DOI:
10.1039/C5QO00027K
(Research Article)
Org. Chem. Front., 2015,
2, 677-680
Oxidative cross S–H/S–H coupling: selective synthesis of unsymmetrical aryl tert-alkyl disulfanes†
Received
23rd January 2015
, Accepted 25th March 2015
First published on 25th March 2015
Abstract
Selective oxidative coupling between aryl and tert-alkyl mercaptans was achieved using tert-butyl hydroperoxide (TBHP) as the oxidant and N-iodosuccinimide (NIS) as a catalyst. This protocol may provide an efficient process to synthesize unsymmetrical aryl tert-alkyl disulfanes.
Unsymmetrical disulfanes are a pivotal structural feature of many functional molecules that exhibit biological activities.1 In this context, aryl alkyl disulfanes have undergone clinical evaluation for tumor treatment.1f,g Hence, the synthesis of unsymmetrical disulfanes is an important task in synthetic chemistry.2 Traditionally, nucleophilic substitution of S–H bonds with sulfenyl derivatives has been employed to achieve unsymmetrical S–S bond formation.3 In this case, a leaving group is required to realize unsymmetrical disulfane construction (Scheme 1, path 1). Although remarkable achievements have been made utilizing this type of reaction, problems have arisen in the wake of the development of green chemistry: the large leaving group leads to low atom-economy. Moreover, harsh conditions or highly active reagents are required to prepare the sulfenyl derivatives. Therefore, it is a crucial task to develop a greener pathway to construct unsymmetrical disulfanes.
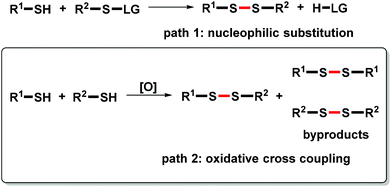 |
| Scheme 1 Two pathways leading to unsymmetrical disulfane construction. | |
Oxidative coupling between R1H and R2H is attracting more and more attention due to its high atom- and step-economy.4 Thus, this protocol is widely utilized to achieve the dimerization of mercaptans to afford symmetrical disulfanes.5 Because the difficulty of suppressing the homocoupling of S–H bonds can result in poor reaction selectivity (Scheme 1, path 2), there have been only isolated reports focusing on the oxidative cross coupling of two different S–H bonds.1h Although some primary and secondary alkyl mercaptans can react with aryl mercaptans to afford the corresponding unsymmetrical disulfanes, the oxidative coupling between aryl mercaptans and tert-alkyl mercaptans remains unexplored. Herein, we report a novel method to construct unsymmetrical aryl tert-alkyl disulfanes.
We initiated our study utilizing 2-mercaptobenzothiazole (1a) and tert-butyl mercaptan (2a) as substrates in a 1
:
1 ratio. They were selected since the corresponding unsymmetrical aryl alkyl disulfane 3aa could be a potential thioredoxin inhibitor.1e To our delight, the desired product was obtained in a yield of 35% in the presence of tert-butyl hydroperoxide (TBHP) as the oxidant (Scheme 2). Then, several iodine catalysts were examined for promoting the S–H/S–H coupling, because they can promote the homocoupling of mercaptans.6 After a series of tests to optimize the conditions, N-iodosuccinimide (NIS) and TBHP were selected as the catalyst and the oxidant, respectively (see ESI, Table S1†). The disulfane was obtained in a yield of 89% under the optimized conditions. The optimized conditions were: 1a (0.5 mmol), 2a (0.5 mmol), TBHP (0.55 mmol), NIS (0.05 mmol) and CH2Cl2 (1 mL) at 25 °C for 1 h under N2 (Scheme 3, 3aa).
