DOI:
10.1039/C4QO00299G
(Research Article)
Org. Chem. Front., 2015,
2, 110-113
Asymmetric synthesis of poly-substituted spirocyclohexane oxindole via a squaramide catalyzed cascade Michael–Michael–aldol sequence†
Received
13th November 2014
, Accepted 26th December 2014
First published on 30th December 2014
Abstract
A squaramide-catalyzed Michael–Michael–aldol cascade sequence of three readily accessible substrates (1,3-dicarbonyl compound, nitroalkene and methyleneindolinone) was developed. The reactions led to a series of enantioenriched spirocyclohexane oxindoles bearing six contiguous stereocenters in good yields (up to 85%) and with excellent stereoselectivities (>20
:
1 dr, >99% ee).
Introduction
The prevalence of spirocyclic oxindole architecture in a variety of natural products and synthetic bioactive compounds has underlined the importance of general asymmetric methods for their efficient construction.1 In particular, spirocyclohexane oxindoles have intrigued the chemistry community for years, with a unique synthetic challenge. Significant efforts have been made for the stereoselective construction of the requisite all-carbon spiroquaternary and other multiple stereocenters for such complex structures.2 Recently, a number of elegant asymmetric assemblies of spirocyclohexane oxindoles3,4 were accomplished by means of organocatalytic domino reactions,5 in which small organic molecules are used to mediate multiple chemical transformations in a consecutive and protecting group free fashion. The reactions are conducted under mild conditions with remarkable step, atom and redox economy. Notably, these established methods are mainly based on chiral amine catalyzed syntheses through cascade reactions3 or Diels–Alder reactions.4 Nevertheless, to the best of our knowledge, the asymmetric synthesis of spirocyclohexane oxindoles bearing six contiguous stereocenters is rare to date. Steric hindrance arising from the multiple crowded groups of cyclohexane, in combination with the difficulties in constructing the all-carbon spiroquaternary stereocenter with an excellent stereocontrol, presents an extremely hard task to design highly stereoselective approaches towards fully substituted spirocyclohexane oxindoles. Therefore, new strategies for the efficient and stereospecific synthesis of fully substituted spirocyclohexane oxindoles from readily available starting materials are still in great demand.
Previously, we reported several organocatalyzed cascade reactions for the asymmetric preparation of densely substituted six-membered rings bearing contiguous stereocenters using easily accessible starting materials.6 Inspired and encouraged by these results, we reasoned that 1,3-dicarbonyl compounds 1 not only could undergo Michael addition with both nitroalkenes 2 and methyleneindolinones 3 but also could serve as electrophiles for an intramolecular aldol or Henry reaction. The conjugated additions of dicarbonyl compounds to nitroalkenes or oxindoles catalyzed by bifunctional catalysts have been well studied.7 As such, these Michael adducts (I, II) would attack another Michael acceptor under identical conditions in the following step. Consequently, two types of multifunctional spirocyclic oxindoles (4, 5) would be generated from these simple substrates through a cascade reaction (Scheme 1). We herein disclose an organocatalytic cascade Michael/Michael/aldol sequence for the construction of spirocyclohexane oxindole bearing six adjacent stereocenters in a highly efficient and stereospecific manner using β-dicarbonyl compound, nitroalkene and methyleneindolinone under mild conditions. It is worth noting that Enders and co-workers reported the synthesis of spiropyrazolones using a similar strategy while this manuscript was in preparation.8
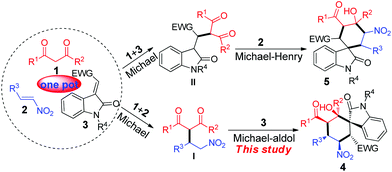 |
| Scheme 1 Asymmetric organocatalytic cascade strategy for the privileged six-membered rings. | |
Results and discussion
