DOI:
10.1039/C5QO00164A
(Research Article)
Org. Chem. Front., 2015,
2, 1040-1044
A concise construction of carbohydrate-tethered axially chiral allenes via copper catalysis†
Received
20th May 2015
, Accepted 15th June 2015
First published on 16th June 2015
Abstract
The efficient construction of the axial chirality of allenes bearing a carbohydrate unit is challenging. Here we observed that the newly developed CuBr2 catalysis may smoothly catalyze the highly diastereoselective three-component reaction of carbohydrates bearing a terminal alkyne unit, aliphatic or aromatic aldehydes, and (R) or (S)-α,α-diphenylprolinol, affording carbohydrates bearing the chiral 1,3-substituted allene entity in very high de (94–99%). Synthetically attractive functionalities, such as hydroxyl, amide, malonate, and even tosylate, etc. could all be well tolerated. The role of the carbohydrate unit has been explored.
It is well known that chirality is a very common and attractive phenomenon existing in nature. For example, the left hand is a non-superimposable mirror image of the right hand. At the molecular level, organic molecules may contain central, axial, planar, and helical chiralities, etc., among which central chirality is the most frequently observed and closely related to the biological activity. Thus, the enantioselective construction of central chirality has been extensively explored. Allenes are a class of compounds with an axial chirality of their two cumulated C
C bonds.1 In comparison, the axial chirality of allenes has received much less attention and is far from being well established.2,3 On the other hand, some natural or unnatural carbohydrates bearing allene units show very interesting biological activities, and the allene unit was even found in some marketed drugs (Fig. 1).4 Considering the biopotential of both carbohydrates and allenes as well as the utility of the chiral allene unit for construction of other units with central chirality via chirality transfer, the development of carbohydrates bearing a chiral allene unit is of high interest and importance.
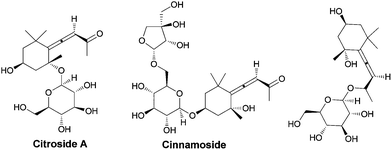 |
| Fig. 1 Some typical natural or unnatural carbohydrates bearing an optically active allene unit with biopotential. | |
We chose 2-propynyl 2,3,4,6-tetra-O-acetyl-β-D-glucopyranoside 1a5 as the model substrate. The recently developed ZnX2-mediated one-pot synthesis of optically active 1,3-disubstituted allenes from terminal alkynes with aldehydes and α,α-diphenylprolinol is straightforward.6,7 However, initial experiments showed that the reaction under our previously developed protocols with 0.75 equiv. of ZnBr2,6 or even CdI2,
8 both failed to afford the expected product (Ra)-4aa (Scheme 1). Quite unexpectedly, we observed that the reaction using newly developed CuBr2 catalysis9 could solve this problem, affording the carbohydrates bearing an allene unit in decent yields with excellent diastereomeric excess!
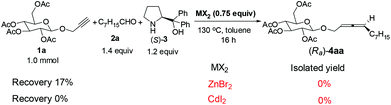 |
| Scheme 1 Failed ZnBr2- or CdI2-mediated reaction of 1a with 2a in the presence of (S)-α,α-diphenylprolinol. | |
Initially, we conducted the reaction of 1a with octanal 2a (1.4 equiv.) and (S)-α,α-diphenylprolinol (S)-3 (1.2 equiv.) under catalysis by CuBr2 (40 mol%) in 1,4-dioxane under reflux. Fortunately, after 16 h, the reaction succeeded to afford the expected product (Ra)-4aa in 59% yield with 94% de (entry 1, Table 1). As a comparison, when CuI10 was applied, the reaction afforded the expected product (Ra)-4aa in 67% yield, however, the diastereoselectivity is rather disappointing (29%) (entry 2, Table 1); compared with CuI, the reaction using CuBr gave (Ra)-4aa in the same yield, however, there is essentially no chiral induction for the allene axial chirality (entry 3, Table 1). The reaction in dioxane with ZnBr2 still failed, indicating the beauty of the Cu2+ catalyst for such a complicated coordination environment of carbohydrates (entry 4, Table 1). Furthermore, it is interesting to observe that both the yield and de depend on the loading of the catalyst (entries 5–8, Table 1): when reducing the loading of CuBr2 to 20 mol%, the diastereomeric excess was improved further to 98% (entry 5, Table 1). Control experiments show that the solvent effect is also striking: when we ran this reaction with CuBr2 (20 mol%) in toluene, the diastereoselectivity is only 86% with a yield of 41% (entry 9, Table 1).
