DOI:
10.1039/C5QO00092K
(Review Article)
Org. Chem. Front., 2015,
2, 849-858
Catalytic asymmetric synthesis of polysubstituted spirocyclopropyl oxindoles: organocatalysis versus transition metal catalysis
Received
23rd March 2015
, Accepted 30th April 2015
First published on 5th May 2015
Abstract
Spirocyclopropyl oxindoles are widely present in natural products and bioactive molecules. In addition, they play a significant role as a type of donor–acceptor (DA) cyclopropanes in catalytic reactions. Four distinct pathways have been developed for the asymmetric synthesis of this framework, which nicely show the potential of organocatalytic and metal catalyzed asymmetric cyclopropanation in the creation of spirocyclic compounds. This minireview summarizes these complementary methods to synthesise optically active spirocyclopropyl oxindoles bearing various functional groups, discusses the difference and advantages of each method, and summarizes synthetic opportunities that still exist.
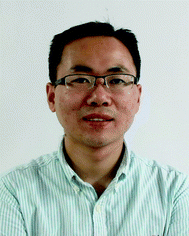 Zhong-Yan Cao | Zhong-Yan Cao was born in Bozhou, P. R. China, and received his B.Sc. from East China Normal University in 2010. Currently he is pursuing his PhD under the guidance of Prof. Jian Zhou at the same university. His research interests focus on the development of soft Lewis acids for constructing tetrasubstituted carbon centers. |
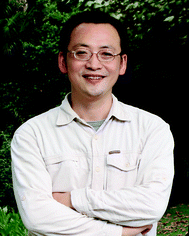 Jian Zhou | Jian Zhou obtained his PhD in 2004 from Shanghai Institute of Organic Chemistry, CAS, under the guidance of Prof. Yong Tang. After postdoctoral research with Professor Shū Kobayashi at the University of Tokyo and Professor Benjamin List at Max-Planck-Institut für Kohlenforschung, he joined East China Normal University as a Professor from the end of 2008. His research interests include the development of new chiral catalysts and asymmetric reactions for the construction of tetrasubstituted carbon stereocenters. He has received the “Thieme Chemistry Journal Award 2011”. He is now a member of the Advisory Board of Organic & Biomolecular Chemistry and Current Organocatalysis. |
1. Introduction
As modern probe- and drug-discovery programmes have met with diminishing returns from high-throughput screening of commercial compound collections, there is a vast demand for synthetic libraries derived from privileged scaffolds.1 In this context, spiro scaffolds have attracted considerable attention, as the conformational restriction imposed by incorporation of a spiro-ring fusion reduces the conformational entropy penalty upon interacting with a protein target.2 Therefore, efficient methods allowing for enantioselective construction of three-dimensional spirocyclic compounds in sufficient diversity are highly desirable.3 Over the past few years, there has been great interest in catalytic stereoselective syntheses of spirooxindoles, a privileged structural motif widely present in bioactive natural products, pharmaceutically active molecules and drugs.4 On the other hand, spirooxindoles are continuously being discovered as exciting leads and drug candidates, which further fuels this research.5 In particular, spirocyclopropyl oxindoles 1, with the smallest three-membered ring fused at the C3 position of oxindoles, is an attractive framework for both medicinal research and synthetic chemistry.
First, through spiro-ring fusion, spirocyclopropyl oxindoles integrate two important pharmacophores, quaternary oxindoles6 and cyclopropanes,7 both of which occur in many bioactive compounds displaying a wide spectrum of biological activities. As a consequence, conformation constrained spirocyclopropyl oxindole derivatives often showed remarkable bioactivities.5 For example, compound 2
5d–f showed nanomolar level activities as a HIV-1 non-nucleoside reverse transcriptase inhibitor; 3
5i and 4
5k were found to be a kinase inhibitor and an arginine vasopressin inhibitor, respectively, whereas compounds of formula 5
5h–j exhibited a distinct antitumor activity and also effectiveness in treating obesity and diabetes (Fig. 1).
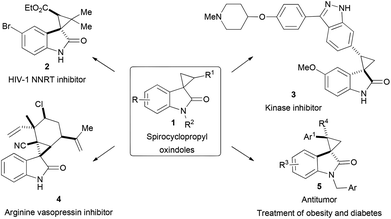 |
| Fig. 1 Representative bioactive molecules. | |
Second, spirocyclopropyl oxindoles can be viewed as a kind of donor–acceptor cyclopropanes for further elaboration.8 The group of Carreira has pioneered the catalytic [3 + 2] ring-expansion reaction of spirocyclopropyl oxindoles with imines to furnish spiro cyclopentane oxindoles (Scheme 1).9 This nice method has demonstrated its powerfulness in the total synthesis of natural products (±)-horsfiline 7
9e (eqn (1)) and spirotryprostatin B 8
9f (eqn (2)). Recently, we have found that the [3 + 2] annulation reaction of spirocyclopropyl oxindole 1c with benzaldehyde could also take place in the presence of 10 mol% Cu(OTf)2, to provide spiro[furan-3,3′-indolin]-2-one 9 without loss of ee values, albeit in a moderate 3
:
1 dr value (eqn (3)).10 It should be pointed out that, although the chemistry of DA cyclopropanes has been intensively studied by Kerr,11 Johnson,12 Tang13 and others,14 the potential of spirocyclopropyl oxindoles in the related reactions remains to be fully explored: not only is there a lack of any catalytic asymmetric version, but also the reaction and dipolarophile types are very limited.
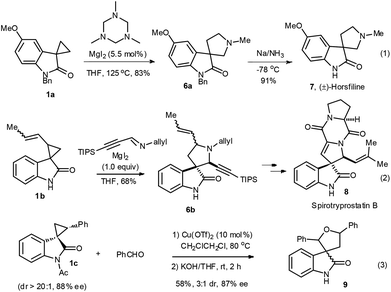 |
| Scheme 1 Synthetic application of spirocyclopropyl oxindoles. | |
Third, owing to the existence of the highly strained cyclopropane bearing a spiro quaternary carbon steocenter,3 catalytic enantioselective synthesis of such spiro compounds, in particular, those with three continuous carbon stereocenters, constitutes a very challenging task.15 This research provides an ideal testing ground for new synthetic methods and new chiral catalyst systems to demonstrate their power and potential.
In view of the importance of spirocyclopropyl oxindoles, much attention has been paid to developing efficient synthetic methods. Apart from a number of racemic methods that have been reported,16 including olefin cyclopropanation using diazooxindole,16a–d tandem Wittig-cyclopropanation from isatin and ylide,16e,f and reductive cyclopropanation,16g,h much progress has been made in catalytic asymmetric studies. To date, four distinct synthetic pathways, classified by the substrates used, have been exploited for the stereoselective synthesis of spirocyclopropyl oxindoles (Fig. 2). Organocatalytic methods allow N-Boc protected alkylideneoxindole 10, oxindole 11, and 3-chlorooxindole 12 to readily transform to the desired spirocyclic framework via the Michael addition-initiated cascade strategy (paths 1–3), whereas transition metal-catalyzed methods are based on diazooxindole 13 (path 4). These methods are complementary and orthogonal to each other in the facile access to spirocyclopropyl oxindoles bearing different functional groups or substituents. In addition, they provide a good platform for readers who just enter the field to understand the advantages and limitation of chiral metal catalysis and two organocatalytic catalysis patterns (bifunctional tertiary amine–H-bond donor catalysis and iminium catalysis) in the asymmetric synthesis of cyclopropanes. While there are three excellent reviews focusing on the catalytic asymmetric synthesis of spirocyclic oxindoles,4b–d they all just mentioned one or two examples of the synthesis of spirocyclopropyl oxindoles. With our continuous efforts in oxindole chemistry,17 we felt it necessary to make a comprehensive review to introduce the latest achievement in catalytic asymmetric synthesis of spirocyclopropyl oxindoles, discuss the advantages and limitation of each method, and summarize possible synthetic opportunities. This review is therefore divided into two parts: (i) organocatalytic protocols and (ii) transition metal-catalyzed olefin cyclopropanation.
