DOI:
10.1039/C4FO00970C
(Paper)
Food Funct., 2015,
6, 296-303
The anti-obesity effect of green tea polysaccharides, polyphenols and caffeine in rats fed with a high-fat diet
Received
25th October 2014
, Accepted 13th November 2014
First published on 14th November 2014
Abstract
Beneficial effects of green tea (Camellia sinensis, Theaceae) extracts against obesity have been reported; however, the anti-obesity ability of the major components of green tea, polysaccharides, polyphenols and caffeine is not clear. Therefore, experiments with total green tea extracts, polyphenols, polysaccharides, caffeine, and a complex of polysaccharide and polyphenol at a dose of 400 or 800 mg kg−1 were conducted on high-fat diet fed rats for 6 weeks to investigate their anti-obesity effects. The results indicated that polyphenols and polysaccharides were responsible for the suppressive effect of green tea extracts on body weight increase and fat accumulation. Moreover, polyphenols, polysaccharides, or caffeine can improve blood lipid and antioxidant levels, and effectively reduce rat serum leptin levels, inhibit the absorption of fatty acids, and markedly reduce the expression levels of the IL-6 and TNF-α gene. Furthermore, it was shown that polysaccharides and polyphenols were synergistic in reduction of serum leptin levels and in anti-inflammatory activity. These results suggest that the polysaccharide combination with polyphenols might be a potential therapy against obesity.
1. Introduction
Obesity is a serious medical health problem in the world, and the prevalence of obesity has increased dramatically over several decades.1–3 It is associated with increased health-care costs, reduced quality of life, and increased risk of various chronic diseases such as type II diabetes, hypertension, coronary artery disease, and various forms of cancer.4,5 Obesity is a complex metabolic disorder which is caused by a positive energy balance, where energy intake exceeds energy expenditure.6,7 Currently, the available therapeutic approaches for treating obesity have a number of side effects.8,9 Therefore, growing attention has been given to natural products that are characterized as anti-obesity agents.10,11
As a beverage, green tea is widely consumed, especially in East Asian countries.12 It contains abundant bioactive substances, including polysaccharides, caffeine and catechins. The catechin found in green tea mainly comprises epigallocatechingallate (EGCG), epigallocatechin (EGC), epicatechingallate (ECG), and epicatechin (EC),13 which has been suggested to be responsible for health effects.14 Due to the ever-growing obesity pandemic, the anti-obesity effects of green tea are being increasingly investigated.15 In 1999, Dulloo et al.16 found that administration of a green tea extract significantly increased energy expenditure and fat oxidation in a group of young males. Since then, several clinical trials have reported the effects of tea preparations on increasing energy expenditure, fat oxidation, weight loss, fat mass, and weight maintenance after weight loss.17–19 Nevertheless, whether these effects are due to catechins or caffeine has yet to be resolved. Several studies in rodent models have shown that the green tea extract decreases weight gain and body fat gain.20,21 In 2005, it was reported that treatment with TEAVIGO, a green tea extract containing 94% EGCG and 0.1% caffeine, significantly reduced body weight (BW) and body fat in different strains of mice fed with a high-fat diet.22,23 Choo reported that consumption with the water extract of green tea for 2 weeks decreased the body fat accumulation in high fat diet rats.24
There are many reports about the anti-obesity effects of green tea;19,25–28 however, little is known about the underlying mechanisms of action, its role in the regulation of body weight and lipolytic action and its relationship with inflammatory status. Recently, scientists showed that green tea polyphenols had anti-obesity effects by up-regulating the adiponectin level in rats. Some studies have suggested that the involved mechanisms are the inhibition of ERK activation, alleviation of peroxisome proliferator-activated receptor γ (PPAR-γ) phosphorylation, and increases in the PPAR-γ expression.29 In addition, Lu et al.30 found that the gene expression of interleukin 6 receptor alpha (IL-6ra) was significantly increased in the rats fed with a high-fat diet compared to the normal control.
In fact, the anti-obesity functions of different teas or of the same tea from different areas are probably different, and the different compositions of the same tea may also have different effects. Analytically, it is difficult to determine a particular composition in green tea that is singly responsible for the anti-obesity effect, and the studies on the relationship between bioactive substances and anti-obesity ability have not been conducted yet. Therefore, the aim of this study was to isolate polyphenols, polysaccharides and caffeine from green tea and then investigate their influence on the development of obesity so that it can be comprehensively concluded how green tea extracts reduce the body fat of rats.
2. Materials and methods
2.1 Chemicals and animal
Coarse old green tea is produced in Fujian, China. Experimental animals were Sprague-Dawley (SD) male rats, 100–130 g, which were purchased from Peking University Health Science Laboratory Animal Science, license number SCXK (Beijing) 2009-0017.
2.2 Separation and extraction from green tea
The total water extract of green tea was obtained under conditions of water heated 3 times to 85 °C at a solid–liquid ratio of 1
:
15 . Tea polysaccharide was extracted from tea water by a method of water extraction–alcohol precipitation. Then ethyl acetate was used to extract tea polyphenols and trichloromethane was used to extract caffeine from tea infusion. The purity of tea polyphenols and caffeine was analyzed by high performance liquid chromatography (HPLC), and tea polysaccharide by the phenol–sulfate method.31 The purity of the polyphenols which mainly contain EGC (45.43%), EGCG (28.64%), EC (11.27%), ECG (14.66%) was 71%, caffeine was 73.5% and tea polysaccharide (neutral sugar) was 27.5%.
