT-29 colon cancer cell cultures, but the preparation process affected only CO97216-1P/P (P = 0.002) (Fig. 7). Aqueous extracts from immature CO97216-1P/P tubers that were prepared via cryogenic grinding prior to extraction in organic solvent inhibited HT-29 cell cultures 39 and 55% more at potato extract concentrations of 7.2 and 14.4 mg mL−1 when compared to aqueous extracts from immature tubers that were prepared via freeze drying prior to extraction in organic solvent (Fig. 7). Aqueous extracts from CO97226-2R/R were not significantly different at P = 0.05 with regards to preparation methods prior to extraction in organic solvent.
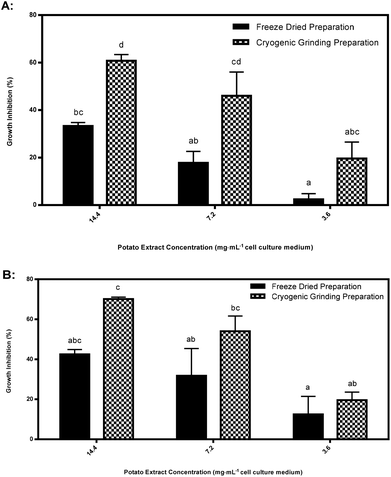 |
| Fig. 7 (A–B) Percent growth inhibition of HT-29 colon cancer cells after 24 hours by extracts reconstituted in cell culture medium that were prepared either cryogenically or by freeze drying from: (A) raw immature CO97216-1P/P tuber tissues, and (B) raw immature CO97226-2R/R tuber tissues. Data are means of experiment repeated twice ± standard errors of three biological replicates for one year of harvest (2010). Letters denote statistically significant differences between preparation methods within an extract concentration. | |
Discussion
Plant explorations and taxonomic systematic research many decades ago established existence of extensive biodiversity among species and land races of potato in the Andes.38 While European plant explorers were instrumental in introducing potatoes as a valuable food staple especially suited to cool climates, clones with red and purple flesh were ignored or selected against in favor of white-fleshed tubers. Recent research6,34,35 has shown that the pigmented genotypes possess several fold greater levels of antioxidant content and radical scavenging capacity, primarily attributed to their much higher polyphenolic anthocyanins and related simple phenolic acids. Thus a logical next step was to explore if these unique genotypes may or may not possess inhibitory properties when exposed to HT-29 colon cancer cells in culture. Results from this research revealed that while maximum inhibition was detected in clones with red and purple-fleshed tubers, not all pigmented clones have inhibitory properties. While a few white-fleshed tubers inhibited HT-29 cell cultures, percent growth inhibition was much less pronounced than that from pigmented tissues.
Selections that express both red and white tissues in the same tuber, such as CO04058-3RW/RW, and display inhibitory properties provide an ideal model to study gene expression differences associated with pigment content. These genotypes eliminate genotype by environment, genotype by developmental stage, genotype by nutrient content and soil nutrient plant-to-plant variation. Expression thus depends on activity of the transcriptome in each type of tissue and greatly sharpens capacity to interpret gene action.35 Inhibition by red tissues exceeded that of white tissues, reinforcing the possibility that some metabolite(s) closely associated with the flavonoid biosynthetic pathway may be involved.8,15,19,21,29–32 Only a few clones were tested in this study, thus it is probable that other untested cultivars and selections may also possess inhibitory properties that could provide valuable dietary intervention to guard against HT-29 colon cancer cells.
Developmental stage
A major factor contributing to inhibition, reported here for the first time, is the unique property of enhanced inhibition associated with developmental stage. Possible explanations include: upregulation of key inhibitory metabolites found only at the outset of tuber initiation and early development or possible degradation of the bioactive agent with tuber maturity for unknown reasons. Ramifications of this finding are important and interesting for both consumers and growers of potato should the phenomenon that exists between cancer inhibition and immature harvested potatoes be discerned. An optimistic view may be a reduced incidence of colon cancer as a dietary intervention simply by consumption of immature cancer inhibiting tuber clones and/or development of effective intervention creating niche markets for growers, and promising research leads on human health.
While it is clear that the earliest developmental stages provided tubers with the highest inhibitory bioactivity, modern potato production practices in the San Luis Valley attempt to control harvest date by employing top kill practices to induce tuber maturity. Thus the true mature stage may vary from year to year as a function of when growers apply top-kill treatments to ensure harvest in a timely manner. Accordingly, accurate identification of immature tubers becomes important to pin point an active inhibitory phase.