 |
| Scheme 2 Oxidative coupling of 2-mercaptobenzothiazole with tert-butyl mercaptan. | |
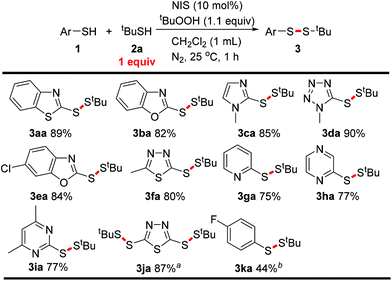 |
| Scheme 3 Cross coupling of tBuSH with aryl mercaptans. Reaction conditions: 1 (0.5 mmol), 2a (0.5 mmol), TBHP (0.55 mmol), NIS (0.05 mmol) and CH2Cl2 (1 mL) at 25 °C for 1 h under N2. Yields shown are isolated yields. a 3j (0.25 mmol). b The yield was determined by GC analysis with biphenyl as the internal standard and 19F NMR with trifluoromethylbenzene as the internal standard. | |
With the optimized conditions in hand, we then investigated the substrate scope of the reaction. First, various aryl mercaptans were tested in the reaction with tert-butyl mercaptan 1b under the optimized conditions. Mercapto N-containing heterocycles performed the S–S bond formation smoothly to give the corresponding aryl alkyl disulfanes. Mercapto 5-membered heterocycles, including benzothiazole, benzimidazole, benzoxazole, imidazole, tetrazole and 1,3,4-thiadiazole, exhibited good selectivity in the transformation. The yields ranged between 80% and 90% (Scheme 3, 3aa–3fa). Notably, the 1,3,4-thiadiazole bearing two mercapto groups also reacted with tBuSH with good selectivity, such that only the dithiolated product was obtained in 87% yield (Scheme 3, 3ja). It is noteworthy that mercapto N-containing 6-membered heterocycles were also suitable substrates for the reaction. Mercapto pyridine, pyrazine and pyrimidine were all tolerated, but their activities were relatively lower than the 5-membered heterocycles and the yields were around 75% (Scheme 3, 3ga–3ia). Unfortunately, when the aryl group was 4-fluorophenyl, only a 44% yield of the cross S–H/S–H coupling product was obtained (Scheme 3, 3ka). In this case, no selectivity was observed in the reaction between 4-fluorothiophenol 1h and 2a. This might result from their similar S–H bond activity.
Inspired by these encouraging results, we further investigated the reactivity of alkyl mercaptans other than tert-butyl mercaptan. 2-Mercapto-1-methylimidazole 1d was selected for the reaction with alkyl mercaptans. Secondary mercaptans were suitable for this transformation, but the yields were lowered to around 50–60% (Scheme 4, 3db, 3dc). Other tertiary mercaptans as well as tert-butyl mercaptan exhibited good reactivity. Cumene mercaptan 2d reacted with 1d to give the corresponding aryl alkyl disulfane 3dd with high selectivity (Scheme 4, 3dd). Triphenylmethyl mercaptan was also tolerated and an 80% yield of the product was obtained (Scheme 4, 3de). Moreover, tertiary mercaptans bearing a larger aliphatic group showed similar activity compared to tert-butyl mercaptan (Scheme 4, 3df, 3dg). However, unlike in previous reports,1h trace amounts of product were observed when primary mercaptans were reacted with 1d. This phenomenon may be ascribed to the faster dimerization of primary mercaptans.
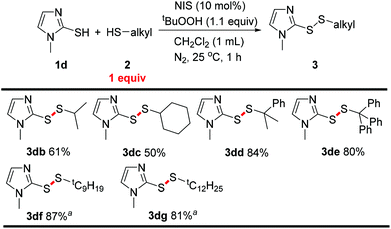 |
| Scheme 4 Cross coupling of 2-mercapto-1-methylimidazole 1d with alkyl mercaptans. Reaction conditions: 1d (0.5 mmol), 2 (0.5 mmol), TBHP (0.55 mmol), NIS (0.05 mmol) and CH2Cl2 (1 mL) at 25 °C for 1 h under N2. Yields shown are isolated yields. a Product was isolated as a mixture, since the starting materials tert-nonyl mercaptan 2f and tert-dodecyl mercaptan 2g were a mixture of isomers. | |
Furthermore, we attempted to gain some mechanistic insight into this selective cross S–H/S–H coupling. Several control experiments were firstly carried out. When tBuSH 2a was excluded from the reaction, 2-mercapto-benzothiazole 1a underwent dimerization smoothly to afford disulfane 4 (Scheme 5, eqn (1)). A similar result was observed when aryl mercaptan 1a was excluded from the reaction: the alkyl mercaptan 2a also performed the dimerization smoothly to give tert-butyl disulfane 5 (Scheme 5, eqn (2)). These results suggested that both aryl and alkyl mercapto radicals may form in the reaction. Moreover, a similar yield was obtained using dimer 4 as a replacement for monomer 1a (Scheme 5, eqn (3)). But the replacement of tert-butyl mercaptan 2a with its dimer 5 inhibited the reaction such that only a trace amount of the product was detected. (Scheme 5, eqn (4)). Therefore, we inferred that the dimer of the aryl mercaptan was the intermediate in this transformation, while the alkyl one was not. The dimer of the alkyl mercaptan was an inactive species in the reaction, thus its slow generation might be the key to achieving selectivity.