2.3 Experimental design and dietary treatment
All the experimental procedures were approved by the Committee on the Ethics of Animal Experiments of Tianjin University of Science & Technology and according to the National Institutes of Health Guide for Care and Use of Laboratory Animals.
One hundred and ten male SD rats were purchased from the Peking University Health Science Laboratory Animal Science and kept in a specific pathogen-free facility. The rats were housed at 23 ± 3 °C, provided free access to water and food, and subjected to a 12 h/12 h light/dark cycle. The animals were acclimatized for 7 days and then randomly divided into a normal control group, positive control group (orlistat), and model control group. The total water extract, tea polyphenols, tea polysaccharides, the complex of tea polysaccharides and polyphenols and the caffeine test group and each treatment group in addition to caffeine were divided into two doses, high and low. These groups were referred to as NC, MC, OC, TWH, TWL, TPPH, TPPL, TPSH, TPSL, TPSM and CF groups, respectively. Since the start of the experiment, the positive control group, model control group, and treatment group received a high-fat diet (79% of the basal feed, 10% lard, 10% egg yolk powder, 0.5% cholesterol, 0.5% cholate). The normal control group was given the basal diet. The specific design is shown in Table 1. Weighing and measurement of body length were conducted daily, and intragastric administration was regular. Each animal's food intake and leftover food were recorded daily. Rat droppings were collected once a week and freeze-dried for later use.
Table 1 Body weight and other characters of rats in each groupa
Groups |
Initial BW (g) |
Final BW (g) |
Lee's index |
Food utilization (%) |
All values are means ± SD (n = 12). Compared with the NC group and MC group #P < 0.05. Compared with the MC group and drug group *P < 0.05. NC, standard chow plus saline; MC, high-fat diet with 79% of the standard chow, 10% lard, 10% egg yolk powder, 0.5% cholesterol, 0.5% cholate content plus saline; OC, high-fat diet feed plus orlistat capsule 40 mg per kg BW; TWH, high-fat diet feed plus total water extract 800 mg per kg BW; TWL, high-fat diet feed plus total water extract 400 mg per kg BW; TPPH, high-fat diet feed plus tea polyphenols 800 mg per kg BW; TPPL, high-fat diet feed plus tea polyphenols 400 mg per kg BW; TPSH, high-fat diet feed plus tea polysaccharide 800 mg per kg BW; TPSL, high-fat diet feed plus tea polysaccharide 400 mg per kg BW; TPSM, high-fat diet feed plus the complex of tea polysaccharide and polyphenols (1 : 1) 800 mg per kg BW. CF, high-fat diet feed plus caffeine 400 mg per kg BW.
|
NC |
176.54 ± 7.54 |
349.06 ± 35.70 |
316.57 ± 10.30 |
11.13 ± 0.55 |
MC |
182.98 ± 13.93 |
384.96 ± 32.59# |
327.63 ± 8.36# |
15.06 ± 1.07# |
OC |
175.80 ± 9.02 |
363.23 ± 46.27 |
315.06 ± 5.67* |
12.12 ± 0.49* |
TWH |
179.91 ± 10.65 |
337.05 ± 37.38* |
315.11 ± 9.48* |
12.59 ± 0.72* |
TWL |
175.40 ± 11.28 |
350.68 ± 39.05 |
314.36 ± 10.58* |
11.36 ± 0.80* |
TPPH |
175.39 ± 5.07 |
341.44 ± 19.55* |
312.68 ± 12.53* |
12.93 ± 0.61* |
TPPL |
181.18 ± 12.53 |
319.87 ± 36.19* |
318.89 ± 15.69* |
12.98 ± 0.53* |
TPSH |
182.09 ± 16.42 |
338.76 ± 33.31* |
312.83 ± 11.59* |
12.91 ± 0.81* |
TPSL |
174.39 ± 5.26 |
368.56 ± 47.46 |
316.99 ± 11.03* |
15.04 ± 0.77 |
TPSM |
177.11 ± 12.90 |
316.07 ± 32.60* |
309.58 ± 10.66* |
13.04 ± 0.89* |
CF |
172.02 ± 14.42 |
311.76 ± 46.26* |
307.31 ± 14.04* |
12.62 ± 1.07* |
2.4 Biochemical analyses of serum parameters
After six weeks of the experiment, all rats fasted for 12 hours and then were dissected, meanwhile fat accumulation in the liver and internals of rats was examined with the naked eye. Blood was taken from the rat femoral artery and serum was separated. An automatic biochemical apparatus was used to estimate the levels of total cholesterol (TC), triglyceride (TG), high density lipoprotein cholesterol (HDL-C), low density lipoprotein cholesterol (LDL-C), total superoxide dismutase (T-SOD), malondialdehyde (MDA), in serum. In addition, the level of leptin was determined by ELISA.