Extract concentration
Identification of the lowest threshold (7.2 mg mL−1) extract concentration that provides consistent inhibition is important to provide a starting point for screening novel clones. In addition, this may serve as a guide to provide a dietary guideline of how much potato product one may consume should future research demonstrate that inhibitory compounds in potato can be successfully delivered to the colon undamaged by the digestive process.
Extraction from uncooked and cooked tubers
Cooking was a major factor affecting inhibition. Overall uncooked tubers retained inhibitory properties better than cooked tubers. In spite of this, immature red sectors of CO04058-3RW/RW and CO97226-2R/R retained half of their inhibitory activity after cooking when compared to their raw counterparts during stage II. This finding is important for several reasons. Potatoes are typically consumed as a cooked product. In order for a consumer to reap the potential protective benefits against colon cancer, inhibitory compounds must be delivered to the colon undamaged. Thus, finding a genotype that possesses inhibitory properties against HT-29 colon cancer cells and can withstand cooking is essential for a consumer to reap these benefits as well as for a grower to market these benefits.
This also suggests more than one bioactive compound may be responsible for inhibition of HT-29 colon cancer cells in culture. Glycoalkaloids, a class of heat stable33 secondary metabolites composed of a trisaccharide chain attached to an aglycone13 exist in potato and have been confirmed in several studies to successfully inhibit cancer cells in culture.1,14,22,41 The partial inhibitory activity retained in these two selections after cooking may be a result of heat stable glycoalkaloids, while the inhibitory activity that has been lost to cooking suggests that glycoalkaloids may not be the sole inhibitors of HT-29 colon cancer cell cultures. In this study, purple tissues correlated well with high solanine content (r = 0.810, P = 0.03), but when glycolakaloid content of several pigmented and white fleshed selections and cultivars, investigated previously,35 was correlated with HT-29 cell culture inhibition using Spearman non-parametric analysis, no relationship of pigmented sources to glycoalkaloid content was detected for solanine (r = 0.1317, P = 0.75) nor for chaconine (r = 0.1557, P = 0.70). Thus, identity of the most likely inhibitory agent requires further investigation.
Plant components
Inhibitory activity varied considerably among potato plant components, as well as between immature and mature tubers. Aqueous flower extracts appear to be an exceptional source of HT-29 cancer cell culture inhibitory metabolites. Flower extracts demonstrated some of the strongest inhibitory activity compared to other plant components where increasing inhibition was found with decreasing extract concentrations as low as 0.5 mg mL−1 cell culture medium (Fig. 4).
Aqueous peel extracts were also a potent source of HT-29 cancer cell culture inhibitory metabolites (Fig. 4). Cultivars such as Mountain Rose, Purple Majesty and Russet Nugget, that typically display nil or weak inhibitory responses to HT-29 cancer cell cultures from extracts of whole tubers and/or flesh revealed strong inhibitory activity in extracts from peels (Fig. 4). Clones such as CO97216-1P/P and CO97226-2R/R that typically display strong inhibitory activity against HT-29 cancer cell cultures at higher extract concentrations of 7.2 mg mL−1 cell culture medium from whole tuber extracts demonstrated strong inhibitory activity at peel extract concentrations as low as 1.8 mg mL−1 cell culture medium (Fig. 4).
This response observed in both peel and flower extracts may be attributable, at least partially to glycoalkaloids. Endogenous content of the prominent glycoalkaloids in potato (solanine and chaconine) are largely dependent on genotype, maturity, stress and other environmental factors.7,13 They are mostly positioned around the eyes located on the outer layer of the tuber and within the first 1 mm from the exterior surface of the tuber decreasing toward the interior of the tuber as well as metabolically active areas of the plant such as flowers, young leaves and small immature tubers.7,13
Generally, peel extracts from CO97216-1P/P had higher inhibitory properties than whole tuber extracts at the lower extract concentrations, and flower extracts were also most inhibitory at the lowest extract concentrations (Fig. 4). Flesh extracts from CO04058-3RW/RW inhibited as well as peel extracts, but only at 3.6 mg mL−1 cell culture medium (Fig. 4). This may suggest that another class of inhibitory metabolites is present at bioactive concentration in the pigmented fleshy tissues in these two selections. However, differences in content of inhibitors may also be due to their genetic differences. Although biological replicates were utilized, this portion of this study was based on only one harvest year of plant components, thus requiring more testing to account for year to year variability that may occur amongst biological replicate clones.