Initially, the cascade Michael–Michael–aldol sequence was set up in a one-pot fashion using acetylacetone 1a, nitroalkene 2a and unprotected methyleneindolinone 3a in DCM at room temperature with 5 mol% of a chiral squaramide as the catalyst. Unfortunately, no desired spirocyclohexane oxindole was obtained but the Michael adduct of 1,3-diketone and nitroalkene was isolated in nearly quantitative yield after 24 hours (Table 1, entry 1). Then, different protecting groups on the N atom of methyleneindolinone were tested. N-Methyl and N-benzyl protected substrates showed less reactivity in this cascade reaction (Table 1, entries 2, 3). N-Acetyl methyleneindolinone furnished the desired product in 15% yield with 1
:
1 dr and >99% ee (Table 1, entry 4). To our delight, when N-Boc protected methyleneindolinone was examined, the desired product 4a was obtained in 25% yield with >20
:
1 dr and >99% ee. However the chemoselectivity (4a
:
5a = 1
:
2.5) and the diastereoselectivity of 5a (2.5
:
1) were very low (Table 1, entry 5). To improve the yield and selectivities, the cascade reaction was performed in a stepwise-fashion by adding N-Boc-protected methyleneindolinone after nitroalkene was consumed completely.9 Gratifyingly, a much higher yield (85%) of 4a was achieved, along with excellent stereoselectivity (>20
:
1 dr, >99% ee) and chemoselectivity (4a
:
5a >20
:
1) (Table 1, entry 6). Other solvents, such as CHCl3, CCl4, THF and toluene, gave slightly lower yields compared to DCM (Table 1, entries 7–10). Taken together, the proposed cascade reaction could be realized by adding N-Boc-protected methyleneindolinone after the first Michael addition was completed in the presence of 5 mol% Q-6 in DCM at room temperature. (For the reaction of isolated Michael addition adducts with methyleneindolinone catalyzed by achiral base, see ESI†).
Table 1 Optimization of cascade reaction conditionsa
With the optimized conditions in hand, the substrate scope was explored as summarized in Fig. 1. In general, the reaction could produce the corresponding products in moderate to good yields (up to 85%) and with excellent stereoselectivities (>20
:
1 dr, >99% ee, in all cases). Aryl substituted nitroalkenes bearing either electron-withdrawing or electron-donating groups on the phenyl ring were well tolerated (Fig. 1, 4a–4f), affording the desired compounds in high yields (71–85%). Nitroalkenes with alkyl substitutions, proven to be not good substrates, gave moderate yields but poor selectivities (see ESI† for details). Desirable results (77–87% yields) were obtained when nitroalkenes bearing heteroaromatic rings were employed (Fig. 1, 4g, 4h). Additionally, this cascade reaction was amenable to various N-Boc protected methyleneindolinones, providing the desired products in moderate to good yields (32–79%) (Fig. 1, 4i–4l). It is worth noting that the employment of ethyl acetoacetate (Fig. 1, 4m–4o) and ethyl benzoylacetate (Fig. 1, 4p) also afforded the expected spirocyclic oxindoles with excellent stereoselectivities (>20
:
1 dr, >99% ee). The absolute configuration of the cascade Michael–Michael–aldol reaction product was unambiguously assigned based on the single-crystal X-ray analysis of the N-Boc-deprotected product of 4b (Fig. 2).10
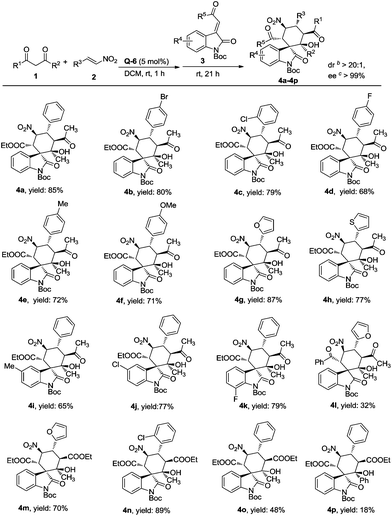 |
| Fig. 1 Substrate scope.a aReaction conditions: the reaction was performed using 0.22 mmol of 1, 0.20 mmol of 2, 5 mol% of Q-6 and 0.3 mmol of 3 in DCM (1.0 M) at room temperature. The isolated yield. bdr was determined by crude 1H NMR. cThe ee value of the major product was determined by chiral HPLC. | |
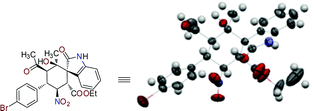 |
| Fig. 2 X-ray structure of the N-Boc-deprotected product of 4b.11 | |
Encouraged by the above results, we next sought to diversify the six-membered spirocyclic oxindoles by reducing the nitro-group or removing the N-Boc-group of the obtained products selectively (Scheme 2). The reactions proceeded smoothly to afford the desired compounds (Scheme 2, 6o, 7o, 8o). Notably, the newly formed amine groups in the products 7o and 8o could serve as a convenient handle for further transformations.