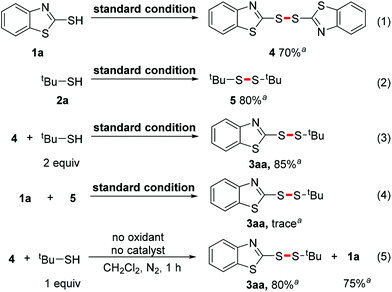 |
| Scheme 5 Control experiments. a The yield was determined by GC analysis with biphenyl as the internal standard. | |
Based on the literature precedent and experimental results, a plausible mechanism was put forward (Scheme 6). First, 2-mercapto-benzothiazole 1a undergoes oxidative dimerization with TBHP as the oxidant and NIS as the catalyst to give dimer 4 (Scheme 6, i). Next, there are two possible pathways. A nucleophilic attack may take place between tert-butyl mercaptan 2a and dimer 4 to produce the product 3aa with the regeneration of 1a. This process was confirmed by the reaction between 4 and 2a in a non-oxidizing environment (Scheme 5, eqn (5)).3l Another possibility leading to formation of the product is radical substitution of 4 by tert-butyl thiyl radical (Scheme 6, ii). A crucial side reaction is the oxidative dimerization of 2a, which leads to the futile consumption of alkyl mercaptan (Scheme 6, iii).
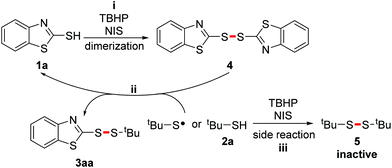 |
| Scheme 6 Plausible mechanism. | |
In summary, we have demonstrated a selective cross S–H/S–H coupling utilizing aryl and tert-alkyl mercaptans as substrates. Various aryl tert-alkyl disulfanes, which may exhibit bioactivity, were obtained in moderate to good yields using inexpensive NIS and TBHP. This protocol may provide an efficient way to synthesise unsymmetrical disulfanes, avoiding the need for S–H bond pre-activation and the low atom-economy caused by a large leaving group. Mechanistic studies revealed that the aryl disulfane was most likely to be the intermediate in the reaction, while the alkyl disulfane was inactive.
Acknowledgements
This work was supported by the 973 Program (2012CB725302, 2011CB808600), the National Natural Science Foundation of China (21390400, 21272180, and 21302148), the Research Fund for the Doctoral Program of Higher Education of China (20120141130002), the Program for Changjiang Scholars and Innovative Research Team in University (IRT1030) and the Ministry of Science and Technology of China (2012YQ120060). The Program of Introducing Talents of Discipline to Universities of China (111 Program) is also appreciated.
Notes and references
-
(a) Y. Mu, M. Nodwell, J. L. Pace, J.-P. Shaw and J. K. Judice, Bioorg. Med. Chem. Lett., 2004, 14, 735–738 CrossRef CAS PubMed;
(b) I. R. Vlahov, H. K. R. Santhapuram, P. J. Kleindl, S. J. Howard, K. M. Stanford and C. P. Leamon, Bioorg. Med. Chem. Lett., 2006, 16, 5093–5096 CrossRef CAS PubMed;
(c) V. M. Vrudhula, J. F. MacMaster, Z. Li, D. E. Kerr and P. D. Senter, Bioorg. Med. Chem. Lett., 2002, 12, 3591–3594 CrossRef CAS;
(d) I. R. Vlahov, H. K. R. Santhapuram, Y. Wang, P. J. Kleindl, F. You, S. J. Howard, E. Westrick, J. A. Reddy and C. P. Leamon, J. Org. Chem., 2007, 72, 5968–5972 CrossRef CAS PubMed;
(e) T. R. DiRaimondo, N. M. Plugis, X. Jin and C. Khosla, J. Med. Chem., 2013, 56, 1301–1310 CrossRef CAS PubMed;
(f) R. Ramanathan, J. Abbruzzese, T. Dragovich, L. Kirkpatrick, J. Guillen, A. Baker, L. Pestano, S. Green and D. Von Hoff, Cancer Chemother. Pharmacol., 2011, 67, 503–509 CrossRef CAS PubMed;
(g) R. K. Ramanathan, D. L. Kirkpatrick, C. P. Belani, D. Friedland, S. B. Green, H. H. Chow, C. A. Cordova, S. P. Stratton, E. R. Sharlow, A. Baker and T. Dragovich, Clin. Cancer Res., 2007, 13, 2109–2114 CrossRef CAS PubMed;
(h) J. K. Vandavasi, W.-P. Hu, C.-Y. Chen and J.-J. Wang, Tetrahedron, 2011, 67, 8895–8901 CrossRef CAS PubMed.