2.5 Adipose and liver histopathology
Suitable epididymal adipose tissue and liver of rats were selected and fixed in 10% formalin for 16 hours. Then all tissues were dehydrated in graded ethanol (70% ethanol 10 min; 80% ethanol 10 min; 95% ethanol 10 min × 3 times, 100% ethanol 15 min × 3 times). Xylene was used to make tissue more clear (15 min × 2 times). Then the tissues were dipped in wax two times at 60 °C, each time 1–2 hours and paraffin embedded at the same temperature. Fat and liver tissue blocks were cut into 5-micro sections and stained with hematoxylin and eosin.
2.6 Analysis of fecal fatty acids
The feces of rats were collected weekly and lyophilized. Fat in feces was extracted. Then after methyl esterification, the kind and content of fatty acids were detected by gas chromatography (GC). Feces was accurately 0.5 g lyophilized and pulverized and joined in 9 mL chloroform–methanol mixed solution. Then it was stored in a refrigerator overnight at −80 °C and filtered into a test tube with a stopper. The crude extract was added to 0.2 times the volume of chloroform–methanol–0.29% NaCl solution and mixed well. After waiting a moment to stratification, the top was aspirated and the interface was cleaned with a small amount of chloroform–methanol mixed solution. Then the appropriate amount of methanol was added and homogenised. In the end, after adding an appropriate amount of anhydrous Na2SO4, and filtering again, evaporation was done with a stream of nitrogen. A 4% sulfuric acid–methanol solution was added in the test tube, it was then placed in a pre-heated water bath at 75 °C, and the reaction heated for 1 h. After completion of the reaction 2 mL hexane and 5 mL distilled water were added to the system. When stratification appeared the supernatant was moved into a small beaker with anhydrous sodium sulfate. Finally the organic syringe filters (0.22 μm) were mounted on syringes and filtered into a centrifuge tube for GC measurements.
2.7 Research methods of detecting mRNA expression
Rats were anesthetized and euthanized, and adipose samples were collected at the end of the experiment. The total mRNA was extracted using trizol (Takara). They were then reverse transcribed into cDNA. Finally the gene expressions of these related genes were detected by RT-PCR (Bio-Rad). The method of operation was according to instructions and the dye used was SYBR Green. Gene specific primers used are given in Table 5. Relative quantification of gene expression with real-time PCR data was calculated relative to GAPDH.
2.8 Statistical analysis
Results are presented as means with their standard errors. Statistical analysis was performed using the SPSS program. Data were analyzed by one-way ANOVA. Differences between the groups were established using the least significant difference (LSD) test and the criterion for statistical significance was set at p < 0.05.
3. Results
3.1 Changes of rat body weight, food utilization and Lee's index
The changes in body weight, food utilization and Lee's index are shown in Table 1. The change of body weight in 6 weeks is shown in Fig. 1. The final body weight of animals in the MC group was significantly higher compared with the NC group, while other groups except OC, TWL, TPSL had a significant reduction in body weight compared with the MC group. The food utilization of rats in the MC group was significantly higher compared with the NC group and other groups except TPSL had a significant reduction in body weight compared with the MC group. Lee's index could reflect the degree of obesity. The result showed that the Lee's index of the MC group was significantly higher compared with the NC group, while all medicated groups had a significant reduction compared with the MC group.
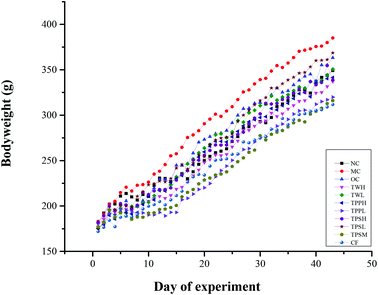 |
| Fig. 1 Changes in body weights of the SD rats when fed with a suitable feed for 42 days. Data are presented as the mean from twelve rats per group. NC was fed the standard chow plus saline; MC was fed a high-fat diet with 79% of the standard chow, 10% lard, 10% egg yolk powder, 0.5% cholesterol, 0.5% cholate content plus saline; OC was fed a high-fat diet feed plus orlistat capsule 40 mg per kg BW; TWH was fed a high-fat diet feed plus total water extract 800 mg per kg BW; TWL was fed a high-fat diet feed plus total water extract 400 mg per kg BW; TPPH was fed a high-fat diet feed plus tea polyphenols 800 mg per kg BW; TPPL was fed a high-fat diet feed plus tea polyphenols 400 mg per kg BW; TPSH was fed a high-fat diet feed plus tea polysaccharide 800 mg per kg BW; TPSL was fed a high-fat diet feed plus tea polysaccharide 400 mg per kg BW; TPSM was fed a high-fat diet feed plus the complex of tea polysaccharide and polyphenols (1 : 1) 800 mg per kg BW; CF was fed a high-fat diet feed plus caffeine 400 g per kg BW. | |
3.2 Body fat weight and fat index
After 6 weeks of the experiment, the epididymal and perirenal adipose tissues were collected and measured. Table 2 indicates that the MC group had a significantly higher weight of two parts of fat when compared with the NC group; similar results also were found in the fat index, while the body fat weight and fat index of all medicated groups except OC and TPSL had a significant reduction compared with the MC group.
