DOI:
10.1039/C5QO00069F
(Research Article)
Org. Chem. Front., 2015,
2, 586-589
Enantioselective synthesis of trifluoromethyl substituted piperidines with multiple stereogenic centers via hydrogenation of pyridinium hydrochlorides†
Received
3rd March 2015
, Accepted 31st March 2015
First published on 31st March 2015
Abstract
An enantioselective iridium-catalyzed hydrogenation of trifluoromethyl substituted pyridinium hydrochlorides is described. Introduction of a trifluoromethyl group increases the reactivity due to the electron-withdrawing effect. Three stereogenic centers could be generated in one operation. This methodology provides a convenient route to chiral poly-substituted piperidines with up to 90% ee.
Chiral piperidines are valuable and prevalent substructures in biologically active natural products, synthetic bioactive compounds and medicines.1 In particular, the introduction of novel substituents on these framed syntheses of multiple stereocenter piperidines has been the focus of many chemists.2 Among them, selective introduction of trifluoromethyl groups can greatly modify the biological properties of the target molecules which are broadly present in several important drugs, such as JAK inhibitors (Fig. 1).3 Although organofluorine chemists have made tireless efforts, stereoselective synthesis of trifluoromethyl piperidines with multiple stereogenic centers is still an area which has been rarely explored to date.4
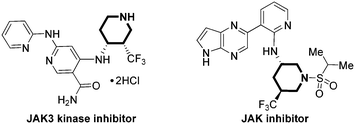 |
| Fig. 1 Selected biologically active molecules containing the trifluoromethylpiperidine motif. | |
Piperidines with multiple stereogenic centers are of great significance; together with our ongoing efforts in the development of asymmetric hydrogenation of N-heteroaromatics, we envision that asymmetric hydrogenation of such poly-substituted trifluoromethyl pyridines would provide straightforward access to these compounds. However, due to the stabilizing aromaticity5 and strong coordination ability of pyridines and the corresponding products, which might poison catalysts, in the past 15 years only a few homogeneous Rh and Ir catalysts6 and organocatalysts7 have been applied to synthesize chiral piperidines through asymmetric hydrogenation of special pyridines bearing strong electron-withdrawing groups or pyridinium salts (eqn (1) and (2)). Notably, very recently, Mashima and co-workers reported an iridium-catalyzed asymmetric hydrogenation of pyridinium salts,6i giving the chiral piperidines with two or three stereogenic centers in 28–82% ee and moderate yields (eqn (3)). Herein, we report an efficient asymmetric hydrogenation of poly-substituted pyridinium salts with excellent enantio- and diastereoselectivity (eqn (4)). Notably, introduction of the trifluoromethyl group increases the reactivity due to the electron-withdrawing effect. Three stereogenic centers could be generated in one operation.
On the basis that the extraneous Brønsted acid could activate substrates and accelerate iminium/enamine isomerization to facilitate hydrogenation,
8 we tried asymmetric hydrogenation of pyridinium hydrochloride. To our delight, 6-methyl-2-phenyl-3-trifluoromethylpyridinium hydrochloride (
1a·HCl) could be hydrogenated in full conversion with 67% ee and excellent diastereoselectivity (
Table 1, entry 1). Subsequently, different solvents were examined (entries 2–7) and the mixture solvents of dichloromethane (DCM) and isopropanol with a ratio of 3/1 gave the best result in terms of both enantioselectivity and conversion (85% ee and >95% conversion; entry 6). Sequentially, various halogen source additives (TCCA: trichloroisocyanuric acid, DCDMH: 1,3-dichloro-5,5-dimethylhydantoin and DBDMH: 1,3-dibromo-5,5-dimethylhydantoin) were tested, and gave similar ee values between 81 and 85% (entries 8–10). Some commercially available chiral bisphosphine ligands were also evaluated (entries 11–13), and the best result was achieved with (
R)-DifluorPhos
L3 (88% ee and >95% conversion; entry 12). Finally, 90% ee was achieved when the temperature was decreased to 25 °C, but the conversion reduced to 85%. Gratifyingly, full conversion with an identical enantioselectivity was obtained (entry 15, 90% ee) when the hydrogen pressure was raised to 800 psi with 2.5 mol% catalyst. Thus, the optimized conditions were established as: [Ir(COD)Cl]
2/(
R)-DifluorPhos/TCCA/(DCM/i-PrOH)/H
2 (800 psi)/25 °C.