Table 1 Identifying a catalytic diastereoselective synthesis of (Ra)-4aa

|
Entry |
Cat. (mol%) |
(Ra)-4aa |
Isolated yield (%) |
de (%) |
46% of 1a was recovered.
Toluene was used as the solvent.
|
1 |
CuBr2 (40) |
59 |
94 |
2 |
CuI (40) |
67 |
29 |
3 |
CuBr (40) |
67 |
1 |
4 |
ZnBr2 (40) |
0a |
— |
5
|
CuBr
2
(20)
|
49
|
98
|
6 |
CuBr2 (30) |
53 |
96 |
7 |
CuBr2 (50) |
54 |
91 |
8 |
CuBr2 (60) |
57 |
88 |
9b |
CuBr2 (20) |
41 |
86 |
The scope of the aldehydes has been demonstrated by using 1a as the terminal alkyne with some of the typical results summarized in Table 1: both n-alkyl (entries 1–4, Table 2) and sec-alkyl (entries 5–6, Table 2) aldehydes, and even aromatic aldehydes 2g and 2h (at a much lower temperature) (entries 7–8, Table 2) may be used, affording the corresponding axially chiral allenyl glucopyranosides in decent yields with 97–99% de.
Table 2 The scope of aldehydes with 2-propynyl 2,3,4,6-tetra-O-acetyl-β-D-glucopyranoside 1a

|
Entry |
R |
(Ra)-4 |
Isolated yield (%) |
de (%) |
The reaction was conducted at 70 °C for 24 h and 40 mol% of CuBr2 was used.
|
1 |
n-C7H15 (2a) |
49 ((Ra)-4aa) |
98 |
2 |
Bn (2b) |
55 ((Ra)-4ab) |
97 |
3 |
PhCH2CH2 (2c) |
50 ((Ra)-4ac) |
99 |
4 |
i-Bu (2d) |
54 ((Ra)-4ad) |
97 |
5 |
Cy (2e) |
57 ((Ra)-4ae) |
99 |
6 |
i-Pr (2f) |
53 ((Ra)-4af) |
99 |
7a |
p-ClC6H4 (2g) |
54 ((Ra)-4ag) |
99 |
8a |
p-MeC6H4 (2h) |
50 ((Ra)-4ah) |
98 |
The absolute configuration of the allene unit was established by the X-ray single crystal diffraction study of (Ra)-4ae with the glucopyranoside as the reference (Fig. 2).11
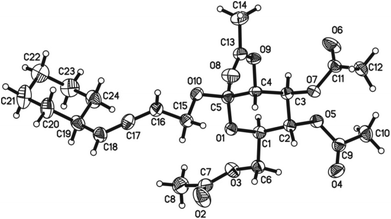 |
| Fig. 2 ORTEP representation of (Ra)-4ae. | |
The reaction is also general with other carbohydrate-based terminal alkynes bearing different synthetically attractive functionalities without protection: for propargyl β-D-glucopyranosides 1b–1d12 bearing –NHCOC7H15, –CH(CO2Me)2, even –OTs unit at the C-6 position, the reactions all afforded the corresponding axially chiral allenyl β-D-glucopyranosides (Ra)-4be–4de in moderate yields with 98–99% de. When propargyl galactopyranoside 1e5 was used, the reaction afforded (Ra)-4ee in 50% yield with 96% de. It is worthy of mention that even the propargyl β-D-glucopyranoside 1o with four free hydroxyl groups without protection could be converted to (Ra)-4ob in 47% yield with 94% de (Scheme 2).