Table 2 Some body fat figures of rats in each groupa
Groups |
Fat weight (g) |
Fat index (%) |
Number of cells |
All values are means ± SD (n = 12). Compared with the NC group and MC group #P < 0.05. Compared with the MC group and drug group *P < 0.05.
|
NC |
6.24 ± 2.51 |
1.96 ± 0.47 |
128.45 ± 15.33 |
MC |
10.99 ± 2.24# |
2.85 ± 0.45# |
56.67 ± 5.05# |
OC |
8.61 ± 3.11b |
2.56 ± 0.68 |
110.82 ± 14.68* |
TWH |
6.88 ± 2.54* |
2.02 ± 0.63* |
116.83 ± 31.29* |
TWL |
7.21 ± 2.21* |
2.01 ± 0.61* |
86.08 ± 21.90* |
TPPH |
7.31 ± 2.70* |
2.33 ± 0.39* |
110.83 ± 18.88* |
TPPL |
4.77 ± 1.32* |
1.80 ± 0.26* |
116.9 ± 12.35* |
TPSH |
7.29 ± 2.95* |
2.35 ± 0.63* |
99.55 ± 32.90* |
TPSL |
9.32 ± 1.63 |
2.53 ± 0.32 |
104.16 ± 32.92* |
TPSM |
6.57 ± 1.08* |
2.24 ± 0.62* |
143.18 ± 30.15* |
CF |
5.22 ± 1.81* |
1.81 ± 0.60* |
118.36 ± 23.50* |
3.3 Serum lipids and antioxidants
Serum lipids and antioxidant profiles are shown in Table 3. The triglyceride and cholesterol levels of rats in the MC group increased significantly compared with the NC group. The serum triglyceride levels of the TWL group had no significant reduction compared with the MC group. Moreover, serum cholesterol levels of TWH and TPPH groups not yet had a significant reduction compared with the MC group. However, the serum LDL-C levels in all the groups were significantly reduced except for the TWH, TWL and TPPH groups. It was interesting that the serum HDL-C levels of only the TPSL and TPSM groups were significantly higher compared with the MC group, but the serum HDL-C level of the CF group was lower than the MC group. In order to investigate the relationship between serum antioxidant and obesity, the levels of MDA and T-SOD were analyzed. The levels of serum MDA of the MC group were significantly higher compared with the NC group, but only OC, TPPH, TPSH, TPSL and TPSM groups had attenuated levels of MDA. On the other hand the levels of serum T-SOD of the MC group were significantly lower compared with the NC group, and the levels of the T-SOD of TWH, TPPL, TPSH and TPSM were significantly higher compared with the MC group. Serum leptin and insulin levels were elevated in rats fed with a high fat diet, and OC, TWL, TPPL, TPSH and TPSM significantly lowered serum leptin levels compared to the MC group.
Table 3 Some serum figures of rats in each groupa
Groups |
TC (mmol L−1) |
TG (mmol L−1) |
HDLC (mmol L−1) |
LDLC (mmol L−1) |
MDA (mmol L−1) |
T-SOD (U ml−1) |
LEP (μg L−1) |
All values are means ± SD (n = 12). Compared with the NC group and MC group #P < 0.05. Compared with the MC group and drug group *P < 0.05.
|
NC |
2.85 ± 0.60 |
0.64 ± 0.14 |
1.02 ± 0.14 |
1.69 ± 0.65 |
3.25 ± 1.10 |
271.85 ± 53.22 |
6.13 ± 0.77 |
MC |
4.32 ± 0.88# |
1.53 ± 0.22# |
1.04 ± 0.11 |
3.04 ± 0.93# |
3.93 ± 1.08# |
193.04 ± 48.02# |
7.43 ± 0.95# |
OC |
3.12 ± 0.77* |
1.01 ± 0.27* |
1.13 ± 0.45 |
1.60 ± 0.51* |
2.79 ± 0.98* |
243.82 ± 63.52 |
6.19 ± 0.68 |
TWH |
3.76 ± 0.79* |
0.86 ± 0.08* |
1.23 ± 0.34 |
2.25 ± 0.77 |
4.13 ± 0.93 |
292.23 ± 50.02* |
6.88 ± 0.80 |
TWL |
3.62 ± 0.59* |
1.33 ± 0.16 |
0.99 ± 0.49 |
2.05 ± 0.62 |
3.77 ± 0.63 |
189.55 ± 66.88 |
5.99 ± 0.90* |
TPPH |
4.23 ± 1.08 |
0.89 ± 0.19* |
1.23 ± 0.21 |
2.57 ± 0.54 |
3.14 ± 0.66* |
225.81 ± 68.62 |
7.00 ± 0.58 |
TPPL |
2.81 ± 0.39* |
0.90 ± 0.12* |
1.14 ± 0.64 |
1.51 ± 0.36* |
3.96 ± 0.77 |
269.57 ± 82.01* |
5.95 ± 0.84* |
TPSH |
2.95 ± 0.99* |
0.93 ± 0.21* |
1.05 ± 0.29 |
1.93 ± 0.19* |
3.38 ± 0.39* |
276.44 ± 57.45* |
6.32 ± 0.74* |
TPSL |
2.78 ± 0.36* |
0.94 ± 0.20* |
1.75 ± 0.56* |
1.51 ± 0.40* |
3.59 ± 0.76* |
254.22 ± 69.10 |
6.94 ± 0.27 |
TPSM |
2.64 ± 0.50* |
0.73 ± 0.19* |
1.79 ± 0.24* |
1.52 ± 0.43* |
3.39 ± 0.91* |
289.87 ± 57.4* |
6.15 ± 0.76* |
CF |
2.78 ± 0.36* |
0.86 ± 0.16* |
0.89 ± 0.31 |
0.94 ± 0.18* |
4.66 ± 1.65 |
238.74 ± 61.95 |
6.75 ± 0.61 |
3.4 Histological analysis of liver and epididymal white adipose tissue
The histology of rat epididymal white adipose tissue is shown in Fig. 2, and the numbers of adipocytes within the same field are expressed in Table 2. The adipocyte size of the MC group was significantly bigger than the NC group, and the numbers of fat cells were significantly less than the NC group. For all medicated groups, their adipocyte sizes were significantly smaller than the MC group, in other words their numbers of fat cells were significantly less than the MC group. Changes in the degree of infiltration of lipid droplets in the liver are shown in Fig. 3. The representative liver section of the MC group shows increased infiltration of lipid droplets, leading to hepatic steatosis conditions. While the lipid droplet infiltration in the representative liver section of the other group except CF is markedly reduced, even the liver histology section of TPSH and TPSL groups is free from lipid droplets.