Table 1 The evaluation of reaction parametersa

|
Entry |
Solvent |
Additive |
L
|
Conv.b (%) |
eec (%) |
Reaction conditions: 1a·HCl (0.125 mmol), [Ir(COD)Cl]2 (2.0 mol%), ligand (4.4 mol%), H2 (600 psi), solvent (3.0 mL), additive (10 mol%), 36 h, 50 °C.
Reaction conversion and dr were determined by 1H NMR spectroscopy. In all cases, dr >20 : 1.
Determined by HPLC analysis of the corresponding N-benzoyl derivatives.
25 °C.
[Ir(COD)Cl]2 (2.5 mol%), (R)-DifluorPhos (5.5 mol%), H2 (800 psi), 25 °C.
|
1 |
THF |
TCCA |
L1
|
>95 |
67 |
2 |
DCM (D) |
TCCA |
L1
|
91 |
82 |
3 |
Benzene |
TCCA |
L1
|
89 |
79 |
4 |
i-PrOH(P) |
TCCA |
L1
|
97 |
79 |
5 |
D/P (1 : 1) |
TCCA |
L1
|
>95 |
82 |
6 |
D/P (3 : 1) |
TCCA |
L1
|
>95 |
85 |
7 |
D/P (4 : 1) |
TCCA |
L1
|
>95 |
83 |
8 |
D/P (3 : 1) |
DCDMH |
L1
|
>95 |
83 |
9 |
D/P (3 : 1) |
DBDMH |
L1
|
>95 |
81 |
10 |
D/P (3 : 1) |
NCS |
L1
|
>95 |
82 |
11 |
D/P (3 : 1) |
TCCA |
L2
|
96 |
78 |
12 |
D/P (3 : 1) |
TCCA |
L3
|
>95 |
88 |
13 |
D/P (3 : 1) |
TCCA |
L4
|
>95 |
79 |
14d |
D/P (3 : 1) |
TCCA |
L3
|
85 |
90 |
15e |
D/P (3 : 1) |
TCCA |
L3
|
>95 |
90 |
|
With the optimized reaction conditions in hand, exploration of the substrate scope was carried out (Table 2). As expected, various substrates performed very well under standard reaction conditions. The electronic properties and position of substituents on the aromatic ring had a marginal effect on the reactivity and enantioselectivity (entries 1–8). Subsequently, the 6-ethyl-2-phenyl-3-(trifluoromethyl)pyridinium hydrochloride (1i·HCl) was also tested, 87% ee and 82% yield were obtained (entry 9). The absolute configuration of hydrogenation product 2f was assigned to be cis-(2R,3S,6R) based on single crystal X-ray diffraction analysis (Fig. 2).9
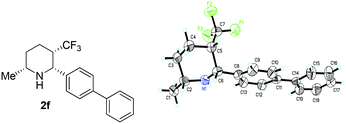 |
| Fig. 2 X-ray crystal structure of compound 2f. | |
Table 2 Asymmetric hydrogenation of 3-(trifluoromethyl)pyridinium hydrochloride (1·HCl)a
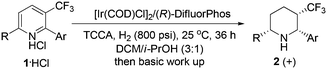
|
Entry |
R/Ar |
Yieldb (%) |
eec (%) |
Reaction conditions: 1·HCl (0.125 mmol), (R)-DifluorPhos (5.5 mol%), [Ir(COD)Cl]2 (2.5 mol%), H2 (800 psi), DCM–i-PrOH (3 : 1, 3.0 mL), TCCA (10 mol%), 36 h, 25 °C.
Isolated yields and in all cases dr >20 : 1.
Determined by HPLC analysis of the corresponding benzamide.