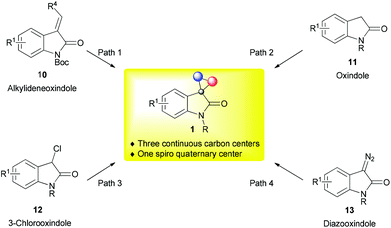 |
| Fig. 2 Four paths to spirocyclopropyl oxindoles. | |
2. Organocatalytic tandem Michael addition-intramolecular alkylation reactions
Organocatalytic asymmetric tandem Michael/intramolecular alkylation reaction is a powerful strategy for the synthesis of chiral cyclopropanes.18 By installing a suitable leaving group on the nucleophile or the electrophile, the intermediate enolate formed in situ via the initial Michael addition further undergoes an intramolecular alkylation to give the desired optically active cyclopropanes. Asymmetric iminium catalysis and Brønsted base catalysis are two viable strategies for reaction design. While iminium catalysis enables LUMO-lowering activation of α,β-unsaturated aldehydes or ketones via the formation of an iminium intermediate, Brønsted base catalysis allows HOMO-raising activation of nucleophiles. MacMillan et al. first applied iminium catalysis to cyclopropanation using sulfonium ylide as the nucleophile,19a and later Wang19b and Córdova19c reported that activated methylene compounds could be employed as well. Chiral Brønsted bases mediated version was first achieved by the group of Takemoto.19d Both organocatalytic methods have now been successfully applied to the stereoselective synthesis of spirocyclopropyl oxindoles, given that one of the reaction partners has a preformed oxindole ring structure, as exhibited by the following advances.
2.1 Path 1: using N-protected alkylideneoxindole
In 2011, Bencivenni and Bartoli pioneered the Michael-addition initiated cascade annulation strategy for asymmetric synthesis of spirocyclopropyl oxindoles. Under the catalysis of a cinchona alkaloid derived bifunctional thiourea catalyst 16a, the annulation of alkylideneoxindole 10 worked well with α-bromo nitroalkane 14 to give spiro nitrocyclopropane oxindoles 15 in excellent enantioselectivity and moderate to high dr value (Scheme 2).20 The pseudoenantiomeric 9-epi-9-thiourea-9-deoxydihydroquiniline catalyst 16b allowed the accessibility to opposite enantiomers of product 15. It was reported that the electron-withdrawing R group of Michael acceptor 10 contributed to the excellent dr value of the products, but alkyl and aryl R groups led to moderate diastereoselectivities. Interestingly, 1-bromo-1-nitroethane 14b was also viable under these conditions to furnish adjacent quaternary carbon stereocenters in excellent enantioselectivities, albeit with moderate diastereoselectivity. This process was possibly initiated by a Michael addition of 14 to 10, followed by an intramolecular alkylation. The authors proposed that the deprotonation of 14 by the quinuclidine moiety of the catalyst facilitated its addition to alkylideneoxindole 10, which was controlled by the thiourea part of the catalyst through H-bond interactions.
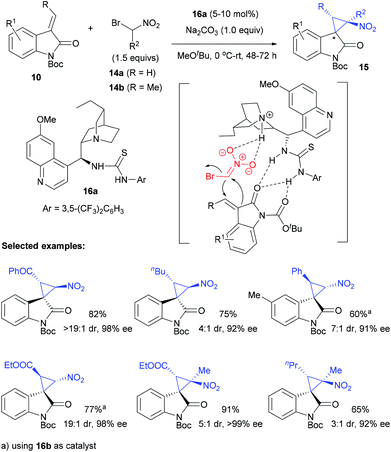 |
| Scheme 2 Asymmetric annulation with bromonitroalkanes. | |
The Michael-annulation strategy could be extended to other C1 synthons bearing similar nucleophilic/electrophilic dualistic properties. For example, the construction of two adjacent quaternary carbon centres within the same cyclopropane along with excellent dr and ee values was achieved by using α-chloro-β-dicarbonyl derivatives 17 (Scheme 3). Malkov et al. found that a similar bifunctional thiourea 16c catalysed the reaction of alkylideneoxindoles 10 and 17 well, to give the desired spirocyclopropanes 18 bearing useful ketone or ester groups in 62–97% yield, up to 99
:
1 dr and 80–98% ee.21 The reactivity of ethyl α-chloroacetoacetate 17a was virtually the same as that of 3-chloroacetylacetone 17b, while chloromalonate 17c proved to be poorest, as evidenced by a slow reaction rate.
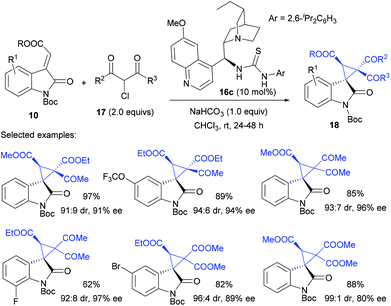 |
| Scheme 3 Annulation with α-chloro-β-dicarbonyl derivatives. | |
2.2 Path 2: using 3-chlorooxindole
Alternatively, the oxindole skeleton could be installed into the nucleophiles for reaction design. For example, 3-chlorooxindoles 12 with intrinsically dualistic properties have been exploited for the synthesis of spirocyclopropyl oxindoles. Kanger et al. reported the reaction of N-Boc 3-chlorooxindole 12 with enones 19 and were able to synthesize α,β-spirocyclopropyl oxindoles 20 (Scheme 4).22 It should be noted that no reaction took place for the N-unprotected 3-chlorooxindole, probably due to the low acidity of C–H at the C3 position. While both the desired trans-substituted products 20 with a nonstereogenic quaternary carbon and achiral diastereomeric cis-20 can be expected, the use of the bifunctional catalyst 16a along with 2.0 equiv. of NaHCO3 dominantly afforded trans-20 from N-Boc 3-chlorooxindole 12 and enones 19 in 58–81% yield, 8
:
1–20
:
1 dr and 71–87% ee. In the case of non-symmetric enones or keto esters, even if there were two different electrophilic centres, only one of four possible stereoisomers was formed, indicating the high regioselectivity for the first Michael addition step. However, in all cases, a small amount of uncyclized Michael adduct 21 was inevitably formed and aliphatic diketones did not proceed at all.
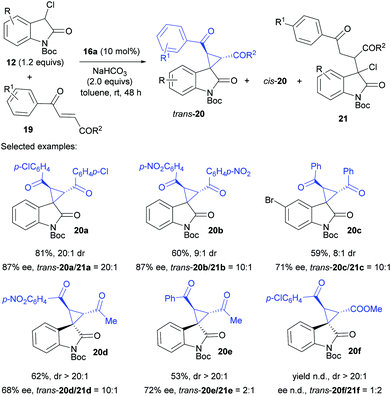 |
| Scheme 4 Annulation with 1,4-dicarbonyl derivatives. | |
While N-Boc 3-chlorooxindoles are highly reactive for the development of the reaction, the introduction and removal of the Boc group required additional procedures. To develop more atom-economical protocols using unprotected 3-chlorooxindoles, Lu utilized their own quinine derived multifunctional catalyst 16d to realize a highly stereoselective synthesis of N-unprotected spiro nitrocyclopropane oxindoles 23 in a 25
:
1 dr value and 86–99% ee (Scheme 5).23 The method was complementary to Bencivenni's work for synthesizing spiro nitrocyclopropanes.20 The initial asymmetric Michael addition of 3-chlorooxindole 12 to β-phenyl nitroolefin 22a catalyzed by 5 mol% of the catalyst 16d worked efficiently to realize excellent stereoselectivity, and the subsequent treatment with HCO2Na facilitated the formation of cyclopropanes 23via a chiral aza-ortho-xylylene intermediate, whilst retaining high selectivities. Nitroolefins with both aryl and alkyl substituents at the β position afforded all products in excellent stereoselectivity.