Caspase-3 protease activities
Apoptosis, programmed cell death, is initiated via a sequential cascade of caspase proteolytic activity by one or more caspase proteases (caspase-2, -3, -6, -7, -8, -9, -10 and -12) and caspase detection may be used as an indicator of apoptotic cell death.26
The mechanism of inhibition may be attributed at least in some part to apoptosis in red sectors of CO04058-3RW/RW (Fig. 5). While, other inhibitory selections tested did not reveal high caspase-3 activities, some protease activity was detected, thus apoptosis may be the source of their inhibition as well (Fig. 5). A possible explanation for reduction in apoptotic activity in strong inhibiting clones as well as a possible shortcoming of this study is that the assay utilized, only detects the presence of caspase-3 activities. Although caspase-3 is a crucial protease implicated in the execution phase of apoptosis, several other caspases exist throughout the apoptoic cascade that also play key roles in initiation and execution of apoptosis that may be more readily induced by strongly inhibiting tuber clones. Although the Apoalert® Caspase-3 Colorimetric Assay Kit utilized in this study detected an increase in caspase-3 protease activities, further analyses measuring other caspase proteases in addition to alternate apoptotic induction markers needs to be assayed to confirm induction of apoptosis as source of inhibitory activity.
Thermal stability of inhibitory properties in heated tubers and heated extracts
Purple pigmented CO97216-1P/P heated tubers were thermally stable and did not lose any inhibitory properties upon heating, but rather increased in inhibitory activity at 60 °C at 7.2 mg mL−1 cell culture concentration, compared to cooler and warmer temperatures (Fig. 6A). Purple pigmented CO97216-1P/P heated tubers were thermally stable up to 90 °C (Fig. 6A). The stability of the inhibitory nature observed at higher temperatures once again suggests partial activity due to glycoalkaloids.
Red pigmented heated tubers, CO97226-2R/R behaved differently where inhibitory activity at boiling decreased by half, suggesting a heat labile compound, perhaps an endogenous enzyme (Fig. 6B).
Higher inhibitory activity was found in heated tubers when compared to heated extracts at all temperatures in CO97216-1P/P and at 45, 55–60 and 72 °C in CO9722-2R/R tubers (Fig. 6A–B). Accordingly, the tuber matrix itself may serve to protect and conserve inhibitory metabolites from thermal degradation whereas inhibitory metabolites alone found in heated extracts do not have a tuber matrix to protect activity.
Significant differences in temperature sensitivity were detected at an extract concentration of 7.2 mg mL−1 cell culture medium. Thus it seems prudent to conduct future analyses at an extract concentration of 7.2 mg mL−1 cell culture medium.
Cryogenic extraction vs. freeze drying
Cryogenic extraction using rapid freezing with liquid nitrogen derived acetone powders significantly enhanced inhibition in purple pigmented tubers compared to freeze dried tissues (Fig. 7). Explanations for degradation of bioactive agents inhibiting HT-29 cancer cell cultures are difficult to pin-point as the precise inhibitory agents are unknown. Possible explanations are: large ice crystal formation during prefreezing at −40 °C was more damaging of enzymatic structure and function compared to formation of small ice crystals during rapid immersion in liquid nitrogen in cryogenically ground tubers;23 cellular dehydration;23 solute toxicity or a pH change causing denaturation of proteins.5 Oxidation of freeze-dried tubers may have also played a role in the degradation of the bioactive agent inhibiting HT-29 cancer cell cultures.5 Only freeze dried purple pigmented tubers declined in inhibitory response while red pigmented tubers did not decline significantly. Perhaps the active agent(s) in purple pigments are more sensitive to degradation than red pigments if they or related compounds play a role as inhibitors of HT-29 cancer cell cultures.
In this phase of the study, biological replicates from only two clones were compared, thus it is possible that other genotypes may also possess elevated inhibitory responses from cryogenically ground preparations, but this requires considerably more research to identify the observed increase between extract preparation methods.
Storage of immature tubers
Earlier we discovered that inhibitory properties in CO97226-2R/R and CO97216-1P/P selections appeared to maximize at discreet immature stages, but decreased as tubers approached field maturity (Fig. 2). However, immature tubers of CO97226-2R/R and CO97216-1P/P stored at 4 °C for six months retained their inhibitory properties under normal potato storage conditions (Table S5;†Fig. 6A–B, 7A–B).