The absolute configuration was determined by single crystal X-ray diffraction analysis of 2f.
|
1 |
Me/C6H5 |
95 (2a) |
90 |
2 |
Me/4-MeC6H4 |
84 (2b) |
89 |
3 |
Me/3-MeC6H4 |
84 (2c) |
88 |
4 |
Me/4-MeOC6H4 |
94 (2b) |
88 |
5 |
Me/2-Naphthyl |
93 (2e) |
89 |
6d |
Me/4-C6H5C6H4 |
90 (2f) |
87 (2R,3S,6R) |
7 |
Me/4-CF3C6H4 |
85 (2g) |
86 |
8 |
Me/3,5-F2C6H3 |
72 (2h) |
84 |
9 |
Et/C6H5 |
82 (2i) |
87 |
In order to further estimate the application possibility, we applied this attractive protocol to the hydrogenation of the simple 2,6-disubstituted pyridinium hydrochloride. Gratifyingly, the reaction proceeded with moderate enantioselectivity and moderate to good reactivity (Scheme 1). In contrast to the asymmetric reduction of 3-(trifluoromethyl)pyridinium hydrochloride 1, in these cases the reactions were carried out under relatively harsh conditions (1200 psi hydrogen pressure and 80 °C). The reactivity discrepancy of these two types of substrates might be ascribed to the electron-withdrawing ability of the trifluoromethyl group that activates pyridine to facilitate hydrogenation.
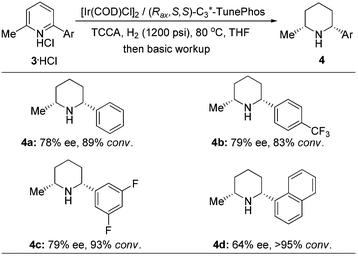 |
| Scheme 1 Asymmetric hydrogenation of 2,6-disubstituted pyridinium hydrochloride (3·HCl). Reaction conditions: 3·HCl (0.125 mmol), (Rax,S,S)-C3*-TunePhos (2.2 mol%), [Ir(COD)Cl]2 (1.0 mol%), H2 (1200 psi), THF (3.0 mL), TCCA (10 mol%), 24 h, 80 °C. Reaction conversion and dr were determined by 1H NMR spectroscopy. In all cases, dr >20 : 1. | |
In conclusion, an efficient and direct approach to chiral trifluoromethyl substituted piperidines with multiple stereogenic centers has been successfully developed via iridium-catalyzed asymmetric hydrogenation of the corresponding pyridinium hydrochlorides with up to 90% ee. Three stereogenic centers could be generated in one operation. Introduction of the trifluoromethyl group increases the reactivity of pyridine hydrogenation due to the strong electron-withdrawing effect. Meanwhile, this attractive protocol can also be applied to the asymmetric hydrogenation of the simple 2,6-disubstituted pyridinium hydrochlorides with moderate reactivity and enantioselectivity. Further investigations on asymmetric hydrogenation of poly-substituted heteroaromatics are currently ongoing in our laboratory.
Experimental section
Typical procedure for asymmetric hydrogenation of 1a
In a nitrogen-filled glove box, a mixture of [Ir(COD)Cl]2 (2.1 mg, 0.0031 mmol) and (R)-DifluorPhos (4.7 mg, 0.0069 mmol) in dichloromethane–isopropanol (3
:
1, 1.0 mL) was stirred at room temperature for 15–20 min, using a syringe the mixture was transferred to a stainless steel autoclave, in which the substrate 1a·HCl (34.0 mg, 0.20 mmol) and TCCA (2.9 mg, 0.0125 mmol) had been placed beforehand. Then, dichloromethane–isopropanol (3
:
1, 2.0 mL) was added. The hydrogenation was performed at 25 °C under 800 psi hydrogen pressure for 36 h. After carefully releasing the hydrogen, triethylamine (56 μL, 0.40 mmol) was added and the mixture was stirred for 30 min. The organic layer was separated and extracted with dichloromethane twice, and the combined organic extracts were dried over sodium sulfate and concentrated in vacuo. The resulting residue was purified by silica gel column chromatography using petroleum ether–ethyl acetate to give the desired product 2a as pale oil (29 mg, 95% yield). Enantiomeric excess was determined by HPLC for the corresponding benzamide (OJ–H, elute: hexanes–i-PrOH = 90/10, detector: 220 nm, flow rate: 1.0 mL min−1), 30 °C, t1 = 10.6 min (maj), t2 = 15.3 min (90% ee).
Acknowledgements
We are grateful for financial support from the National Natural Science Foundation of China (21125208 and 21372220).