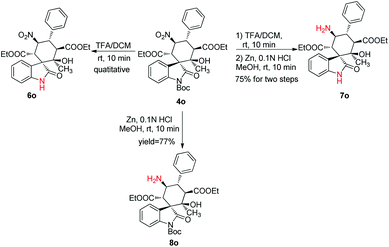 |
| Scheme 2 Transformations of the obtained spirocyclohexane oxindoles. | |
To further demonstrate the practicality and efficiency of this cascade Michael–Michael–aldol sequence, a gram-scale synthesis of poly-substituted spirocyclohexane oxindole was achieved in 76% yield with excellent stereoselectivity (>20
:
1 dr, >99% ee) (Scheme 3). Although a prolonged reaction time was needed, this result illustrated a very promising outlook for the applicability of this cascade reaction. When a lower catalyst loading (2 mol%) was applied in the gram-scale synthesis, a high dr (>20
:
1) and an excellent ee (>99%) was achieved but a lower yield was observed regretfully (see ESI† for details).
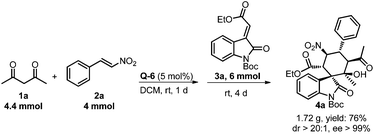 |
| Scheme 3 Gram-scale cascade reaction. | |
Conclusions
In summary, we have developed an organocatalytic Michael–Michael–aldol sequence that generated a series of polysubstituted spirocyclohexane oxindoles using a bifunctional squaramide as the catalyst. These structurally complex scaffolds were obtained in good yields (up to 85%) with excellent stereoselectivities (>20
:
1 dr, >99% ee in all cases), featuring the efficient formation of three C–C bonds and six contiguous stereocenters including one all-carbon spiroquaternary stereocenter. We envision that these important skeletons would show great promise in the further synthesis of bioactive molecules.
Acknowledgements
Financial support from the National Natural Science Foundation of China (21302020, 21342009 and 21472019) is gratefully acknowledged.