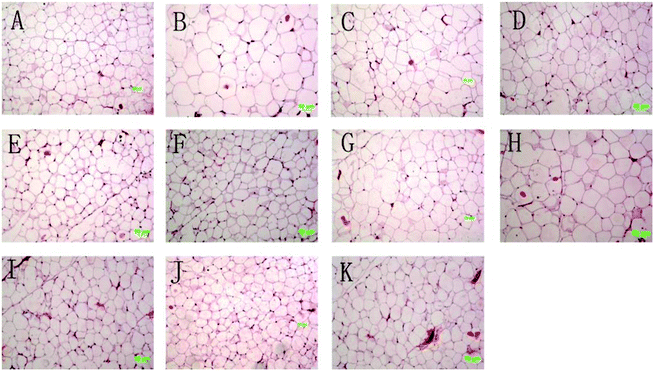 |
| Fig. 2 Histopathological analysis of adipose tissues. All pictures were taken at 200 times. The letters A–K in the figure represent in proper order the following groups: NC, MC, OC, TWH, TWL, TPPH, TPPL, TPSH, TPSL, TPSM and CF groups. | |
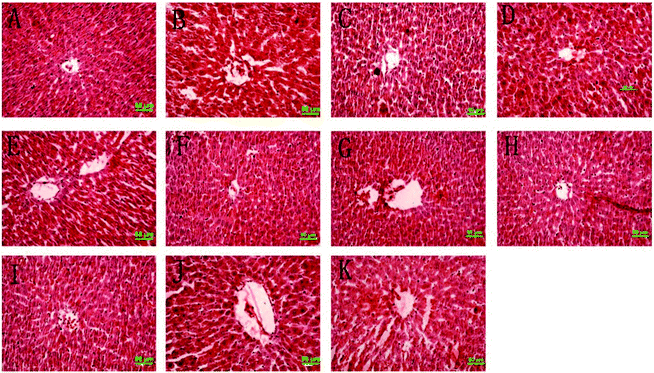 |
| Fig. 3 Histopathological analysis of liver. All pictures were taken at 200 times. The letters A–K in the figure represent in proper order the following groups: NC, MC, OC, TWH, TWL, TPPH, TPPL, TPSH, TPSL, TPSM and CF groups. | |
3.5 Rat feces fatty acid analysis
The rat feces fatty acids were determined by GC, and the result is expressed in Table 4. According to the result we found that the total fatty acids were mainly composed of palmitic acid, stearic acid, oleic acid and linoleic acid. Both the total amount of fatty acids and specific ones in rat feces were significantly increased except in the TPSL group when compared to the MC group, which indicates that the green tea extract, polyphenols, polysaccharides, and caffeine can inhibit the absorption of fatty acids in the body.
Table 4 Fatty acids in rat fecesa
Groups |
Palmitic acid (mg g−1) |
Stearic acid (mg g−1) |
Oleic acid (mg g−1) |
Linoleic acid (mg g−1) |
Total acid (mg g−1) |
All values are means ± SD (n = 12). Compared with the MC group and drug group *P < 0.05.