Notes and references
-
(a)
M. Rubiralta, E. Giralt and A. Diez, Piperidine: Structure, Preparation and Synthetic Applications of Piperidine and its Derivatives, Elsevier, Amsterdam, 1991 Search PubMed. For a recent review, see:
(b) D. M. Stout and A. I. Meyers, Chem. Rev., 1982, 82, 223 CrossRef CAS;
(c) P. D. Bailey, P. A. Millwood and P. D. Smith, Chem. Commun., 1998, 633 RSC;
(d) H. P. Husson and J. Royer, Chem. Soc. Rev., 1999, 28, 383 RSC;
(e) A. Mitchenson and A. Nadin, J. Chem. Soc., Perkin Trans. 1, 2000, 2862 RSC;
(f) S. Laschat and T. Dickner, Synthesis, 2000, 1781 CrossRef CAS PubMed;
(g) F.-X. Felpin and J. Lebreton, Eur. J. Org. Chem., 2003, 3693 CrossRef CAS PubMed;
(h) P. Weintraub, Tetrahedron, 2003, 59, 2953 CrossRef CAS;
(i) M. G. P. Buffat, Tetrahedron, 2004, 60, 1701 CrossRef CAS PubMed;
(j) J. P. A. Harrity and O. Provoost, Org. Biomol. Chem., 2005, 3, 1349 RSC;
(k) C. Escolano, M. Amat and J. Bosch, Chem. – Eur. J., 2006, 12, 8198 CrossRef CAS PubMed;
(l) M. Ahamed and M. H. Todd, Eur. J. Org. Chem., 2010, 5935 CrossRef CAS PubMed.
- For a recent review, see:
(a) P. M. Weintraub, J. S. Sabol, J. M. Kane and D. R. Borcherding, Tetrahedron, 2003, 59, 2953 CrossRef CAS. For selected examples of biologically active molecules containing the fluoropiperidine motif, see:
(b) R. Surmont, G. Verniest, J. W. Thuring, P. ten Holte, F. Deroose and N. De Kimpe, Org. Biomol. Chem., 2010, 8, 4514 RSC;
(c) A. Orliac, J. Routier, F. B. Charvillon, W. H. B. Sauer, A. Bombrun, S. S. Kulkarini, D. G. Pardo and J. Cossy, Chem. – Eur. J., 2014, 20, 3813 CrossRef CAS PubMed;
(d) Z. Yuan, H.-Y. Wang, X. W.-P. Chen, Y.-L. Guo and G. Liu, J. Am. Chem. Soc., 2015, 137, 2468 CrossRef CAS PubMed.
-
(a) P. S. Watson, B. Jiang and B. Scott, Org. Lett., 2000, 2, 3679 CrossRef CAS PubMed;
(b) M. G. P. Buffat, Tetrahedron, 2004, 60, 1701 CrossRef CAS PubMed;
(c) M. S. M. Pearson, M. Mathé-Allainmat, V. Fargeas and J. Lebreton, Eur. J. Org. Chem., 2005, 2159 CrossRef CAS PubMed;
(d) S. Escolano, M. Amat and J. Bosch, Chem. – Eur. J., 2006, 12, 8199 CrossRef PubMed;
(e) S. Källström and R. Leino, Bioorg. Med. Chem., 2008, 16, 601 CrossRef PubMed;
(f) K. Muller, C. Faeh and F. Diederich, Science, 2007, 317, 1881 CrossRef PubMed;
(g) W. K. Hagmann, J. Med. Chem., 2008, 51, 4359 CrossRef CAS PubMed;
(h) D. O'Hagan, Chem. Soc. Rev., 2008, 37, 308 RSC;
(i) S. Purser, P. R. Moore, S. Swallow and V. Gouverneur, Chem. Soc. Rev., 2008, 37, 320 RSC;
(j)
V. A. Petrov, Fluorinated Heterocyclic Compounds: Synthesis, Chemistry, and Applications, John Wiley and Sons, Hoboken, New Jersey, 2009 Search PubMed;
(k) T. Wu, G. Yin and G. Liu, J. Am. Chem. Soc., 2009, 131, 16354 CrossRef CAS PubMed;
(l)
J. de Vicente Fidalgo, J. C. Hermann, R. Lemoine, H. Li, J. A. Lover, E. B. Sjogren and M. Soth, US, 20110059118 A1, 2011 Search PubMed;
(m)
S. Shirkami, F. Takahashi, Y. Nakajima, H. Omura, N. Aoyama, H. Sasaki, T. Hiroshi and H. Tominaga, WO, 2010058846 A1, 2010 Search PubMed.