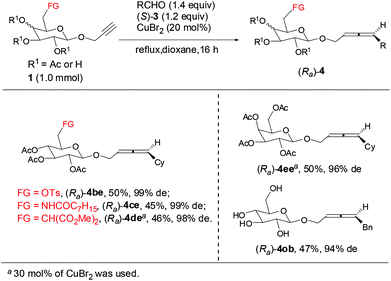 |
| Scheme 2 The scope of propargyl glycosides. | |
Propargyl β-D-thioglucoside 1f13 could also be converted with 46% yield and 96% de to (Ra)-4fe, indicating that even the thio unit does not poison the Cu2+ catalyst (eqn (1)).
| 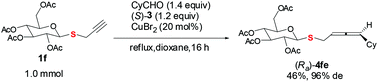 | (1) |
For homopropargyl β-D-glucopyranoside 1g,14 the expected product (Ra)-4ge was obtained in 37% yield with 98% de under catalysis with 20 mol% CuBr2. Increasing the amount of CuBr2 to 30 mol% could increase the yield to 42% with 97% de (Scheme 3).
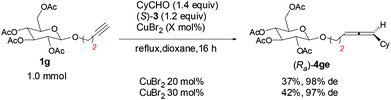 |
| Scheme 3 Synthesis of axially chiral 3,4-allenyl β-D-glucopyranoside (Ra)-4ge. | |
This protocol is even suitable for the synthesis of more complex axially chiral allenyl disaccharides (Ra)-4he (48% yield, 98% de) and (Ra)-4ie (51% yield, 98% de) from 1h and 1i15 (Scheme 4).
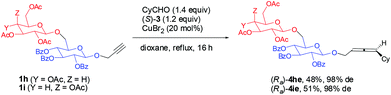 |
| Scheme 4 Synthesis of axially chiral 2,3-allenyl disaccharides (Ra)-4he and (Ra)-4ie. | |
When we used the glucopyranoside 1j bearing two terminal alkyne units at the O-2 and O-3 positions and a terminal allene unit, the reaction afforded the corresponding product (Ra,Ra)-4jb without touching the acetal and terminal allene units in 43% yield with 97% de (dr > 99/1), indicating that the axial allene chirality could be constructed at both α- and β-positions (eqn (2)).
| 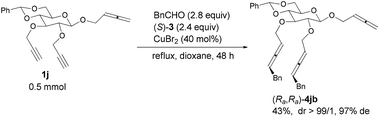 | (2) |
Moreover, this method could be extended to construct axially chiral 2,3-allenyl five-membered furanosides in moderate yields with 96–99% de. No reaction occurred at the acetal unit (Table 3).
Table 3 The scope of aldehydes with propargyl furanoside 1k
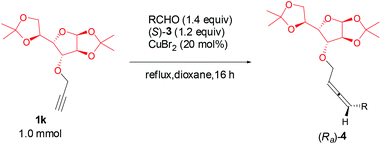
|
Entry |
R |
(Ra)-4 |
Isolated yield (%) |
de (%) |
The reaction was conducted at 70 °C for 24 h and 40 mol% of CuBr2 was used.