Conclusion
This study clearly identifies potato genotypes providing tissues that show considerable variation in their capacity to inhibit HT-29 colon cancer cell growth. Further work is needed to investigate whether these elite potato genotypes possess similar inhibitory activity against various other mammalian colon cancer cell lines in culture. Additionally, uncovering the inhibitory mode of action (e.g. cell cycle regulation, apoptoic induction and/or cytotoxicity) against various colon cancer cell lines are necessary. Future work may take advantage of recently evolving metabolomic approaches, coupled with statistical methods to identify the metabolites driving this trait difference. Powerful association genetic approaches may also be possible to understand the genetic architecture of the trait and ultimately to provide the necessary tools to develop cultivars with enhanced health properties.
Acknowledgements
The authors gratefully acknowledge provision of graduate fellowship support for Tatiana Zuber from the USDA-NIFA National Needs Graduate Fellowship Program (grant no. 2007-38420-17766) and the Crops for Health program of the CSU College of Agricultural Sciences, research support from the Colorado Potato Research Committee, as well as production and provision of potato samples from the Colorado Potato Breeding program, San Luis Valley Research Center.
References
- H. Al-Ashaal, Fitoterapia, 2010, 81, 600–606 CrossRef CAS PubMed.
-
American Cancer Society, Global Cancer Facts and Figures, Amer. Cancer Soc., Atlanta, 2nd edn, 2011 Search PubMed.
-
American Cancer Society, Colorectal Cancer Facts and Figures 2014–2016, Amer. Cancer Soc., Atlanta, 2014 Search PubMed.
- D. Aune, R. Lau, D. S. Chan, R. Vieira, D. C. Greenwood, E. Kampman and T. Norat, Gastroenterology, 2011, 141, 106–118 CrossRef PubMed.
-
J. G. Brennan and A. S. Grandison, Food processing handbook, Wiley-VCH, Weinheim, Germany, 2nd edn, 2012 Search PubMed.
- C. R. Brown, Am. J. Potato Res., 2005, 82, 163–172 CrossRef CAS.
-
Solanine and Chaconine, http://www.inchem.org/documents/jecfa/jecmono/v30je19.htm12 (accessed November 2011).
- P. Bontempo, V. Carafa, R. Grassi, A. Basile, G. C. Tenore, C. Formisano, D. Rigano and L. Altucci, Food Chem. Toxicol., 2013, 55, 304–312 CrossRef CAS PubMed.
- H. R. Cheng, Z. Y. Zhang, J. Y. Leng, D. Kiu, M. Hao, X. G. Gao, G. H. Tai and Y. F. Zhou, Int. J. Food Sci. Nutr., 2013, 64, 36–43 CrossRef CAS PubMed.
- R. Ezekiel, N. Singh, S. Sharma and A. Kaur, Food Res. Int., 2013, 50, 487–496 CrossRef CAS PubMed.
- R. Feng, Y. Lu, L. L. Bowman, Y. Qian, V. Castranova and M. Ding, J. Biol. Chem., 2005, 280, 7888–7895 Search PubMed.
-
J. Ferlay, I. Soerjomataram, M. Ervik, R. Dikshit, S. Eser, C. Mathers, M. Rebelo, D. M. Parkin, D. Forman and F. Bray, GLOBOCAN 2012 v1.0, Cancer Incidence and Mortality Worldwide: IARC CancerBase No. 11 [Internet], International Agency for Research on Cancer, Lyon, France, 2013 Search PubMed (accessed on April 2014).
- M. Friedman, J. Agric. Food Chem., 2006, 54, 8655–8681 CrossRef CAS PubMed.
- M. Friedman, K. R. Lee, H. J. Kim, I. S. Lee and N. Kozukue, J. Agric. Food Chem., 2005, 53, 6162–6169 CrossRef CAS PubMed.
-
J. Harborne, The flavonoids advances in research since 1986, Chapman and Hall/CRC, London, 1st edn, 1993 Search PubMed.
- P. Harris, A. Robertson, H. Hollands and L. Ferguson, Mutat. Res., Fundam. Mol. Mech. Mutagen, 1991, 260, 203–213 CAS.
- K. Hayashi, H. Hibasami, T. Murakami, N. Terahara, M. Mori and A. Tsukui, Food Sci. Technol. Res., 2006, 12, 22–26 CrossRef CAS.
-
M. S. Kang and P. Priyadarshan, Breeding major food staples, Blackwell Publishing, Ames, Iowa, 2007 Search PubMed.
- J. Kuhanu, World Rev. Nutr. Diet., 1976, 24, 117–120 Search PubMed.
- R. Liu and G. Xu, Food Chem. Toxicol., 2008, 46, 2672–2679 CrossRef CAS PubMed.
- G. P. Madiwale, L. Reddivari, M. Stone, D. G. Holm and J. Vanamala, J. Agric. Food Chem., 2012, 60, 11088–11096 CrossRef CAS PubMed.