Notes and references
- For selected reviews on spirocyclic oxindoles, see:
(a) R. M. Williams and R. J. Cox, Acc. Chem. Res., 2003, 36, 127 CrossRef CAS PubMed;
(b) H. Lin and S. J. Danishefsky, Angew. Chem., Int. Ed., 2003, 42, 36 CrossRef CAS;
(c) C. V. Galliford and K. A. Scheidt, Angew. Chem., Int. Ed., 2007, 46, 8748 CrossRef CAS PubMed;
(d) J. Yang, X. Z. Wearing, P. W. Le Quesne, J. R. Deschamps and J. M. Cook, J. Nat. Prod., 2008, 71, 1431 CrossRef CAS PubMed;
(e) G. S. Singh and Z. Y. Desta, Chem. Rev., 2012, 112, 6104 CrossRef CAS PubMed. For selected examples on spirocyclic oxindoles, see:
(f)
J.-J. Liu and Z. Zhang, (Hoffmann-La Roche AG) PCT Int. Appl, WO2008/055812, 2008 Search PubMed;
(g) T. Onishi, P. R. Sebahar and R. M. Williams, Org. Lett., 2003, 5, 3135 CrossRef CAS PubMed;
(h) B. M. Trost, N. Cramer and H. Bernsmann, J. Am. Chem. Soc., 2007, 129, 3086 CrossRef CAS PubMed;
(i) X. Zhou, T. Xiao, Y. Iwama and Y. Qin, Angew. Chem., Int. Ed., 2012, 51, 4909 CrossRef CAS PubMed;
(j) B. M. Trost, D. A. Bringley, T. Zhang and N. Cramer, J. Am. Chem. Soc., 2013, 135, 16720 CrossRef CAS PubMed;
(k) Y.-L. Liu, X. Wang, Y.-L. Zhao, F. Zhu, X.-P. Zeng, L. Chen, C.-H. Wang, X.-L. Zhao and J. Zhou, Angew. Chem., Int. Ed., 2013, 52, 13735 CrossRef CAS PubMed.
- For selected examples and reviews on the synthesis of spirocyclohexane oxindoles, see:
(a) C. Marti and E. M. Carreira, Eur. J. Org. Chem., 2003, 2209 CrossRef CAS;
(b) B. M. Trost and M. K. Brennan, Synthesis, 2009, 3003 CrossRef CAS;
(c) F. Zhou, Y.-L. Liu and J. Zhou, Adv. Synth. Catal., 2010, 352, 1381 CrossRef CAS;
(d) J. J. Badillo, N. V. Hanhan and A. K. Franz, Curr. Opin. Drug Discovery Dev., 2010, 13, 758 CAS;
(e) R. Dalpozzo, G. Bartoli and G. Bencivenni, Chem. Soc. Rev., 2012, 41, 7247 RSC;
(f) N. R. Ball-Jones, J. J. Badillo and A. K. Franz, Org. Biomol. Chem., 2012, 10, 5165 RSC;
(g) L. Hong and R. Wang, Adv. Synth. Catal., 2013, 355, 1023 CrossRef CAS;
(h) Y. Liu, H. Wang and J. Wan, Asian J. Org. Chem., 2013, 2, 374 CrossRef CAS;
(i) G. Zhu, W. Sun, C. Wu, G. Li, L. Hong and R. Wang, Org. Lett., 2013, 15, 4988 CrossRef CAS PubMed;
(j) D. Cheng, Y. Ishihara, B. Tan and C. F. Barbas III, ACS Catal., 2014, 4, 743 CrossRef CAS;
(k) C. M. R. Volla, I. Atodiresei and M. Rueping, Chem. Rev., 2014, 114, 2390 CrossRef CAS PubMed.
- For selected examples on the cascade organocatalytic asymmetric synthesis of spirocyclohexane oxindoles, see:
(a) G. Bencivenni, L.-Y. Wu, A. Mazzanti, B. Giannichi, F. Pesciaioli, M.-P. Song, G. Bartoli and P. Melchiorre, Angew. Chem., Int. Ed., 2009, 48, 7200 CrossRef CAS PubMed;
(b) K. Jiang, Z.-J. Jia, S. Chen, L. Wu and Y.-C. Chen, Chem. – Eur. J., 2010, 16, 2852 CrossRef CAS PubMed;
(c) K. Jiang, Z.-J. Jia, X. Yin, L. Wu and Y.-C. Chen, Org. Lett., 2010, 12, 2766 CrossRef CAS PubMed;
(d) Y.-B. Lan, H. Zhao, Z.-M. Liu, G.-G. Liu, J.-C. Tao and X.-W. Wang, Org. Lett., 2011, 13, 4866 CrossRef CAS PubMed;
(e) K. S. Halskov, T. K. Johansen, R. L. Davis, M. Steurer, F. Jensen and K. A. Jørgensen, J. Am. Chem. Soc., 2012, 134, 12943 CrossRef CAS PubMed;
(f) X.-F. Huang, Z.-M. Liu, Z.-C. Geng, S.-Y. Zhang, Y. Wang and X.-W. Wang, Org. Biomol. Chem., 2012, 10, 8794 RSC;
(g) A. K. Ghosh and B. Zhou, Tetrahedron Lett., 2013, 54, 2311 CrossRef CAS PubMed;
(h) B. Zhou, Y. Yang, J. Shi, Z. Luo and Y. Li, J. Org. Chem., 2013, 78, 2897 CrossRef CAS PubMed;
(i) S. Roy, M. Amireddy and K. Chen, Tetrahedron, 2013, 69, 8751 CrossRef CAS PubMed.