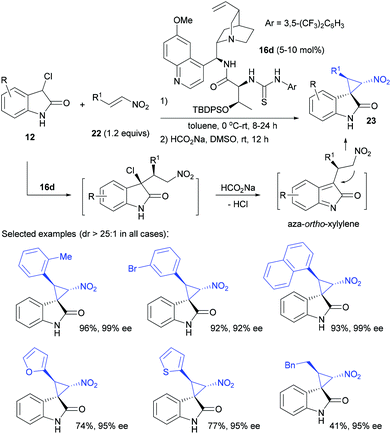 |
| Scheme 5 One pot procedure for asymmetric annulation. | |
In the case of 3-chlorooxindoles as nucleophiles, α,β-unsaturated aldehydes could be used as the Michael acceptors upon iminium catalysis activation. In 2013, Kanger and colleagues reported a highly stereoselective cyclopropanation of enals 25 with 3-chlorooxindole 12 using the proline-derived secondary amine catalyst 24a (Scheme 6).24a,b It was proposed that the initial addition of 12 to iminium ion I formed an enamine intermediate II, followed by intramolecular alkylation and hydrolysis to give the desired products 26 (after in situ reduction) with 44–76% yield, 2
:
1–19
:
1 dr and 36–99% ee.
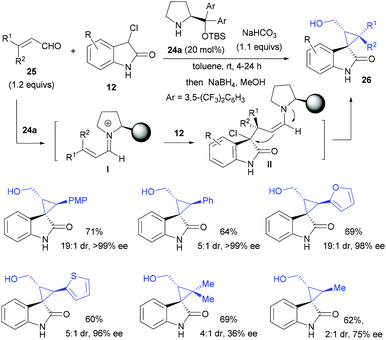 |
| Scheme 6 Asymmetric cyclopropanation via iminium catalysis. | |
The sequential iminium/enamine activation also enabled 2,4-dienals as effective Michael acceptors for cyclopropanation. In their efforts in related areas,25 Melchiorre et al. found that asymmetric 1,6-addition of 12 to linear 2,4-dienals 27 took place at room temperature with complete δ-site selectivity using the second amine 24b as the catalyst (Scheme 7).26 Even though the reactive centre was far away from the chiral centre of the catalyst, the desired products 28 were still obtained in 7
:
1–15
:
1 dr and 92–99% ee. It is noteworthy that installation of a tert-butyl group at the β position of dienal 27 was key to the observed δ-site selectivity, whereas alkyl substituents bearing an enolizable hydrogen atom (Me, nPr, iPr) inhibited the process.
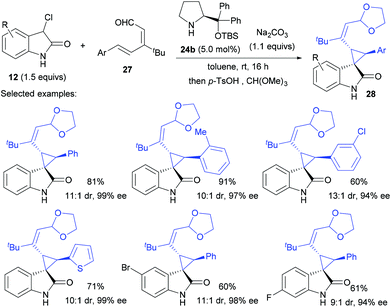 |
| Scheme 7 Asymmetric iminium catalysis with linear 2,4-dienals. | |
While merging oxindole based nucleophiles and electrophiles, it is possible to prepare bis-spirocyclic oxindoles, as evidenced by Kanger's work. The 3-chlorooxindole 12 reacted with 10, under the catalysis of 29, to give bis-spirooxindole 30 in 71–99% yield, 12
:
1–30
:
1 dr and 83–99% ee (Scheme 8).24 To guarantee an excellent dr value, the presence of a C4 substituent R2 on 3-chlorooxindoles was necessary.
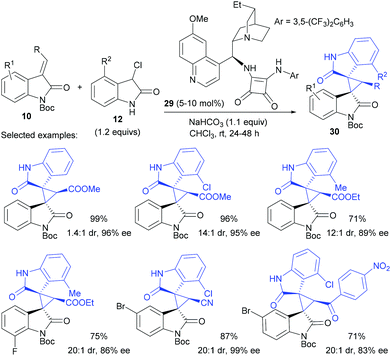 |
| Scheme 8 Asymmetric annulation with 3-chlorooxindoles. | |
2.3 Path 3: using N-Boc oxindoles
The paths 1 and 2 take advantage of the dualistic properties of oxindole derivatives, alkylideneoxindole or 3-chlorooxindole, for the construction of cyclopropanes. Instead, Lu et al. developed a new strategy which directly couples dinucleophilic oxindoles with a suitable dielectrophile such as α-halogenated nitroolefins. As seen in eqn (1) of Scheme 9, when N-Boc oxindole 11 was used as the C1 dinucleophile, asymmetric cyclopropanation using bromonitroolefins 31 was accomplished by utilizing 10 mol% of the amino acid derived multifunctional catalyst 16e developed in their group, in the presence of 1.0 equiv. of ammonium carbonate. This procedure readily provided spiro nitrocyclopropanes 32 in good yield, moderate to excellent dr and excellent ee values.27
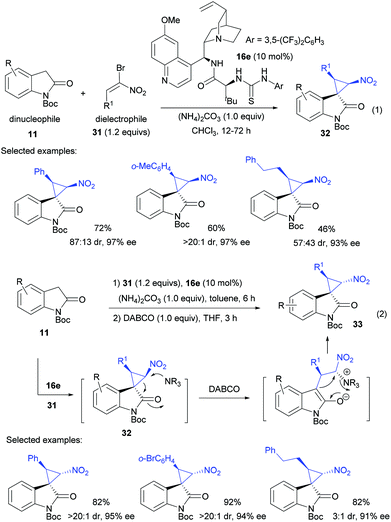 |
| Scheme 9 Asymmetric annulation of oxindoles. | |
Noticeably, the relative configuration of product 32 was different from that of 15, both of which were diastereomers of spiro nitrocyclopropanes (Scheme 2). Therefore, these studies were complementary to each other. Furthermore, adding DABCO as a promoter in the subsequent step enabled the diastereodivergent synthesis of the third thermodynamically more stable diastereomer 33 through a nucleophilic-promoter-initiated cyclopropane ring opening–closing process (eqn (2)). As shown above, the results were generally good for the β-aryl nitroolefins; however, there was still room for improvement in the diastereoselectivity when β-alkyl nitroolefins were used.
3. Transition metal catalysed asymmetric cyclopropanation of diazooxindoles
While the organocatalytic Michael-intramolecular alkylation sequence enables the synthesis of spirocyclopropyl oxindoles from electron-deficient olefins, it fails to incorporate electron-neutral or rich olefins, at least at the current stage. This is probably the limitation of the organocatalytic cyclopropanation strategy. On the other hand, olefin cyclopropanation using diazo compounds is a particularly attractive strategy for converting electron-neutral or rich olefins to cyclopropanes.28 However, despite the long and continuing history of metal-catalysed olefins cyclopropanation using diazo reagents,29 the use of cyclic diazo compounds for the construction of spirocyclic cyclopropanes met with limited success,30 and it is very difficult to achieve highly efficient and stereoselective cyclopropanation of donor–acceptor diazo reagents with 1,2-disubstituted or trisubstituted olefins.31 Therefore, while olefin cyclopropanation using easily available diazooxindole is a straightforward pathway for the synthesis of spirocyclopropyl oxindoles, its catalytic asymmetric versions have been developed only recently.6d
3.1 Path 4: using diazooxindoles
Since the pioneering work of Doyle28a and Davies,32 chiral dirhodium catalysts are popularly used for the decomposition of diazo compounds for olefin cyclopropanation. At the end of 2012, Awata and Arai reported the use of Hashimoto's Rh2(S-PTTL)4
33 to catalyse the cyclopropanation reaction of alkenes and diazooxindoles, and the desired products 34 were obtained in 65–99% yield, with 98
:
2 dr and 48–74% ee (Scheme 10).34 Both aryl and alkyl monosubstituted alkenes were viable substrates by Rh-catalysis.