- For examples of synthesis of chiral piperidines bearing trifluoromethyl, see:
(a) F. Glorius, N. Spielkamp, S. Holle, R. Goddard and C. W. Lehmann, Angew. Chem., Int. Ed., 2004, 43, 2850 CrossRef CAS PubMed;
(b) G. Magueur, J. Legros, F. Meyer, M. Ouréitch, B. Crousse and D. Bonnet-Delpon, Eur. J. Org. Chem., 2005, 1258 CrossRef CAS PubMed;
(c) G. Kim and N. Kim, Tetrahedron Lett., 2005, 46, 423 CrossRef CAS PubMed;
(d) S. Fustero, S. Monteagudo, M. Sánchez-Roselló, S. Flores, P. Barrio and C. del Pozo, Chem. – Eur. J., 2010, 16, 9835 CrossRef CAS PubMed;
(e) S. Fustero, L. Albert, N. Mateu, G. Chiva, J. Miro, J. Gonzalez and J. L. Aceña, Chem. – Eur. J., 2012, 18, 3753 CrossRef CAS PubMed;
(f) W.-B. Jatoi, A. Desiront, A. Job, Y. Troin and J.-L. Canet, J. Fluorine Chem., 2013, 145, 8 CrossRef CAS PubMed;
(g) R.-N. Guo, Z.-P. Chen, X.-F. Cai and Y.-G. Zhou, Synthesis, 2014, 2751 CAS.
- For selected reviews on asymmetric hydrogenation of aromatics, see:
(a) Y.-G. Zhou, Acc. Chem. Res., 2007, 40, 1357 CrossRef CAS PubMed;
(b) D.-S. Wang, Q.-A. Chen, S.-M. Lu and Y.-G. Zhou, Chem. Rev., 2012, 112, 2557 CrossRef CAS PubMed;
(c) S.-M. Lu, X.-W. Han and Y.-G. Zhou, Chin. J. Org. Chem., 2005, 25, 634 CAS;
(d) F. Glorius, Org. Biomol. Chem., 2005, 3, 4171 RSC. For recent examples see:
(e) G.-Y. Chen, S.-M. Lu and Y.-G. Zhou, Chin. J. Org. Chem., 2006, 11, 1548 Search PubMed;
(f) T. Wang, L.-G. Zhou, Z. Li, F. Chen, Z. Ding, Y. He, Q.-H. Fan, J. Xiang, Z.-X. Yu and A. S. C. Chan, J. Am. Chem. Soc., 2011, 133, 9878 CrossRef CAS PubMed;
(g) S. Urban, B. Beiring, N. Ortega, D. Paul and F. Glorius, J. Am. Chem. Soc., 2012, 134, 15241 CrossRef CAS PubMed;
(h) D. Cartigny, F. Berhal, T. Nagano, P. Phansavath, T. Ayad, J.-P. Genêt, T. Ohshima, K. Mashima and V. Ratovelomanana-Vidal, J. Org. Chem., 2012, 77, 4544 CrossRef CAS PubMed;
(i) T. Wang, F. Chen, J. Qin, Y.-M. He and Q.-H. Fan, Angew. Chem., Int. Ed., 2013, 52, 7172 CrossRef CAS PubMed;
(j) J. Wysocki, N. Ortega and F. Glorius, Angew. Chem., Int. Ed., 2014, 53, 8751 CrossRef CAS PubMed.
- For metal-catalyzed asymmetric hydrogenation of pyridines, see:
(a) M. Studer, C. Wedemeyer-Exl, F. Spindler and H. U. Blaser, Monatsh. Chem., 2000, 131, 1335 CrossRef CAS;
(b) C. Y. Legault and A. B. Charette, J. Am. Chem. Soc., 2005, 127, 8966 CrossRef CAS PubMed;
(c) C. Y. Legault, A. B. Charette and P. G. Cozzi, Hetereocycles, 2008, 76, 1271 CrossRef CAS PubMed;
(d) X.-B. Wang, W. Zeng and Y.-G. Zhou, Tetrahedron Lett., 2008, 49, 4922 CrossRef CAS PubMed;
(e) W. Tang, Y. Sun, L. Xu, T. Wang, Q.-H. Fan, K.-H. Lam and A. S. C. Chan, Org. Biomol. Chem., 2010, 8, 3464 RSC;
(f) W.-J. Tang, J. Tan, L.-J. Xu, K.-H. Lam, Q.-H. Fan and A. S. C. Chan, Adv. Synth. Catal., 2010, 352, 1055 CrossRef CAS PubMed;
(g) Z.-S. Ye, M.-W. Chen, Q.-A. Chen, L. Shi, Y. Duan and Y.-G. Zhou, Angew. Chem., Int. Ed., 2012, 51, 10181 CrossRef CAS PubMed;
(h) A. Cadu, P. Upadhyay and P. G. Andersson, Asian J. Org. Chem., 2013, 2, 1061 CrossRef CAS PubMed;
(i) Y. Kita, A. Iimuro, S. Hida and K. Mashima, Chem. Lett., 2014, 43, 284 CrossRef CAS;
(j) M. Chang, Y. Huang, S. Liu, Y. Chen, S. W. Krska, I. W. Davies and X. Zhang, Angew. Chem., Int. Ed., 2014, 53, 12761 CrossRef CAS PubMed.