|
1 |
n-C7H15 (2a) |
52 ((Ra)-4ka) |
97 |
2 |
Cy (2e) |
56 ((Ra)-4ke) |
96 |
3a |
p-MeC6H4 (2h) |
49 ((Ra)-4kh) |
98 |
4a |
Ph (2i) |
50 ((Ra)-4ki) |
98 |
5a |
m-ClC6H4 (2j) |
46 ((Ra)-4kj) |
99 |
The reaction may be easily conducted on a gram scale showing its practicality (eqn (3), Scheme 5). The diastereoisomer (Sa)-4aa could be prepared with the prolinol enantiomer (R)-3 with a similar level of de, indicating the absolute configuration of the allene axial chirality is determined by that of the amino alcohol 3, not the skeleton of the carbohydrate (eqn (4), Scheme 5). However, it is interesting to observe that under the same conditions the reaction of propargyl alcohol 1y afforded chiral 2,3-allenol (Ra)-4ya in only 39% yield with just 83% ee,9a indicating the unique role of the carbohydrate unit in both the yield and de – the sterically bulky carbohydrate unit ensures the stereoselectivity of the allene formation as a stereodictating protecting group (eqn (5), Scheme 5).6b
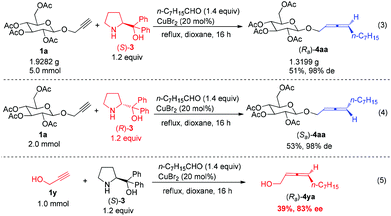 |
| Scheme 5 Control experiments. | |
In conclusion, we have observed that CuBr2 may catalyze the 1,3-disubstituted allene unit bearing a carbohydrate unit from aldehydes and carbohydrates bearing a terminal alkyne unit with very high diastereoselectivity. The following advances have been realized: (1) CuBr2 has been identified as a catalyst compatible with the in situ generated water, the highly oxygenated nature of the carbohydrates, and the in situ generated imine; (2) the current method provides one-pot approach to chiral allenes with common functionalities such as hydroxyl, amide, malonate, even tosylate, enjoying a very wide scope. (3) The absolute configuration of the newly formed allene unit is determined by the absolute configuration of the readily available and cheap (S)- or (R)-α,α-diphenylprolinol,16 while the carbohydrate unit acts as a stereodictating group to improve the diastereomeric excess. This one-pot highly diastereoselective approach for the construction of the chiral allene unit will greatly stimulate interest in the science of allenes and carbohydrates for organic chemists and medicinal chemists. Further studies including the synthetic application are being pursued in this laboratory.
Acknowledgements
Financial support from the National Natural Science Foundation of China (21232006) and National Natural Basic Research Program of China (2015CB856600) are greatly appreciated. We thank Mr Hongwen Luo in this group for reproducing the preparation of (Ra)-4ac, (Ra)-4de. Shengming Ma is a Qiu Shi Adjunct Professor at Zhejiang University.
Notes and references
-
(a) S. Yu and S. Ma, Angew. Chem., Int. Ed., 2012, 51, 3074 CrossRef CAS PubMed;
(b) Progress in allene chemistry, Chem. Soc. Rev., 2014, 43(9), 2879–3206.
- For selected reviews, see:
(a) L. K. Sydnes, Chem. Rev., 2003, 103, 1133 CrossRef CAS PubMed;
(b) N. Krause and A. Hoffmann-Röder, Tetrahedron, 2004, 60, 11671 CrossRef CAS;
(c) K. M. Brummond and J. E. DeForrest, Synthesis, 2007, 795 CrossRef CAS;
(d) M. Ogasawara, Tetrahedron: Asymmetry, 2009, 20, 259 CrossRef CAS;
(e) S. Yu and S. Ma, Chem. Commun., 2011, 47, 5384 RSC;
(f) R. K. Neff and D. E. Frantz, ACS Catal., 2014, 4, 519 CrossRef CAS;
(g) J. Ye and S. Ma, Org. Chem. Front., 2014, 1, 1210 RSC.