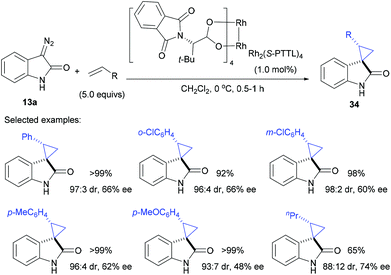 |
| Scheme 10 Asymmetric cyclopropanation using Rh catalysis. | |
Meanwhile, with our continuous efforts in the catalytic asymmetric syntheses of quaternary oxindoles,17 we started to develop catalytic asymmetric reactions based on diazooxindoles. We reported a highly enantioselective mercury-catalyzed olefin cyclopropanation using diazooxindoles, which is also the first mercury-catalyzed cyclopropanation of olefin and diazo reagents.10 We envisioned that the identification of an excellent soft Lewis acid was crucial to overcome the low reactivity of diazooxindoles,16d a kind of soft base.35 Therefore, we carefully screened various soft Lewis acids, and found to our delight that Hg(OTf)2
10 and PPh3AuOTf36 could efficiently decompose diazooxindole 13 for cyclopropanation with styrene even at 0 °C, giving the desired product in good yield with >20
:
1 diastereoselectivity. This result was obviously superior to the performance of other commonly used metal catalysts for this reaction, including Rh2(OAc)4, Cu(OTf)2, CuOTf and Pd(OTf)2.
It turned out that using 2.0–5.0 mol% of (R)-difluorphos3735/Hg(II) catalyzed the cyclopropanation of diazooxindoles with styrene derivatives efficiently, giving the desired product 36 with 40–99% yield, >20
:
1 dr, 60–99% ee (Scheme 11).10 A dramatic ligand acceleration effect was observed, as the use of 0.2 mol% of ligand 35 and 2.0 mol% of Hg(OTf)2 enabled the cyclopropanation reaction of diazooxindole 13a and styrene with 74% yield and 83% ee in toluene, whereas the product was isolated with less than 7% yield in the absence of 35. This demonstrates for the first time that the catalytic properties of Hg(II) could be modulated by the ligand.38
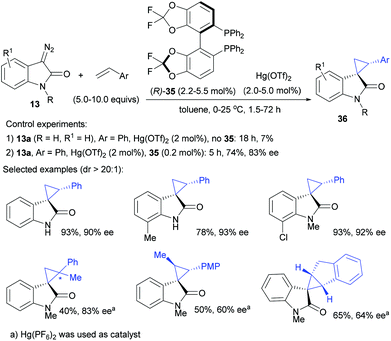 |
| Scheme 11 Cyclopropanation using Hg(II) catalysis. | |
We also tried chiral mercury catalysis in cyclopropanation of diazooxindoles with disubstituted alkenes such as trans-anethole, α-methylstyrene and indene. It was found that while varying the counter anion of the mercury catalyst to PF6−, these reactions could all take place but could give products in moderate ee values, as shown in Scheme 11.
It is worth mentioning that how to achieve highly efficient and enantioselective catalytic asymmetric cyclopropanation of diazo compounds with 1,2-disubstituted alkenes is still very challenging, since the metallocarbene intermediates are very sensitive to the steric hindrance and geometry of alkenes.31 As pointed out by Davies, the trans alkenes are particularly difficult substrates since the competition between C–H insertion and cyclopropanation existed in the reaction of aryldiazoacetates with trans 1-aryl-1-propenes if chiral Rh(II) was employed as the catalyst.31c Furthermore, although Sun and Tang reported in 2012 the first highly stereoselective cyclopropanation of acceptor-only diazoacetate with both cis and trans 1,2-disubstituted alkenes, using a chiral bisoxazoline derived Cu(I) catalyst,31e cyclopropanation of donor/acceptor diazo reagents with 1,2-disubstituted alkenes still remains a challenge.
In view of this challenge, together with the fact that Au(I) proves to be superior to Hg(II) in many reactions,38 we turned our attention to the exploration of Au(I) catalysis in asymmetric olefin cyclopropanation using diazooxindoles, considering the work of Toste39 and Davies40 in related studies. Intensive screenings of various chiral phosphine ligands revealed that the novel spiroketal bisphosphine (SKP) ligand, recently invented by Ding and co-workers,41 has been proved to be a powerful new type of bisphosphine ligand for Au(I)-catalyzed highly stereoselective synthesis of quaternary spirocyclopropyl oxindoles from a broad range of alkenes, such as 1,1-disubstitued, 1,1,2-trisubstituted, simple 1,2-disubstituted cis and trans, and terminal ones. The results are generally excellent with 18–98% yield, excellent dr values (dr > 20
:
1 in all cases) and 70–95% ee (Scheme 12).36 Compared with the previous work using Hg(II), the use of chiral digold catalysis effectively extended the scope of alkenes. However, in the Au(I) catalyzed versions, only unprotected diazooxindoles could give the desired products in excellent ee values, but chiral Hg(II) catalysis was able to allow N-protected diazooxindoles to afford products in excellent ee values as well. This also represents the first example of highly stereoselective Au(I)-catalyzed asymmetric cyclopropanation of diazo reagents.
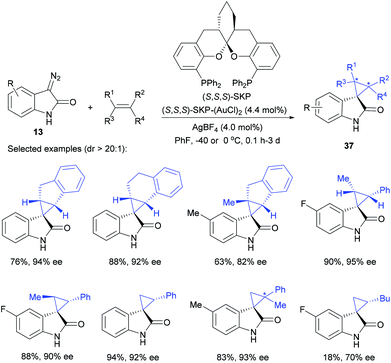 |
| Scheme 12 Asymmetric cyclopropanation using Au(I) catalysis. | |
4. Conclusions
In summary, there are four pathways developed for the synthesis of optically active spirocyclopropyl oxindoles. These research studies persuasively demonstrate the potential of both organocatalytic and metal-catalyzed olefin cyclopropanation reactions in the construction of spirocyclic compounds with fused cyclopropane rings, by using cyclic starting materials. In addition, related studies also contribute to the development of a new catalyst system for the olefin cyclopropanation using diazo compounds. Both organocatalysis and metal catalysis are complementary and orthogonal to each other in terms of the olefin substrates. While organocatalytic methods rely on the use of electron-deficient olefins, the metal-catalyzed protocols are limited to electron-rich and neutral olefins. How to expand the scope of olefins is the next challenging task in this field, which requires the development of new powerful catalysts and new strategies. Considering the recent progress in the metal-catalyzed asymmetric olefin cyclopropanation of electron-deficient olefins,42 it seems that research into the reaction of donor–acceptor diazo reagents and electron-deficient olefins would be an exciting direction to explore. In addition, it is very important to develop new strategies to directly activate easily available unprotected substrates with relatively low reactivity for use and to raise related methods to the standard of ideal asymmetric catalysis.43 In addition, it is highly rewarding to explore the possibility of synthesizing optically active spirocyclopropyl oxindoles bearing three continuous quaternary carbon centres with full control of selectivities. Nevertheless, these advances have provided a variety of structures and stereochemistry diversified spirocyclopropyl oxindoles, bearing two important pharmacophores, which would facilitate structure–activity relationship studies.