- T. Mandimika, H. Baykus, Y. Vissers, P. Jeurink, J. Poortman, C. Garza, H. Kuiper and A. Peijnenburg, J. Agric. Food Chem., 2007, 55, 10055–10066 CrossRef CAS PubMed.
- P. Mazur, Am. J. Physiol., 1984, 247, C125–C142 CAS.
- National Cancer Institute, PDQ® High-Dose Vitamin C, National Cancer Institute, Bethesda, MD. Date last modified 7.10.2014. Available at: http://www.cancer.gov/cancertopics/pdq/cam/highdosevitaminc/healthprofessional. Accessed 17.10.2014 Search PubMed.
- M. Nzaramba, L. Reddivari, J. Bamber and J. Miller, J. Agric. Food Chem., 2009, 57, 8308–8315 CrossRef CAS PubMed.
- Q. P. Peterson, D. R. Goode, D. C. West, R. C. Botham and P. J. Hergenrother, Nat. Protocols, 2010, 5, 294–302 CrossRef CAS PubMed.
-
H. B. Pollard, M. A. Levine, O. Eidelman and M. Pollard, In Vivo, 2010, 24, 249–255 Search PubMed.
-
Promega Corporation, CellTiter 96 Aqueous Non-Radioactive Cell Proliferation Assay, Promega Corporation, Madison, WI., 2007 Search PubMed.
- L. Reddivari, J. Vanamala, S. Chintharlapalli, S. H. Safe and J. C. Miller, Carcinogenesis, 2007, 28, 2227–2235 CrossRef CAS PubMed.
- C. A. Rice-Evans, N. J. Miller and G. Paganga, Free Radicals Biol. Med., 1996, 20, 933–956 CrossRef CAS.
- K. Robards, P. D. Prenzler, G. Tucker, P. Swatsitang and W. Glover, Food Chem., 1999, 66, 400–436 CrossRef.
- S. Sharma, J. D. Stutzman, G. J. Kelloff and V. E. Steele, Cancer Res., 1994, 54, 5848–5855 CAS.
- J. Skarkova, V. Ostry and J. Ruprich, J. Planar Chromatogr. Mod. TLC, 2008, 21, 113–117 CrossRef CAS.
- C. Stushnoff, D. Holm, M. D. Thompson, W. Jian, H. J. Thompson, N. I. Joyce and P. Wilson, Am. J. Potato Res., 2008, 85, 267–276 CrossRef PubMed.
- C. Stushnoff, L. J. M. Ducreux, R. D. Hancock, P. E. Hedley, D. G. Holm, G. J. McDougall, J. W. McNicol, J. Morris, W. L. Morris, J. A. Sungurtas, S. R. Verrall, T. Zuber and M. A. Taylor, J. Exp. Bot., 2010, 61, 1225–1238 CrossRef CAS PubMed.
- Clontech Laboratories of Takara Bio Company: ApoAlert Caspase-3 Colorimetric Assay Kit, http://www.clontech.com/US/Products/Cell_Biology_and_Epigenetics/Apoptosis_Kits/Caspase_Profiling?sitex=10020:22372:US (accessed August 2011).
- M. D. Thompson, H. J. Thompson, J. N. McGinley, E. S. Neil, D. K. Rush, D. G. Holm and C. Stushnoff, J. Food Compos. Anal., 2009, 22, 571–576 CrossRef CAS PubMed.
-
N. I. Vavilov, The origin, immunity and breeding of cultivated Plants, The Ronald Press Co., New York, 1951 Search PubMed.
-
World Cancer Research Fund/American Institute for Cancer Research, Food, Nutrition, Physical Activity and the Prevention of Cancer: a Global Perspective, AICR, Washington DC, 2007 Search PubMed.
-
World Cancer Research Fund/American Institute for Cancer Research. Policy and Action for Cancer Prevention, Food, Nutrition, Physical Activity: a Global Perspective, AICR, Washington DC, 2009 Search PubMed.
- S. A. Yang, S. H. Paek, N. Kozukue, K. R. Lee and J. A. Kim, Food Chem. Toxicol., 2006, 44, 839–846 CrossRef CAS PubMed.
-
SAS Institute Inc., SAS® 9.2, SAS Institute Inc., Cary, NC, 2008 Search PubMed.
Footnote |
† Electronic supplementary information (ESI) available. See DOI: 10.1039/c4fo00649f |
|
This journal is © The Royal Society of Chemistry 2015 |
Click here to see how this site uses Cookies. View our privacy policy here.