- For selected reports on the synthesis of chiral allenes, see:
(a) J.-L. Luche, E. Barreiro, J.-M. Dollat and P. Crabbé, Tetrahedron Lett., 1975, 16, 4615 CrossRef;
(b) A. Claesson and L.-I. Olsson, Acta Chem. Scand. Ser. B, 1979, 33, 679 Search PubMed;
(c) C. J. Elsevier, P. Vermeer, A. Gedanken and W. Runge, J. Org. Chem., 1985, 50, 364 CrossRef CAS;
(d) M. Yoshida, T. Okada and K. Shishido, Tetrahedron, 2007, 63, 6996 CrossRef CAS;
(e) A. G. Myers and B. Zheng, J. Am. Chem. Soc., 1996, 118, 4492 CrossRef CAS;
(f) H. Ohmiya, U. Yokobori, Y. Makida and M. Sawamura, Org. Lett., 2011, 13, 6312 CrossRef CAS PubMed;
(g) M. R. Uehling, S. T. Marionni and G. Lalic, Org. Lett., 2012, 14, 362 CrossRef CAS PubMed;
(h) M. Yang, N. Yokokawa, H. Ohmiya and M. Sawamura, Org. Lett., 2012, 14, 816 CrossRef CAS PubMed;
(i) Y. Imada, M. Nishida, K. Kutsuwa, S.-I. Murahashi and T. Naota, Org. Lett., 2005, 7, 5837 CrossRef CAS PubMed;
(j) B. M. Trost, D. R. Fandrick and D. C. Dinh, J. Am. Chem. Soc., 2005, 127, 14186 CrossRef CAS PubMed;
(k) Y. Imada, M. Nishida and T. Naota, Tetrahedron Lett., 2008, 49, 4915 CrossRef CAS;
(l) M. Ogasawara, H. Ikeda, T. Nagano and T. Hayashi, J. Am. Chem. Soc., 2001, 123, 2089 CrossRef CAS PubMed;
(m) M. Ogasawara, K. Ueyama, T. Nagano, Y. Mizuhata and T. Hayashi, Org. Lett., 2003, 5, 217 CrossRef CAS PubMed;
(n) M. Ogasawara, T. Nagano and T. Hayashi, J. Org. Chem., 2005, 70, 5764 CrossRef CAS PubMed;
(o) M. Ogasawara, Y. Ge, A. Okada and T. Takahashi, Eur. J. Org. Chem., 2012, 1656 CrossRef CAS;
(p) T. Nemoto, M. Kanematsu, S. Tamura and Y. Hamada, Adv. Synth. Catal., 2009, 351, 1773 CrossRef CAS;
(q) W. Zhang, H. Xu, H. Xu and W. Tang, J. Am. Chem. Soc., 2009, 131, 3832 CrossRef CAS PubMed;
(r) H. Qian, X. Yu, J. Zhang and J. Sun, J. Am. Chem. Soc., 2013, 135, 18020 CrossRef CAS PubMed;
(s) I. T. Crouch, R. K. Neff and D. E. Frantz, J. Am. Chem. Soc., 2013, 135, 4970 CrossRef CAS PubMed;
(t) T. Hashimoto, K. Sakata, F. Tamakuni, M. J. Dutton and K. Maruoka, Nat. Chem., 2013, 5, 240 CrossRef CAS PubMed;
(u) Y. Wang, W. Zhang and S. Ma, J. Am. Chem. Soc., 2013, 135, 11517 CrossRef CAS PubMed.
- A. Hoffmann-Röder and N. Krause, Angew. Chem., Int. Ed., 2004, 43, 1196 CrossRef PubMed.
- H. B. Mereyala and S. R. Gurrala, Carbohydr. Res., 1998, 307, 351 CrossRef CAS.
-
(a) J. Ye, S. Li, B. Chen, W. Fan, J. Kuang, J. Liu, Y. Liu, B. Miao, B. Wan, Y. Wang, X. Xie, Q. Yu, W. Yuan and S. Ma, Org. Lett., 2012, 14, 1346 CrossRef CAS PubMed;
(b) J. Ye, W. Fan and S. Ma, Chem. – Eur. J., 2013, 19, 716 CrossRef CAS PubMed;
(c) J. Ye, R. Lü, W. Fan and S. Ma, Tetrahedron, 2013, 69, 8959 CrossRef CAS;
(d) R. Lü, J. Ye, T. Cao, B. Chen, W. Fan, W. Lin, J. Liu, H. Luo, B. Miao, S. Ni, X. Tang, N. Wang, Y. Wang, X. Xie, Q. Yu, W. Yuan, W. Zhang, C. Zhu and S. Ma, Org. Lett., 2013, 15, 2254 CrossRef PubMed;
(e) X. Zhang, Y. Qiu, C. Fu and S. Ma, Org. Chem. Front., 2014, 1, 247 RSC.