-
(a) B. Mandal and B. Basu, RSC Adv., 2014, 4, 13854–13881 RSC;
(b) D. Witt, Synthesis, 2008, 2491–2509 CrossRef CAS PubMed;
(c) D. Witt, R. Klajn, P. Barski and B. A. Grzybowski, Curr. Org. Chem., 2004, 8, 1763–1797 CrossRef CAS;
(d)
I. Shcherbakova and A. F. Pozharskii, in Comprehensive Organic Functional Group Transformations II, ed. A. R. Katritzky, R. Taylor and Ch. Ramsden, Pergamon, Oxford, 2004, vol. 2, pp. 210–233 Search PubMed;
(e)
P. C. Bulman Page, R. D. Wilkes and D. Reynolds, in Comprehensive Organic Functional Group Transformations, ed. A. R. Katritzky, O. Meth-Cohn and C. W. Rees, Pergamon, Oxford, 1995, vol. 2, pp. 177–187 Search PubMed;
(f)
R. Sato and T. Kimura, in Science of Synthesis, ed. N. Kambe, J. Drabowicz and G. A. Molander, Thieme, Stuttgart – New York, 2007, vol. 39, pp. 573–588 Search PubMed.
-
(a) M. Szymelfejnik, S. Demkowicz, J. Rachon and D. Witt, Synthesis, 2007, 3528–3534 CAS;
(b) M. Szymelfejnik, S. Demkowicz, J. Rachon and D. Witt, Synthesis, 2007, 3528–3534 CAS;
(c) S. Antoniow and D. Witt, Synthesis, 2007, 363–366 CAS;
(d) R. Hunter, M. Caira and N. Stellenboom, J. Org. Chem., 2006, 71, 8268–8271 CrossRef CAS PubMed;
(e) K. Tanaka and K. Ajiki, Tetrahedron Lett., 2004, 45, 5677–5679 CrossRef CAS PubMed;
(f) M. Bao and M. Shimizu, Tetrahedron, 2003, 59, 9655–9659 CrossRef CAS PubMed;
(g) M. Arisawa and M. Yamaguchi, J. Am. Chem. Soc., 2003, 125, 6624–6625 CrossRef CAS PubMed;
(h) J. Klose, C. B. Reese and Q. Song, Tetrahedron, 1997, 53, 14411–14416 CrossRef CAS;
(i) C. Brown and G. R. Evans, Tetrahedron Lett., 1996, 37, 9101–9104 CrossRef CAS;
(j) C. Leriverend and P. Metzner, Synthesis, 1994, 761–762 CrossRef CAS;
(k) P. Hiver, A. Dicko and D. Paquer, Tetrahedron Lett., 1994, 35, 9569–9572 CrossRef CAS;
(l) E. Brzezinska and A. L. Ternay, J. Org. Chem., 1994, 59, 8239–8244 CrossRef CAS;
(m) M. Ohtani and M. Narisada, J. Org. Chem., 1991, 56, 5475–5478 CrossRef CAS;
(n) D. H. R. Barton, R. H. Hesse, A. C. O'Sullivan and M. M. Pechet, J. Org. Chem., 1991, 56, 6697–6702 CrossRef CAS;
(o) D. H. R. Barton, C. Chen and G. Michael Wall, Tetrahedron, 1991, 47, 6127–6138 CrossRef CAS;
(p) D. P. Gamblin, P. Garnier, S. van Kasteren, N. J. Oldham, A. J. Fairbanks and B. G. Davis, Angew. Chem., Int. Ed., 2004, 43, 828–833 CrossRef CAS PubMed.