- For selected D–A strategy involved examples for the asymmetric synthesis of spirocyclohexane oxindoles, see:
(a) Q. Wei and L.-Z. Gong, Org. Lett., 2010, 12, 1008 CrossRef CAS PubMed;
(b) G. Li, T. Liang, L. Wojtas and J. C. Antilla, Angew. Chem., Int. Ed., 2013, 52, 4628 CrossRef CAS PubMed;
(c) Q.-Q. Zhou, X. Yuan, Y.-C. Xiao, L. Dong and Y.-C. Chen, Tetrahedron, 2013, 69, 10369 CrossRef CAS PubMed;
(d) P.-Q. Chen, Y.-C. Xiao, C.-Z. Yue and Y.-C. Chen, Org. Chem. Front., 2014, 1, 490 RSC.
- For selected reviews or book on the synthesis of spirocyclohexane oxindoles via domino reactions, see:
(a) K. C. Nicolaou, D. J. Edmonds and P. G. Bulger, Angew. Chem., Int. Ed., 2006, 45, 7134 CrossRef CAS PubMed;
(b)
L. F. Tietze, G. Brasche and K. M. Gericke, Domino Reactions in Organic Synthesis, Wiley-VCH, Weinheim, 2006 Search PubMed;
(c) D. Enders, C. Grondal and M. R. M. Hűttl, Angew. Chem., Int. Ed., 2007, 46, 1570 CrossRef CAS PubMed;
(d) X. Yu and W. Wang, Org. Biomol. Chem., 2008, 6, 2037 RSC;
(e) A. Dondoni and A. Massi, Angew. Chem., Int. Ed., 2008, 47, 4638 CrossRef CAS PubMed;
(f) C. Grondal, M. Jeanty and D. Enders, Nat. Chem., 2010, 2, 167 CrossRef CAS PubMed;
(g) Ł. Albrecht, H. Jiang and K. A. Jørgensen, Angew. Chem., Int. Ed., 2011, 50, 8492 CrossRef PubMed;
(h) A. Moyano and R. Rios, Chem. Rev., 2011, 111, 4703 CrossRef CAS PubMed;
(i) S. B. Jones, B. Simmons, A. Mastracchio and D. W. C. MacMillan, Nature, 2011, 475, 183 CrossRef CAS PubMed;
(j) L.-Q. Lu, J.-R. Chen and W.-J. Xiao, Acc. Chem. Res., 2012, 45, 1278 CrossRef CAS PubMed;
(k) C. C. J. Loh and D. Enders, Chem. – Eur. J., 2012, 18, 10212 CrossRef CAS PubMed;
(l) A. Grossmann and D. Enders, Angew. Chem., Int. Ed., 2012, 51, 314 CrossRef CAS PubMed;
(m) H. Pellissier, Adv. Synth. Catal., 2012, 354, 237 CrossRef CAS;
(n) P. Chauhan and S. S. Chimni, Tetrahedron: Asymmetry, 2013, 24, 343 CrossRef CAS PubMed;
(o) I. Chatterjee, D. Bastida and P. Melchiorrea, Adv. Synth. Catal., 2013, 355, 3124 CrossRef CAS;
(p) Z. Du and Z. Shao, Chem. Soc. Rev., 2013, 42, 1337 RSC;
(q) H. Pellissier, Chem. Rev., 2013, 113, 442 CrossRef CAS PubMed;
(r) X. Zeng, Chem. Rev., 2013, 113, 6864 CrossRef CAS PubMed;
(s) X. Zeng, Q. Ni, G. Raabe and D. Enders, Angew. Chem., Int. Ed., 2013, 52, 2977 CrossRef CAS PubMed;
(t) P. Chauhan and D. Enders, Angew. Chem., Int. Ed., 2014, 53, 1485 CrossRef CAS PubMed.