|
NC |
0.12 ± 0.01 |
0.11 ± 0.01 |
0.06 ± 0.01 |
0.06 ± 0.00 |
0.3 ± 0.04 |
MC |
0.43 ± 0.01 |
0.28 ± 0.02 |
0.34 ± 0.01 |
0.22 ± 0.03 |
1.4 ± 0.22 |
OC |
0.60 ± 0.04* |
0.30 ± 0.01* |
0.44 ± 0.01* |
0.28 ± 0.01* |
1.8 ± 0.22* |
TWH |
0.93 ± 0.05* |
0.60 ± 0.04* |
0.73 ± 0.02* |
0.38 ± 0.03* |
2.81 ± 0.22* |
TWL |
0.92 ± 0.05* |
0.60 ± 0.04* |
0.75 ± 0.01* |
0.42 ± 0.03* |
2.91 ± 0.25* |
TPPH |
0.56 ± 0.04* |
0.30 ± 0.02* |
0.47 ± 0.01* |
0.29 ± 0.04* |
1.99 ± 0.29* |
TPPL |
0.63 ± 0.03* |
0.40 ± 0.02* |
0.48 ± 0.02* |
0.31 ± 0.03* |
1.87 ± 0.26* |
TPSH |
0.71 ± 0.02* |
0.40 ± 0.02* |
0.56 ± 0.01* |
0.38 ± 0.02* |
2.25 ± 0.23* |
TPSL |
0.48 ± 0.03 |
0.29 ± 0.01 |
0.37 ± 0.02 |
0.25 ± 0.02 |
1.51 ± 0.20 |
TPSM |
0.92 ± 0.04* |
0.60 ± 0.02* |
0.79 ± 0.02* |
0.51 ± 0.03* |
2.95 ± 0.02* |
CF |
0.73 ± 0.03* |
0.50 ± 0.01* |
0.60 ± 0.02* |
0.39 ± 0.01* |
2.37 ± 0.24* |
Table 5 Primer sequences used in the study
|
Gene primer sequence |
1 |
LEP |
F: 5′-AAAAGAACGGGACAGAACA-3′ |
R: 5′-TGACCAAGGTGACATAGCG-3′ |
2 |
IL-6 |
F: 5′-TGCCTTCTTGGGACTGATG-3′ |
R: 5′-ATACTGGTCTGTTGTGGGTG-3′ |
3 |
TNF-α |
F: 5′-CCACGCTCTTCTGTCTACTG-3′ |
R: 5′-GCTACGGGCTTGTCACTC-3′ |
4 |
GAPDH |
F:5′-GCAAGTTCAACGGCACAG-3′ |
R:5′-GCCAGTAGACTCCACGACAT-3′ |
3.6 The result of detecting mRNA expression
The mRNA expression levels of LEP, IL-6 and TNF-α were determined in white adipose tissue (Fig. 4). Compared to the NC group, rats fed with a high fat diet caused the up-regulation of LEP, IL-6 and TNF-α genes. OC, TWH, TWL, TPPH, TPPL, TPSL, TPSM and CF markedly reduced the expression levels of LEP compared to the MC group (Fig. 4A). However, only OC, TPPH, TPSH, TPSL and TPSM significantly attenuated the expression of IL-6. In addition, Fig. 4C indicates that all the medicated groups except OC and CF groups showed a lower level of TNF-α expression compared to the MC group.
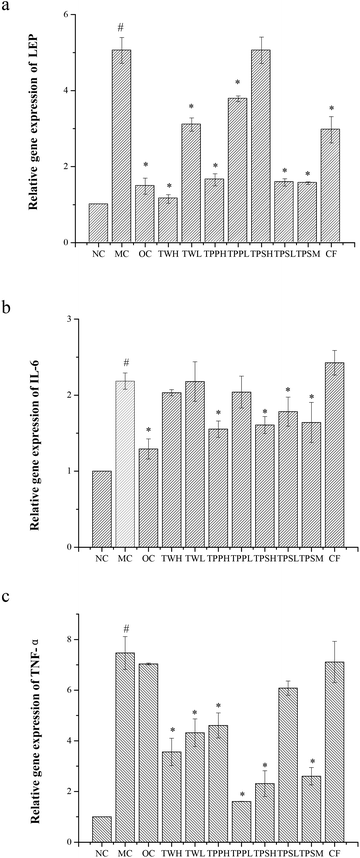 |
| Fig. 4 Effects of the green tea extract on the mRNA expression level of LEP (a), IL-6 (b), TNF-α (c) in the adipose tissues. NC, MC, OC, TWH, TWL, TPPH, TPPL, TPSH, TPSL, TPSM and CF groups. Compared with the NC group and MC group #P < 0.05. Compared with the MC group and drug group *P < 0.05. | |
4. Discussion
Several studies have evaluated the effects of green tea extracts on the development of obesity. However, there is extremely limited research focused on the relationship between the bioactive substances of green tea and their anti-obesity effects.19,25–28 Therefore, in the present study we isolate polyphenols, polysaccharides and caffeine from green tea and then investigate their anti-obesity capabilities. According to the present study, we found that TWH, TPPH, TPPL, TPSH, TPSM and CF could significantly prevent rat body weight gain compared with the model group, but TWL and TPSL did not change the rat body weight. There might be dose-dependent effects of the tea water extract and polysaccharides, and a high dose of the green tea water extract or polysaccharide is more efficacious in preventing obesity.
Sayama et al.32–34 reported that green tea could reduce the weight of mice by suppression of food intake. Kao et al.35 also observed reduction in food intake after the administration of EGCG which was contained in tea polyphenols. We also found that the decrease in weight gain induced by polyphenols, polysaccharides, caffeine or the complex of tea polysaccharides and polyphenols, respectively, is relevant to food utilization.
Zheng et al.33 found that caffeine and theanine could suppress body weight and body fat, while catechins did not change these parameters but reduced serum triglycerides and free fatty acids in female ICR mice. Our results suggested that polyphenols, polysaccharides, caffeine or the complex of tea polysaccharides and polyphenols can significantly reduce serum triglyceride levels. Furthermore, tea polysaccharides could effectively reduce serum LDL-C levels.