-
(a) L. Zhou and W. Lu, Chem. – Eur. J., 2014, 20, 634–642 CrossRef CAS PubMed;
(b) C. Liu, D. Liu and A. Lei, Acc. Chem. Res., 2014, 47, 3459–3470 CrossRef CAS PubMed;
(c) C. Zhang, C. Tang and N. Jiao, Chem. Soc. Rev., 2012, 41, 3464–3484 RSC;
(d) A. E. Wendlandt, A. M. Suess and S. S. Stahl, Angew. Chem., Int. Ed., 2011, 50, 11062–11087 CrossRef CAS PubMed;
(e) C. Liu, H. Zhang, W. Shi and A. Lei, Chem. Rev., 2011, 111, 1780–1824 CrossRef CAS PubMed;
(f) Z. Shao and F. Peng, Angew. Chem., Int. Ed., 2010, 49, 9566–9568 CrossRef CAS PubMed;
(g) J. Feng, M. F. Lv, G. P. Lu and C. Cai, Org. Chem. Front., 2015, 2, 60–64 RSC;
(h) L. Zhou, S. Tang, X. Qi, C. Lin, K. Liu, C. Liu, Y. Lan and A. Lei, Org. Lett., 2014, 16, 3404–3407 CrossRef CAS PubMed;
(i) C. Zhang and N. Jiao, Org. Chem. Front., 2014, 1, 109–112 RSC;
(j) S. Tang, X. Wu, W. Liao, K. Liu, C. Liu, S. Luo and A. Lei, Org. Lett., 2014, 16, 3584–3587 CrossRef CAS PubMed.
-
(a) X.-B. Li, Z.-J. Li, Y.-J. Gao, Q.-Y. Meng, S. Yu, R. G. Weiss, C.-H. Tung and L.-Z. Wu, Angew. Chem., Int. Ed., 2014, 53, 2085–2089 CrossRef CAS PubMed;
(b) A. Dhakshinamoorthy, S. Navalon, D. Sempere, M. Alvaro and H. Garcia, ChemCatChem, 2013, 5, 241–246 CrossRef CAS PubMed;
(c) R. Kumar, N. Sharma, U. K. Sharma, A. Shard and A. K. Sinha, Adv. Synth. Catal., 2012, 354, 2107–2112 CrossRef CAS PubMed;
(d) A. Corma, T. Rodenas and M. J. Sabater, Chem. Sci., 2012, 3, 398–404 RSC;
(e) D. R. Dreyer, H.-P. Jia, A. D. Todd, J. Geng and C. W. Bielawski, Org. Biomol. Chem., 2011, 9, 7292–7295 RSC;
(f) A. Dhakshinamoorthy, M. Alvaro and H. Garcia, Chem. Commun., 2010, 46, 6476–6478 RSC;
(g) J. L. Garcia Ruano, A. Parra and J. Aleman, Green Chem., 2008, 10, 706–711 RSC;
(h) R. Priefer, Y. J. Lee, F. Barrios, J. H. Wosnick, A.-M. Lebuis, P. G. Farrell, D. N. Harpp, A. Sun, S. Wu and J. P. Snyder, J. Am. Chem. Soc., 2002, 124, 5626–5627 CrossRef CAS PubMed;
(i) J. B. Arterburn, M. C. Perry, S. L. Nelson, B. R. Dible and M. S. Holguin, J. Am. Chem. Soc., 1997, 119, 9309–9310 CrossRef CAS.
-
(a) J. Yuan, X. Ma, H. Yi, C. Liu and A. Lei, Chem. Commun., 2014, 50, 14386–14389 RSC;
(b) M. Kirihara, Y. Asai, S. Ogawa, T. Noguchi, A. Hatano and Y. Hirai, Synthesis, 2007, 3286–3289 CrossRef CAS PubMed.
Footnote |
† Electronic supplementary information (ESI) available. See DOI: 10.1039/c5qo00027k |
|
This journal is © the Partner Organisations 2015 |
Click here to see how this site uses Cookies. View our privacy policy here.