-
(a) H. Lin, Y. Tan, X.-W. Sun and G.-Q. Lin, Org. Lett., 2012, 14, 3818 CrossRef CAS PubMed;
(b) H. Lin, Y. Tan, W.-J. Liu, Z.-C. Zhang, X.-W. Sun and G.-Q. Lin, Chem. Commun., 2013, 49, 4024 RSC;
(c) H. Lin, X.-W. Sun and G.-Q. Lin, Org. Lett., 2014, 16, 752 CrossRef CAS PubMed;
(d) Y. Tan, H.-L. Luan, H. Lin, X.-W. Sun, X.-D. Yang, H.-Q. Dong and G.-Q. Lin, Chem. Commun., 2014, 50, 10027 RSC;
(e) Y. Tan, Y.-J. Chen, H. Lin, H.-L. Luan, X.-W. Sun, X.-D. Yang and G.-Q. Lin, Chem. Commun., 2014, 50, 15913 RSC.
- For selected examples on Michael addition catalyzed by a bifunctional squaramide, see:
(a) J. P. Malerich, K. Hagihara and V. H. Rawal, J. Am. Chem. Soc., 2008, 130, 14416 CrossRef CAS PubMed;
(b) H. Y. Bae, S. J. Some, S. Oh, Y. S. Lee and C. E. Song, Chem. Commun., 2011, 47, 9621 RSC;
(c) S.-W. Duan, H.-H. Lu, F.-G. Zhang, J. Xuan, J.-R. Chen and W.-J. Xiao, Synthesis, 2011, 1847 CAS;
(d) Y.-F. Wang, R.-X. Chen, K. Wang, B.-B. Zhang, Z.-B. Lib and D.-Q. Xu, Green Chem., 2012, 14, 893 RSC;
(e) O. Baslé, W. Raimondi, M. M. S. Duque, D. Bonne, T. Constantieux and J. Rodriguez, Org. Lett., 2010, 12, 5246 CrossRef PubMed;
(f) W. Raimondi, M. M. S. Duque, S. Goudedranche, A. Quintard, T. Constantieux, X. Bugaut, D. Bonne and J. Rodriguez, Synthesis, 2013, 1659 CAS.
- P. Chauhan, S. Mahajan, C. C. J. Loh, G. Raabe and D. Enders, Org. Lett., 2014, 16, 2954 CrossRef CAS PubMed.
-
(a) A. Noole, K. Ilmarinen, I. Järving, M. Lopp and T. Kanger, J. Org. Chem., 2013, 78, 8117 CrossRef CAS PubMed;
(b) A. Quintavalla, F. Lanza, E. Montroni, M. Lombardo and C. Trombini, J. Org. Chem., 2013, 78, 12049 CrossRef CAS PubMed.
- CCDC 1007247 (for N-Boc-deprotected product of 4b).
- The proposed transition states for the explanation of stereochemistry outcome.
.
Footnote |
† Electronic supplementary information (ESI) available. CCDC 1007247. For ESI and crystallographic data in CIF or other electronic format see DOI: 10.1039/c4qo00299g |
|
This journal is © the Partner Organisations 2015 |
Click here to see how this site uses Cookies. View our privacy policy here.