In this study, we demonstrate that polyphenols, polysaccharides, caffeine or the complex of tea polysaccharides and polyphenols can inhibit the absorption of fatty acids in the body. This suggests, in agreement with previous reports, that green tea extracts present anti-obesity properties.36
Accumulated evidence indicates that obesity induced by high fat food can increase production of obesity related inflammation cytokines, such as leptin, IL-6, TNF-α, etc. Matsubara et al. reported that a high-fat diet resulted in an increase in the content of saturated fatty acids that could lead to an upward trend of fat cytokine mRNA such as leptin and adipsin.37Our studies revealed that polysaccharides, caffeine or the complex of tea polysaccharides and polyphenols markedly reduced the expression levels of leptin in white adipose tissue except the high dose of polyphenol. Some studies have reported that serum leptin levels in obese patients were significantly higher than the control group, while several studies have shown that serum leptin levels of obese patients were significantly lower.38,39Our results showed that suitable doses of the green tea extract, polysaccharides, or polyphenols significantly lowered serum leptin levels. Moreover, it was shown that polysaccharides and polyphenols were synergistic in reduction of serum leptin levels. Wu et al. suggested that fruit polyphenols exerted potential anti-inflammatory effects.11,40 In the present study we found that both green tea extracts, polysaccharides, and polyphenols revealed anti-inflammatory effects. In addition, polysaccharides and polyphenols were synergistic in their anti-inflammatory activity, which means that the polysaccharide combination with polyphenol could better strengthen the anti-obesity function than their individual effects.
In conclusion, coarse old green tea extracts prevent body weight gain in male SD rats; polyphenols, caffeine, and especially polysaccharides may play important part. The present experiments demonstrated that multiple factors in green tea contributed to anti-obesity effects. All the main ingredients in green tea contributed to the anti-obesity function and every ingredient in green tea played many beneficial roles to achieve weight loss effects such as reducing food utilization, lowering serum triglyceride levels, inhibiting the absorption of fatty acids, regulating some relevant genes’ expression and so on. In addition, tea polyphenols, polysaccharides, or caffeine effectively inhibit the absorption of fatty acids and markedly reduce the expression levels of inflammatory genes. Furthermore, polysaccharides and polyphenols were synergistic in reduction of serum leptin levels and in anti-inflammatory activity. Therefore the polysaccharide combination with polyphenols might be a potential therapy to treat obesity, and further clinical studies are needed.
Conflicts of interest
The authors declare no conflict of interest.
Acknowledgements
The research was supported by the Project of the Ministry of Science and Technology of the People's Republic of China (no. 2012BAD33B08).
References
- E. Dirinck, P. G. Jorens, A. Covaci, T. Geens, L. Roosens, H. Neels, I. Mertens and L. Van Gaal, Obesity, 2011, 19, 709–714 CrossRef CAS PubMed.
- K. M. Flegal, M. D. Carroll, B. K. Kit and C. L. Ogden, JAMA, J. Am. Med. Assoc., 2012, 307, 491–497 CrossRef PubMed.
- E. Stimpson, J. Patel, M. Kittleson, M. Rafiei, A. Osborne, F. Lee, D. H. Chang, A. Hage, D. Ramzy, L. Czer, M. Hamilton and J. Kobashigawa, J. Heart Lung Transpl., 2013, 32, S212–S212 CrossRef PubMed.
- R. H. Eckel, S. E. Kahn, E. Ferrannini, A. B. Goldfine, D. M. Nathan, M. W. Schwartz, R. J. Smith and S. R. Smith, J. Clin. Endocrinol. Metab., 2011, 96, 1654–1663 CrossRef CAS PubMed.
- C. N. Lumeng and A. R. Saltiel, J. Clin. Invest., 2011, 121, 2111–2117 CrossRef CAS PubMed.
- J. O. Hill, H. R. Wyatt and J. C. Peters, Circulation, 2012, 126, 126–132 CrossRef PubMed.
- I. Imayama, C. M. Ulrich, C. M. Alfano, C. Wang, L. Xiao, M. H. Wener, K. L. Campbell, C. Duggan, K. E. Foster-Schubert, A. Kong, C. E. Mason, C. Y. Wang, G. L. Blackburn, C. E. Bain, H. J. Thompson and A. McTiernan, Cancer Res., 2012, 72, 2314–2326 CrossRef CAS PubMed.
- E. Colman, J. Golden, M. Roberts, A. Egan, J. Weaver and C. Rosebraugh, N. Engl. J. Med., 2012, 367, 1577–1579 CrossRef CAS PubMed.
- M. A. Jordan, Drug Discovery Today, 2013, 107–155 CAS.
-
G. A. Mohamed, S. R. M. Ibrahim, E. S. Elkhayat and R. S. El Dine, Bulletin of Faculty of Pharmacy, Cairo University, 2014, DOI:10.1016/j.bfopcu.2014.05.001.
- T. Wu, X. Qi, Y. Liu, J. Guo, R. Zhu, W. Chen, X. Zheng and T. Yu, Food Chem., 2013, 141, 482–487 CrossRef CAS PubMed.
- S. P. J. Namal Senanayake, J. Funct. Foods, 2013, 5, 1529–1541 CrossRef CAS PubMed.
- M. Friedrich, K. J. Petzke, D. Raederstorff, S. Wolfram and S. Klaus, Int. J. Obes., 2012, 36, 735–743 CrossRef CAS PubMed.