Acknowledgements
Financial support from the 973 program (2015CB856600), NSFC (21172075 and 21222204), the Ministry of Education (NCET-11-0147 and PCSIRT) and the Program of SSCS (13XD1401600) is appreciated. Mr Zhong-Yan Cao thanks the financial support of “outstanding doctoral dissertation cultivation plan of action” from East China Normal University.
Notes and references
-
(a) M. E. Welsch, S. A. Snyder and B. R. Stockwell, Curr. Opin. Chem. Biol., 2010, 14, 347 CrossRef CAS PubMed
;
(b) W. R. J. D. Galloway, A. Isidro-LIobet and D. R. Spring, Nat. Commun., 2010, 1, 80 Search PubMed
;
(c) I. Sharma and D. S. Tan, Nat. Chem., 2013, 5, 157 CrossRef CAS PubMed
;
(d) A. W. Hung, A. Ramek, Y. Wang, T. Kaya, J. A. Wilson, P. A. Clemons and D. W. Young, Proc. Natl. Acad. Sci. U. S. A., 2011, 108, 6799 CrossRef CAS PubMed
.
- Y. Zheng, C. M. Tice and S. B. Singh, Bioorg. Med. Chem. Lett., 2014, 24, 3673 CrossRef CAS PubMed
.
-
(a) E. M. Carreira and T. C. Fessard, Chem. Rev., 2014, 114, 8257 CrossRef CAS PubMed
;
(b) J.-H. Xie and Q.-L. Zhou, Acta Chim. Sin., 2014, 72, 778 CrossRef CAS
;
(c) R. Rios, Chem. Soc. Rev., 2012, 41, 1060 RSC
.
-
(a) F. Zhou, Y.-L. Liu and J. Zhou, Adv. Synth. Catal., 2010, 352, 1381 CrossRef CAS PubMed
;
(b) H. Wang and R. Wang, Adv. Synth. Catal., 2013, 355, 1023 CrossRef PubMed
;
(c) N. R. Ball-Jones, J. J. Badillo and A. K. Franz, Org. Biomol. Chem., 2012, 10, 5165 RSC
;
(d) D.-J. Cheng, Y. Ishihara, B. Tan and C. F. Barbas III, ACS Catal., 2014, 4, 743 CrossRef CAS
;
(e) B. M. Trost and M. K. Brennan, Synthesis, 2009, 3003 CrossRef CAS
;
(f) C. V. Galliford and K. A. Scheidt, Angew. Chem., Int. Ed., 2007, 46, 8748 CrossRef CAS PubMed
.
- For selected examples:
(a) M. Rottmann, C. McNamara, B. K. S. Yeung, M. C. S. Lee, B. Zou, B. Russell, P. Seitz, D. M. Plouffe, N. V. Dharia, J. Tan, S. B. Cohen, K. R. Spencer, G. E. González-Páez, S. B. Lakshminarayana, A. Goh, R. Suwanarusk, T. Jegla, E. K. Schmitt, H.-P. Beck, R. Brun, F. Nosten, L. Renia, V. Dartois, T. H. Keller, D. A. Fidock, E. A. Winzeler and T. T. Diagana, Science, 2010, 329, 1175 CrossRef CAS PubMed
;
(b) V. V. Vintonyak, K. Warburg, H. Kruse, S. Grimme, K. Hübel, D. Rauh and H. Waldmann, Angew. Chem., Int. Ed., 2010, 49, 5902 CrossRef CAS PubMed
;
(c) B. K. S. Yeung, B. Zou, M. Rottmann, S. B. Lakshminarayana, S.-H. Ang, S. Y. Leong, J. Tan, J. Wong, S. Keller-Maerki, C. Fischli, A. Goh, E. K. Schmitt, P. Krastel, E. Francotte, K. Kuhen, D. Plouffe, K. Henson, T. Wagner, E. A. Winzeler, F. Peterson, R. Brun, V. Dartois, T. T. Diagana and T. H. Keller, J. Med. Chem., 2010, 53, 5155 CrossRef CAS PubMed
;
(d) T. Jiang, K. L. Kuhen, K. Wolff, H. Yin, K. Bieza, J. Caldwell, B. Bursulaya, T. Y.-H. Wu and Y. He, Bioorg. Med. Chem. Lett., 2006, 16, 2105 CrossRef CAS PubMed
;
(e) T. Jiang, K. L. Kuhen, K. Wolff, H. Yin, K. Bieza, J. Caldwell, B. Bursulaya, T. Tuntland, K.-Y. Zhang, D. Karanewsky and Y. He, Bioorg. Med. Chem. Lett., 2006, 16, 2109 CrossRef CAS PubMed
;
(f)
Y. He, T. Jiang, K. L. Kuhen, D. A. Ellis, B. Wu, T. Y.-H. Wu and B. Bursulaya, PCT Int. Appl, WO 2004/037247 A1, 2004 Search PubMed
;
(g) G. Kumari, Nutan, M. Modi, S. K. Gupta and R. K. Singh, Eur. J. Med. Chem., 2011, 46, 1181 CrossRef CAS PubMed
;
(h)
P. B. Sampson, S.-W. Li, Y. Liu, H. W. Pauls, L. G. Edwards, B. T. Forrest, M. Feher, N. K. B. Patel and G.-H. Pan, PCT Int. Appl, WO 2010/115279 A1, 2010 Search PubMed
;
(i)
H. W. Pauls, S.-W. Li, P. B. Sampson and B. T. Forrest, PCT Int. Appl, WO 2012/048411 A1, 2012 Search PubMed
;
(j)
L. Chen, L.-C. Feng, Y. He, M. W. Huang and H.-Y. Yun, PCT Int. Appl, WO 2011/070039 A1, 2011 Search PubMed
;
(k) Y. Masuyama, N. Kinugawa and Y. Kurusu, J. Org. Chem., 1987, 52, 3704 CrossRef
;
(l)
L. Horoszok, C. Leung, M. Tomaszewski and C. Walpole, PCT Int. Appl, WO2007091946, 2007 Search PubMed
.
-
(a) K. Shen, X.-H. Liu, L.-L. Lin and X.-M. Feng, Chem. Sci., 2012, 3, 327 RSC
;
(b) G. S. Singh and Z. Y. Desta, Chem. Rev., 2012, 112, 6104 CrossRef CAS PubMed
;
(c) Y.-L. Liu, F. Zhou, C.-H. Wang and J. Zhou, Chin. J. Org. Chem., 2013, 33, 1595 CrossRef CAS
;
(d) Z.-Y. Cao, Y.-H. Wang, X.-P. Zeng and J. Zhou, Tetrahedron Lett., 2014, 55, 2571 CrossRef CAS PubMed
.
-
(a) D. Y.-K. Chen, R. H. Pouwer and J.-A. Richard, Chem. Soc. Rev., 2012, 41, 4631 RSC
;
(b) Y. Tang, S. Ye and X.-L. Sun, Synlett, 2005, 2720 CrossRef CAS PubMed
;
(c) L. A. Wessjohann, W. Brandt and T. Thiemann, Chem. Rev., 2003, 103, 1625 CrossRef CAS PubMed
;
(d) R. Faust, Angew. Chem., Int. Ed., 2001, 40, 2251 CrossRef CAS
;
(e) W. A. Donaldson, Tetrahedron, 2001, 57, 8589 CrossRef CAS
;
(f) J. Salaün, Top. Curr. Chem., 2000, 207, 1 Search PubMed
.
-
(a) T. F. Schneider, J. Kaschel and D. B. Werz, Angew. Chem., Int. Ed., 2014, 53, 5504 CrossRef CAS PubMed
;
(b) H.-U. Reissig and R. Zimmer, Chem. Rev., 2003, 103, 1151 CrossRef CAS PubMed
.