- Periasamy et al. also reported the synthesis of axially chiral allenes from simple non-functionalized terminal alkynes by using α,α-diphenylprolinol right after our report (ref. 6a); we have not been able to reproduce their results in terms of ee and yield: the calibrated specific optical rotations of the same allenes with similar ee values from our study and the data in their report are different. The problem is most probably caused by inaccurate HPLC analysis for both the racemic and optically active allenes: M. Periasamy, N. Sanjeevakumar, M. Dalai, R. Gurubrahamam and P. O. Reddy, Org. Lett., 2012, 14, 2932 CrossRef CAS PubMed . For details, see: ref. 6c. Later, we developed the ZnBr2–CuBr-mediated protocol for simple terminal alkynes (see ref. 6d).
- X. Tang, C. Zhu, T. Cao, J. Kuang, W. Lin, S. Ni, J. Zhang and S. Ma, Nat. Commun., 2013, 4, 2450, DOI:10.1038/ncomms3450.
-
(a) X. Huang, T. Cao, Y. Han, X. Jiang, W. Lin, J. Zhang and S. Ma, Chem. Commun., 2015, 51, 6956 RSC;
(b) X. Tang, X. Huang, T. Cao, Y. Han, X. Jiang, W. Lin, Y. Tang, J. Zhang, Q. Yu, C. Fu and S. Ma, Org. Chem. Front., 2015, 2, 688 RSC.
- J. Kuang, H. Luo and S. Ma, Adv. Synth. Catal., 2012, 354, 933 CrossRef CAS.
- Crystal data for compound (Ra)-4ae: C24H34O10, MW = 482.51, orthorhombic, space group P212121, final R indices [I > 2σ(I)], R1 = 0.0800, wR2 = 0.2036, R indices (all data) R1 = 0.1020, wR2 = 0.2267, a = 5.8507(10) Å, b = 14.177(2) Å, c = 30.834(4) Å, α = 90.00°, β = 90.00°, γ = 90.00°, V = 2557.5(7) Å3, T = 293(2) K, Z = 4, reflections collected/unique 15536/2706 (Rint = 0.1325), number of observations [>2σ(I)] 2010, parameters: 313. CCDC 1013803 contains the supplementary crystallographic data for this paper.
-
(a) N. Pietrzik, D. Schmollinger and T. Ziegler, Beilstein J. Org. Chem., 2008, 4, 30 Search PubMed;
(b) F. M. Menger and B. N. A. Mbadugha, J. Am. Chem. Soc., 2001, 123, 875 CrossRef CAS PubMed;
(c) S. Muthana, H. Yu, S. Huang and X. Chen, J. Am. Chem. Soc., 2007, 129, 11918 CrossRef CAS PubMed.
-
(a) M. Singer, M. Lopez, L. F. Bornaghi, A. Innocenti, D. Vullo, C. T. Supuran and S.-A. Poulsen, Bioorg. Med. Chem. Lett., 2009, 19, 2273 CrossRef CAS PubMed;
(b) N. Pietrzik, C. Schips and T. Ziegler, Synthesis, 2008, 519 CAS.
-
(a) L. F. Tietze, U. Bothe, U. Griesbach, M. Nakaichi, T. Hasegawa, H. Nakamurac and Y. Yamamoto, Bioorg. Med. Chem., 2009, 9, 1747 CrossRef;
(b) S. G. Gouin, L. Bultel, C. Falentin and J. Kovensky, Eur. J. Org. Chem., 2007, 1160 CrossRef CAS.
-
(a) P. Verma and B. Mukhopadhyay, Carbohydr. Res., 2009, 344, 2554 CrossRef CAS PubMed;
(b) S. Dasgupta and B. Mukhopadhyay, Eur. J. Org. Chem., 2008, 5770 CrossRef CAS.
- The prices for (S) and (R)-α,α-diphenylprolinol are 635 $ per kg and 2443 $ per kg, respectively, from Shanghai Darui Fine Chemicals.
Footnote |
† Electronic supplementary information (ESI) available: Experimental section, characterization of all compounds, and copies of 1H and 13C NMR spectra of selected compounds. CCDC 1013803. For ESI and crystallographic data in CIF or other electronic format see DOI: 10.1039/c5qo00164a |
|
This journal is © the Partner Organisations 2015 |
Click here to see how this site uses Cookies. View our privacy policy here.