- M. Rueping and A. P. Antonchick, Angew. Chem., Int. Ed., 2007, 46, 4562 CrossRef CAS PubMed.
- For selected reviews on the Brønsted acid effect in asymmetric hydrogenation by forming the corresponding iminium salts, see:
(a) Z.-W. Li, T.-L. Wang, Y.-M. He, Z.-J. Wang, Q.-H. Fan, J. Pan and L.-J. Xu, Org. Lett., 2008, 10, 5265 CrossRef CAS PubMed;
(b) H. Tadaoka, D. Cartigny, T. Nagano, T. Gosavi, T. Ayad, J.-P. Genêt, T. Ohshima, V. Ratovelomanana-Vidal and K. Mashima, Chem. – Eur. J., 2009, 15, 9990 CrossRef CAS PubMed;
(c) G. Hou, F. Gosselin, W. Li, J. C. McWilliams, Y. Sun, M. Weisel, P. D. O'Shea, C. Chen, I. W. Davies and X. Zhang, J. Am. Chem. Soc., 2009, 131, 9882 CrossRef CAS PubMed;
(d) G. Hou, W. Li, M. Ma, X. Zhang and X. Zhang, J. Am. Chem. Soc., 2010, 132, 12844 CrossRef CAS PubMed;
(e) G. Hou, R. Tao, Y. Sun, X. Zhang and F. Gosselin, J. Am. Chem. Soc., 2010, 132, 2124 CrossRef CAS PubMed;
(f) D.-S. Wang, Q.-A. Chen, W. Li, C.-B. Yu, Y.-G. Zhou and X. Zhang, J. Am. Chem. Soc., 2010, 132, 8909 CrossRef CAS PubMed;
(g) D.-S. Wang, J. Tang, Y.-G. Zhou, M.-W. Chen, C.-B. Yu, Y. Duan and G.-F. Jiang, Chem. Sci., 2011, 2, 803 RSC;
(h) D.-S. Wang, Z.-S. Ye, Q.-A. Chen, Y.-G. Zhou, C.-B. Yu, H.-J. Fan and Y. Duan, J. Am. Chem. Soc., 2011, 133, 8866 CrossRef CAS PubMed;
(i) Z. Yu, W. Jin and Q. Jiang, Angew. Chem., Int. Ed., 2012, 51, 6060 CrossRef CAS PubMed;
(j) A. Iimuro, K. Yamaji, S. Kandula, T. Nagano, Y. Kita and K. Mashima, Angew. Chem., Int. Ed., 2013, 52, 2046 CrossRef CAS PubMed;
(k) R.-N. Guo, X.-F. Cai, L. Shi, Z.-S. Ye, M.-W. Chen and Y.-G. Zhou, Chem. Commun., 2013, 49, 8537 RSC;
(l) Y. Duan, L. Li, M.-W. Chen, C.-B. Yu, H.-J. Fan and Y.-G. Zhou, J. Am. Chem. Soc., 2014, 136, 7688 CrossRef CAS PubMed.
- CCDC 1009006 contains the supplementary crystallographic data for this paper.
Footnote |
† Electronic supplementary information (ESI) available: Experimental details. CCDC 1009006. For ESI and crystallographic data in CIF or other electronic format see DOI: 10.1039/c5qo00069f |
|
This journal is © the Partner Organisations 2015 |
Click here to see how this site uses Cookies. View our privacy policy here.