-
(a) P. B. Alper, C. Meyers, A. Lerchner, D. R. Siegel and E. M. Carreira, Angew. Chem., Int. Ed., 1999, 38, 3186 CrossRef CAS
;
(b) V. Helan, A. Mills, D. Drewry and D. Grant, J. Org. Chem., 2010, 75, 6693 CrossRef CAS PubMed
;
(c) J. L. Wood, A. A. Holubec, B. M. Stoltz, M. M. Weiss, J. A. Dixon, B. D. Doan, M. F. Shamji, J. M. Chen and T. P. Heffron, J. Am. Chem. Soc., 1999, 121, 6326 CrossRef CAS
;
(d) A. Lerchner and E. M. Carreira, J. Am. Chem. Soc., 2002, 124, 14826 CrossRef CAS PubMed
;
(e) C. Fischer, C. Meyers and E. M. Carreira, Helv. Chim. Acta, 2000, 83, 1175 CrossRef CAS
;
(f) C. Marti and E. M. Carreira, J. Am. Chem. Soc., 2005, 127, 11505 CrossRef CAS PubMed
.
- Z.-Y. Cao, F. Zhou, Y.-H. Yu and J. Zhou, Org. Lett., 2013, 15, 42 CrossRef CAS PubMed
.
-
(a) H. K. Grover, M. R. Emmett and M. A. Kerr, Org. Biomol. Chem., 2015, 13, 655 RSC
;
(b) W. J. Humenny, P. Kyriacou, K. Sapeta, A. Karadeolian and M. A. Kerr, Angew. Chem., Int. Ed., 2012, 51, 11088 CrossRef CAS PubMed
;
(c) C. A. Carson and M. A. Kerr, Chem. Soc. Rev., 2009, 38, 3051 RSC
;
(d) S. K. Jackson, A. Karadeolian, A. B. Driega and M. A. Kerr, J. Am. Chem. Soc., 2008, 130, 4196 CrossRef CAS PubMed
;
(e) C. A. Carson and M. A. Kerr, J. Org. Chem., 2005, 70, 8242 CrossRef CAS PubMed
;
(f) I. S. Young and M. A. Kerr, Org. Lett., 2004, 6, 139 CrossRef CAS PubMed
;
(g) I. S. Young and M. A. Kerr, Angew. Chem., Int. Ed., 2003, 42, 3023 CrossRef CAS PubMed
.
-
(a) W. D. Mackay, M. Fistikci, R. M. Carris and J. S. Johnson, Org. Lett., 2014, 16, 1626 CrossRef CAS PubMed
;
(b) S. M. Wales, M. M. Walker and J. S. Johnson, Org. Lett., 2013, 15, 2558 CrossRef CAS PubMed
;
(c) A. T. Parsons, A. G. Smith, A. J. Neel and J. S. Johnson, J. Am. Chem. Soc., 2010, 132, 9688 CrossRef CAS PubMed
;
(d) A. T. Parsons and J. S. Johnson, J. Am. Chem. Soc., 2009, 131, 3122 CrossRef CAS PubMed
;
(e) P. D. Pohlhaus, S. D. Sanders, A. T. Parsons, W. Li and J. S. Johnson, J. Am. Chem. Soc., 2008, 130, 8642 CrossRef CAS PubMed
;
(f) P. D. Pohlhaus and J. S. Johnson, J. Am. Chem. Soc., 2005, 127, 16014 CrossRef CAS PubMed
;
(g) P. D. Pohlhaus and J. S. Johnson, J. Org. Chem., 2005, 70, 1057 CrossRef CAS PubMed
.
-
(a) S.-H. Liao, X.-L. Sun and Y. Tang, Acc. Chem. Res., 2014, 47, 2260 CrossRef CAS PubMed
;
(b) Y.-Y. Zhou, J. Li, L. Ling, S.-H. Liao, X.-L. Sun and Y. Tang, Angew. Chem., Int. Ed., 2013, 52, 1452 CrossRef CAS PubMed
;
(c) H. Xu, J.-P. Qu, S.-H. Liao, H. Xiong and Y. Tang, Angew. Chem., Int. Ed., 2013, 52, 4004 CrossRef CAS PubMed
;
(d) H. Xiong, H. Xu, S.-H. Liao, Z. Xie and Y. Tang, J. Am. Chem. Soc., 2013, 135, 7851 CrossRef CAS PubMed
;
(e) Y.-Y. Zhou, L.-J. Wang, J. Li, X.-L. Sun and Y. Tang, J. Am. Chem. Soc., 2012, 134, 9066 CrossRef CAS PubMed
;
(f) Y.-B. Kang, X.-L. Sun and Y. Tang, Angew. Chem., Int. Ed., 2007, 46, 3918 CrossRef CAS PubMed
.
-
(a) Y. Xia, X.-H. Liu, H.-F. Zheng, L.-L. Lin and X.-M. Feng, Angew. Chem., Int. Ed., 2015, 54, 227 CrossRef CAS PubMed
;
(b) S. Chakrabarty, I. Chatterjee, B. Wibbeling, C. G. Daniliuc and A. Studer, Angew. Chem., Int. Ed., 2014, 53, 5964 CrossRef CAS PubMed
;
(c) F. de Nanteuil, E. Serrano, D. Perrotta and J. Waser, J. Am. Chem. Soc., 2014, 136, 6239 CrossRef CAS PubMed
;
(d) Y.-Q. Zhang and J.-L. Zhang, Chem. Commun., 2012, 48, 4710 RSC
;
(e) S.-Y. Xing, W.-Y. Pan, C. Liu, J. Ren and Z.-W. Wang, Angew. Chem., Int. Ed., 2010, 49, 3215 CrossRef CAS PubMed
;
(f) Y.-Q. Zhang, F. Liu and J.-L. Zhang, Chem. – Eur. J., 2010, 16, 6146 CrossRef CAS PubMed
;
(g) O. A. Ivanova, E. M. Budynina, Y. K. Grishin, I. V. Trushkov and P. V. Verteletskii, Angew. Chem., Int. Ed., 2008, 47, 1107 CrossRef CAS PubMed
;
(h) M. P. Sibi, Z.-H. Ma and C. P. Jasperse, J. Am. Chem. Soc., 2005, 127, 5764 CrossRef CAS PubMed
.
- The construction of continuous quaternary carbon stereocenters is particularly challenging: E. A. Peterson and L. E. Overman, Proc. Natl. Acad. Sci. U. S. A., 2004, 101, 11943 CrossRef CAS PubMed
.
-
(a) M. K. Eberle, G. G. Kahle and M. J. Shapiro, J. Org. Chem., 1982, 47, 2210 CrossRef CAS
;
(b) S. Muthusamy and C. Gunanathan, Synlett, 2003, 1599 CrossRef CAS PubMed
;
(c) P. Shanmugam, V. Vaithiyanathan and B. Viswambharan, Tetrahedron, 2006, 62, 4342 CrossRef CAS PubMed
;
(d) S.-F. Chen, J. Ma and J.-B. Wang, Tetrahedron Lett., 2008, 49, 6781 CrossRef CAS PubMed
;
(e) S. R. Yong, A. T. Ung, S. G. Pyne, B. W. Skelton and A. H. White, Tetrahedron, 2007, 63, 1191 CrossRef CAS PubMed
;
(f) S. Jaegli, J.-P. Vors, L. Neuville and J.-P. Zhu, Tetrahedron, 2010, 66, 8911 CrossRef CAS PubMed
;
(g) S. Muthusamy, D. Azhagan, B. Gnanaprakasam and E. Suresh, Tetrahedron Lett., 2010, 51, 5662 CrossRef CAS PubMed
;
(h) H. Yu, Y. Liu, H. Zhang, J. Chen, H. Deng, M. Shao, Z.-J. Ren and W.-G. Cao, Tetrahedron, 2010, 66, 2598 CrossRef CAS PubMed
;
(i) T.-R. Li, S.-W. Duan, W. Ding, Y.-Y. Liu, J.-R. Chen, L.-Q. Liu and W.-J. Xiao, J. Org. Chem., 2014, 79, 2296 CrossRef CAS PubMed
;
(j) R. Zhou, C. J. Yang, Y.-Y. Liu, R. F. Li and Z.-J. He, J. Org. Chem., 2014, 79, 10709 CrossRef CAS PubMed
;
(k) S. Muthusamy and R. Ramkumar, Tetrahedron Lett., 2014, 55, 6389 CrossRef CAS PubMed
;
(l) C. L. Ladd, D. S. Roman and A. B. Charette, Org. Lett., 2013, 15, 1350 CrossRef CAS PubMed
;
(m) S. Roy and K. Chen, J. Chin. Chem. Soc., 2013, 60, 597 CrossRef CAS PubMed
.