- K. Huvaere, J. H. Nielsen, M. Bakman, M. Hammershoj, L. H. Skibsted, J. Sorensen, L. Vognsen and T. K. Dalsgaard, J. Agric. Food Chem., 2011, 59, 8718–8723 CrossRef CAS PubMed.
- S. Sae-tan, K. A. Grove and J. D. Lambert, Pharmacol. Res., 2011, 64, 146–154 CrossRef CAS PubMed.
- A. G. Dulloo, C. Duret, D. Rohrer, L. Girardier, N. Mensi, M. Fathi, P. Chantre and J. Vandermander, Am. J. Clin. Nutr., 1999, 70, 1040–1045 CAS.
- E. M. Kovacs, M. P. Lejeune, I. Nijs and M. S. Westerterp-Plantenga, Br. J. Nutr., 2004, 91, 431–437 CrossRef CAS PubMed.
- T. Nagao, Y. Komine, S. Soga, S. Meguro, T. Hase, Y. Tanaka and I. Tokimitsu, Am. J. Clin. Nutr., 2005, 81, 122–129 CAS.
- T. M. Rains, S. Agarwal and K. C. Maki, J. Nutr. Biochem., 2011, 22, 1–7 CrossRef CAS PubMed.
- T. M. Jurgens, A. M. Whelan, L. Killian, S. Doucette, S. Kirk and E. Foy, Cochrane Database Syst. Rev., 2012, 12 Search PubMed , Cd008650.
- I. Ikeda, R. Hamamoto, K. Uzu, K. Imaizumi, K. Nagao, T. Yanagita, Y. Suzuki, M. Kobayashi and T. Kakuda, Biosci., Biotechnol., Biochem., 2005, 69, 1049–1053 CrossRef CAS.
- K. A. Grove and J. D. Lambert, J. Nutr., 2010, 140, 446–453 CrossRef CAS PubMed.
- S. Wolfram, D. Raederstorff, Y. Wang, S. R. Teixeira, V. Elste and P. Weber, Ann. Nutr. Metab., 2005, 49, 54–63 CrossRef CAS PubMed.
- J. J. Choo, J. Nutr. Biochem., 2003, 14, 671–676 CrossRef CAS PubMed.
- C. L. Shen, S. Chanjaplammootil, J. K. Yeh, J. J. Cao, M. C. Chyu, R. Y. Dagda and J. S. Wang, FASEB J., 2011, 25 Search PubMed.
- C. H. Hsu, T. H. Tsai, Y. H. Kao, K. C. Hwang, T. Y. Tseng and P. Chou, Clin. Nutr., 2008, 27, 363–370 CrossRef CAS PubMed.
- M. C. Lonac, J. C. Richards, M. M. Schweder, T. K. Johnson and C. Bell, Obesity, 2011, 19, 298–304 CrossRef CAS PubMed.
- O. J. Phung, W. L. Baker, L. J. Matthews, M. Lanosa, A. Thorne and C. I. Coleman, Am. J. Clin. Nutr., 2010, 91, 73–81 CrossRef PubMed.
- C. Tian, X. Ye, R. Zhang, J. Long, W. Ren, S. Ding, D. Liao, X. Jin, H. Wu, S. Xu and C. Ying, PLoS One, 2013, 8, e53796 CAS.
- C. Lu, W. Zhu, C. L. Shen and W. Gao, PLoS One, 2012, 7, e38332 CAS.
- L. Wang, L.-H. Gong, C.-J. Chen, H.-B. Han and H.-H. Li, Food Chem., 2012, 131, 1539–1545 CrossRef CAS PubMed.
- S. Sae-tan, K. A. Grove and J. D. Lambert, Pharmacol. Res., 2011, 64, 146–154 CrossRef CAS PubMed.
- G. Zheng, K. Sayama, T. Okubo, L. R. Juneja and I. Oguni, In Vivo, 2004, 18, 55–62 CAS.
- K. Sayama, S. Lin, G. Zheng and I. Oguni, In Vivo, 2000, 14, 481–484 CAS.
- Y. H. Kao, R. A. Hiipakka and S. Liao, Endocrinology, 2000, 141, 980–987 CAS.
- M. Yang, C. Wang and H. Chen, J. Nutr. Biochem., 2001, 12, 14–20 CrossRef CAS.
- Y. Matsubara, K. Kano, D. Kondo, H. Mugishima and T. Matsumoto, Ann. Nutrition Metab., 2009, 54, 258–267 CrossRef CAS PubMed.
- M. G. Myers Jr., R. L. Leibel, R. J. Seeley and M. W. Schwartz, Trends Endocrinol. Metab., 2010, 21, 643–651 CrossRef PubMed.
- M. Polotsky, A. S. Elsayed-Ahmed, L. Pichard, C. C. Harris, P. L. Smith, H. Schneider, J. P. Kirkness, V. Polotsky and A. R. Schwartz, J. Appl. Physiol., 2012, 112, 1637–1643 CrossRef CAS PubMed.
- T. Wu, Z. Yu, Q. Tang, H. Song, Z. Gao, W. Chen and X. Zheng, Food Funct., 2013, 4, 1654–1661 CAS.
|
This journal is © The Royal Society of Chemistry 2015 |
Click here to see how this site uses Cookies. View our privacy policy here.