-
(a) X.-P. Yin, X.-P. Zeng, Y.-L. Liu, F.-M. Liao, J.-S. Yu, F. Zhou and J. Zhou, Angew. Chem., Int. Ed., 2014, 53, 13740 CrossRef CAS PubMed
;
(b) J.-S. Yu, Y.-L. Liu, J. Tang, X. Wang and J. Zhou, Angew. Chem., Int. Ed., 2014, 53, 9512 CrossRef CAS PubMed
;
(c) F. Zhou, C. Tan, J. Tang, Y.-Y. Zhang, W.-M. Gao, H.-H. Wu, Y.-H. Yu and J. Zhou, J. Am. Chem. Soc., 2013, 135, 10994 CrossRef CAS PubMed
;
(d) Y.-L. Liu, X. Wang, Y.-L. Zhao, F. Zhu, X.-P. Zeng, L. Chen, C.-H. Wang, X.-L. Zhao and J. Zhou, Angew. Chem.,
Int. Ed., 2013, 52, 13735 CAS
;
(e) Y.-L. Liu, B.-L. Wang, J.-J. Cao, L. Chen, Y.-X. Zhang, C. Wang and J. Zhou, J. Am. Chem. Soc., 2010, 132, 15176 CrossRef CAS PubMed
;
(f) M. Ding, F. Zhou, Y.-L. Liu, C.-H. Wang, X.-L. Zhao and J. Zhou, Chem. Sci., 2011, 2, 2035 RSC
;
(g) Y.-L. Liu, F.-M. Liao, Y.-F. Niu, X.-L. Zhao and J. Zhou, Org. Chem. Front., 2014, 1, 742 RSC
;
(h) W.-M. Gao, J.-S. Yu, Y.-L. Zhao, Y.-L. Liu, F. Zhou, H.-H. Wu and J. Zhou, Chem. Commun., 2014, 50, 15179 RSC
;
(i) Y.-H. Wang, Z.-Y. Cao, Y.-F. Niu, X.-L. Zhao and J. Zhou, Acta Chim. Sin., 2014, 72, 867 CrossRef CAS
;
(j) F. Zhou, X.-P. Zeng, C. Wang, X.-L. Zhao and J. Zhou, Chem. Commun., 2013, 49, 2022 RSC
;
(k) Y.-L. Liu and J. Zhou, Chem. Commun., 2013, 49, 4421 RSC
;
(l) Y.-L. Liu and J. Zhou, Chem. Commun., 2012, 48, 1919 RSC
;
(m) Y.-L. Liu and J. Zhou, Acta Chim. Sin., 2012, 70, 1451 CrossRef CAS
;
(n) Z.-Y. Cao, Y. Zhang, C.-B. Ji and J. Zhou, Org. Lett., 2011, 13, 6398 CrossRef CAS PubMed
;
(o) Y.-L. Liu, T.-D. Shi, F. Zhou, X.-L. Zhao, X. Wang and J. Zhou, Org. Lett., 2011, 13, 3826 CrossRef CAS PubMed
;
(p) F. Zhou, M. Ding, Y.-L. Liu and J. Zhou, Adv. Synth. Catal., 2011, 353, 2945 CrossRef CAS PubMed
;
(q) Y.-L. Liu, F. Zhou, J.-J. Cao, C.-B. Ji, M. Ding and J. Zhou, Org. Biomol. Chem., 2010, 8, 3847 RSC
;
(r) Z.-Q. Qian, F. Zhou, T.-P. Du, B.-L. Wang, M. Ding, X.-L. Zhao and J. Zhou, Chem. Commun., 2009, 6753 RSC
;
(s) J.-S. Yu, F.-M. Liao, W.-M. Gao, K. Liao, R.-L. Zuo and J. Zhou, Angew. Chem., Int. Ed., 2015, 54 DOI:10.1002/anie.201501747
.
-
A. Kamimura, [2+1] Type Cyclopropanation Reactions, in Methods and Applications of Cycloaddition Reactions in Organic Syntheses, ed. N. Nishiwaki, John Wiley & Sons, Inc., Hoboken, NJ, USA, 2014, p. 1 Search PubMed
.
-
(a) R. K. Kunz and D. W. C. MacMillan, J. Am. Chem. Soc., 2005, 127, 3240 CrossRef CAS PubMed
;
(b) H.-X. Xie, L.-S. Zu, H. Li, J. Wang and W. Wang, J. Am. Chem. Soc., 2007, 129, 10886 CrossRef CAS PubMed
;
(c) R. Rios, H. Sundén, J. Vesely, G.-L. Zhao, P. Dziedzic and A. Córdova, Adv. Synth. Catal., 2007, 349, 1028 CrossRef CAS PubMed
;
(d) T. Inokuma, S. Sakamoto and Y. Takemoto, Synlett, 2009, 1627 CAS
.
- F. Pesciaioli, P. Righi, A. Mazzanti, G. Bartoli and G. Bencivenni, Chem. – Eur. J., 2011, 17, 2842 CrossRef CAS PubMed
.
- A. Noole, N. S. Sucman, M. A. Kabeshov, T. Kanger, F. Z. Macaev and A. V. Malkov, Chem. – Eur. J., 2012, 18, 14929 CrossRef CAS PubMed
.
- M. Ošeka, A. Noole, S. Žari, M. Öeren, I. Järving, M. Lopp and T. Kanger, Eur. J. Org. Chem., 2014, 3599 CrossRef PubMed
.
- X.-W. Dou, W.-J. Yao, B. Zhou and Y.-X. Lu, Chem. Commun., 2013, 49, 9224 RSC
.
-
(a) A. Noole, M. Ošeka, T. Pehk, M. Öeren, I. Järving, M. R. J. Elsegood, A. V. Malkov, M. Lopp and T. Kanger, Adv. Synth. Catal., 2013, 355, 829 CrossRef CAS PubMed
;
(b) A. Noole, A. V. Malkov and T. Kanger, Synthesis, 2013, 2520 CAS
;
(c) A. Noole, I. Järving, F. Werner, M. Lopp, A. Malkov and T. Kanger, Org. Lett., 2012, 14, 4922 CrossRef CAS PubMed
.
- I. D. Jurberg, I. Chatterjee, R. Tannert and P. Melchiorre, Chem. Commun., 2013, 49, 4869 RSC
.
- R. C. da Silva, I. Chatterjee, E. Escudero-Adán, M. W. Paixão and P. Melchiorre, Asian J. Org. Chem., 2014, 3, 466 CrossRef PubMed
.
- X.-W. Dou and Y.-X. Lu, Chem. – Eur. J., 2012, 18, 8315 CrossRef CAS PubMed
.
- For selected reviews:
(a) M. P. Doyle and D. C. Forbes, Chem. Rev., 1998, 98, 911 CrossRef CAS PubMed
;
(b) H. Lebel, J.-F. Marcoux, C. Molinaro and A. B. Charette, Chem. Rev., 2003, 103, 977 CrossRef CAS PubMed
;
(c) Z.-H. Zhang and J.-B. Wang, Tetrahedron, 2008, 64, 6577 CrossRef CAS PubMed
;
(d) H. Pellissier, Tetrahedron, 2008, 64, 7041 CrossRef CAS PubMed
.
- For selected examples, Rh-catalysis:
(a) Y. Lou, M. Horikawa, R. A. Kloster, N. A. Hawryluk and E. J. Corey, J. Am. Chem. Soc., 2004, 126, 8916 CrossRef CAS PubMed
;
(b) A. DeAngelis, O. Dmitrenko, G. P. A. Yap and J. M. Fox, J. Am. Chem. Soc., 2009, 131, 7230 CrossRef CAS PubMed
;
(c) S. Chuprakov, S. W. Kwok, L. Zhang, L. Lercher and V. V. Fokin, J. Am. Chem. Soc., 2009, 131, 18034 CrossRef CAS PubMed
;
(d) C.-M. Qin, V. Boyarskikh, J. H. Hansen, K. I. Hardcastle, D. G. Musaev and H. M. L. Davies, J. Am. Chem. Soc., 2011, 133, 19198 CrossRef CAS PubMed
; For Co-catalysis:
(e) A. Nakamura, A. Konishi, Y. Tatsuno and S. Otsuka, J. Am. Chem. Soc., 1978, 100, 3443 CrossRef CAS
;
(f) S.-F. Zhu, J. A. Perman and X. P. Zhang, Angew. Chem., Int. Ed., 2008, 47, 8460 CrossRef CAS PubMed
;
(g) X. Xu, H.-J. Lu, J. V. Ruppel, X. Cui, S. L. de Mesa, L. Wojtas and X. P. Zhang, J. Am. Chem. Soc., 2011, 133, 15292 CrossRef CAS PubMed
;
(h) B. Morandi, B. Mariampillai and E. M. Carreira, Angew. Chem., Int. Ed., 2011, 50, 1101 CrossRef CAS PubMed
; For Ru-catalysis:
(i) Z.-J. Xu, R. Zhang, C.-Y. Zhao, J.-S. Huang, G.-Y. Li, N.-Y. Zhu and C.-M. Che, J. Am. Chem. Soc., 2009, 131, 4405 CrossRef CAS PubMed
;
(j) A. Abu-Elfotoh, K. Phomkeona, K. Shibatomi and S. Iwasa, Angew. Chem., Int. Ed., 2010, 49, 8439 CrossRef CAS PubMed
; For other metals:
(k) J. R. Wolf, C. G. Hamaker, J. P. Djukic, T. Kodadek and L. K. Woo, J. Am. Chem. Soc., 1995, 117, 9194 CrossRef CAS
;
(l) S. Kanchiku, H. Suematsu, K. Matsumoto, T. Uchida and T. Katsuki, Angew. Chem., Int. Ed., 2007, 46, 3889 CrossRef CAS PubMed
.
-
(a) D. Bykowski, K.-H. Wu and M. P. Doyle, J. Am. Chem. Soc., 2006, 128, 16038 CrossRef CAS PubMed
;
(b) M. Ichinose, H. Suematsu and T. Katsuki, Angew. Chem., Int. Ed., 2009, 48, 3121 CrossRef CAS PubMed
.
-
(a) N. Østergaard, J. F. Jensen and D. Tanner, Tetrahedron, 2001, 57, 6083 CrossRef
;
(b) H. Suematsu, S. Kanchiku, T. Uchida and T. Katsuki, J. Am. Chem. Soc., 2008, 130, 10327 CrossRef CAS PubMed
;
(c) H. M. L. Davies, M. G. Coleman and D. L. Ventura, Org. Lett., 2007, 9, 4971 CrossRef CAS PubMed
;
(d) D. L. Ventura, Z. Li, M. G. Coleman and H. M. L. Davies, Tetrahedron, 2009, 65, 3052 CrossRef CAS PubMed
;
(e) J. Li, S.-H. Liao, H. Xiong, Y.-Y. Zhou, X.-L. Sun, Y. Zhang, X.-G. Zhou and Y. Tang, Angew. Chem., Int. Ed., 2012, 51, 8838 CrossRef CAS PubMed
.
-
H. M. L. Davies and B. T. Parr, Rhodium Carbenes, in Contemporary Carbene Chemistry, ed. R. A. Moss and M. P. Doyle, John Wiley & Sons, Inc., Hoboken, NJ, USA, 2014, p. 363 Search PubMed
.
- N. Watanabe, T. Ogawa, Y. Ohtake, S. Ikegami and S. Hashimoto, Synlett, 1996, 85 CrossRef CAS PubMed
.
- A. Awata and T. Arai, Synlett, 2013, 29 CAS
.
- T.-L. Ho, Chem. Rev., 1975, 75, 1 CrossRef CAS
.
- Z.-Y. Cao, X.-M. Wang, C. Tan, X.-L. Zhao, J. Zhou and K.-L. Ding, J. Am. Chem. Soc., 2013, 135, 8197 CrossRef CAS PubMed
.
- J.-P. Genet, T. Ayad and V. Ratovelomanana-Vidal, Chem. Rev., 2014, 114, 2824 CrossRef CAS PubMed
.
- A. Leyva-Pérez and A. Corma, Angew. Chem., Int. Ed., 2012, 51, 614 CrossRef PubMed
.
- M. J. Johansson, D. J. Gorin, S. T. Staben and F. D. Toste, J. Am. Chem. Soc., 2005, 127, 18002 CrossRef CAS PubMed
.
- J. F. Briones and H. M. L. Davies, J. Am. Chem. Soc., 2012, 134, 11916 CrossRef CAS PubMed
.
-
(a) X.-M. Wang, Z.-B. Han, Z. Wang and K.-L. Ding, Angew. Chem., Int. Ed., 2012, 51, 936 CrossRef CAS PubMed
;
(b) X.-M. Wang, F.-Y. Meng, Y. Wang, Z.-B. Han, Y.-J. Chen, L. Liu, Z. Wang and K.-L. Ding, Angew. Chem., Int. Ed., 2012, 51, 9410 CrossRef PubMed
;
(c) X.-M. Wang, P.-H. Guo, Z.-B. Han, X.-B. Wang, Z. Wang and K.-L. Ding, J. Am. Chem. Soc., 2014, 136, 405 CrossRef CAS PubMed
.
-
(a) H.-B. Wang, D. M. Guptill, A. Varela-Alvarez, D. G. Musaev and H. M. L. Davies, Chem. Sci., 2013, 4, 2844 RSC
;
(b) V. N. G. Lindsay, D. Fiset, P. J. Gritsch, S. Azzi and A. B. Charette, J. Am. Chem. Soc., 2013, 135, 1463 CrossRef CAS PubMed
;
(c) W. I. Dzik, X. Xu, X. P. Zhang, J. N. H. Reek and B. de Bruin, J. Am. Chem. Soc., 2010, 132, 10891 CrossRef CAS PubMed
;
(d) Y. Chen, J. V. Ruppel and X. P. Zhang, J. Am. Chem. Soc., 2007, 129, 12074 CrossRef CAS PubMed
;
(e) J. A. Miller, W. Jin and S. T. Nguyen, Angew. Chem., Int. Ed., 2002, 41, 2953 CrossRef CAS
.
-
J. Zhou, Multicatalyst system in asymmetric catalysis, John Wiley & Sons, New York, 2014, ch. 1 Search PubMed
.
|
This journal is © the Partner Organisations 2015 |
Click here to see how this site uses Cookies. View our privacy policy here.