DOI:
10.1039/C4RA12960A
(Paper)
RSC Adv., 2015,
5, 4693-4697
Highly efficient click reaction on water catalyzed by a ruthenium complex†
Received
23rd October 2014
, Accepted 8th December 2014
First published on 8th December 2014
Abstract
The highly efficient click reaction between terminal alkynes and azides has been achieved on water using ruthenium complex RuH2(CO)(PPh3)3 as the catalyst, and the catalyst loading was decreased to 0.2 mol% on water from 5 mol% in organic solvent. The RuH2(CO)(PPh3)3/H2O system also catalyzed the one-pot click reaction of bromides, sodium azide and alkynes; in this process, azides formed in situ and then underwent a click reaction with alkynes. In both aqueous processes, 1,4-disubstituted 1,2,3-triazoles were obtained in 50–89% yield with high regioselectivity.
Introduction
Water, which is unquestionably cheap, safe, non-toxic and readily available,1 is becoming an increasingly popular medium for organic reactions.2 Ever since Breslow adapted the Diels–Alder reaction to water,3 extraordinary advances have been made in performing organic chemistry in aqueous media.2,4 Chemists who make use of water as a solvent are often confronted with problems such as the antagonistic nature of water toward nucleophilic organic compounds5 and the limited solubility of the organic components. However, in some cases, using water as a solvent can accelerate reaction rates and enhance yield and selectivity compared to the same reaction in organic solvent,6 even when the reactants are only sparingly soluble or insoluble in water. Various factors have been proposed to explain how water can cause these enhancements. These factors include the hydrophobic effect,7 hydrogen bonding,8,9 and the method used to mix reactants in water.10 Another advantage of conducting reactions in aqueous solvent is that it facilitates the design of one-pot consecutive and multicomponent reactions (MCRs), which tend to be more environmentally friendly and atom-economical than conventional organic syntheses.11
One of the most ingenious examples of “click chemistry”12 is the copper-catalyzed Huisgen 1,3-dipolar cycloaddition of azides and alkynes (CuAAC), discovered by Meldal13 and Sharpless.14 This click reaction is the most direct route to 1,4-disubstituted 1,2,3-triazoles,15 which are applied widely across various fields, including biological science,16 synthetic organic chemistry,17 medicinal chemistry18 and material chemistry.19 Therefore, tremendous attention has been given to develop new protocols for the synthesis of various 1,2,3-triazoles.20 The ruthenium-catalyzed azide–alkyne cycloaddition reaction (RuAAC) relying on pentamethylcyclopentadienyl ruthenium chloride catalysts has been reported to give 1,5-disubstituted-1,2,3-triazole with high regioselectivity.21
In an effort to adapt the RuAAC reaction to aqueous solvent, we took advantage of a ruthenium hydride complex, RuH2(CO)(PPh3)3, which we previously showed to catalyze the click reaction in organic solvent to afford 1,4-disubstituted-1,2,3-triazole with high regioselectivity.22 Here we report that the RuH2(CO)(PPh3)3-catalyzed click reaction on water led to much higher reactivity and proceeded efficiently at catalyst loadings as low as 0.2 mol%. The synthetic usefulness of this catalytic system was further demonstrated by achieving the one-pot multicomponent cycloaddition of bromides, sodium azide and alkynes.
Results and discussion
We began our investigation of ruthenium-catalyzed cycloaddition using benzyl azide (1a) and phenylacetylene (2a) as the model substrates, and the resulting reaction mixture was analyzed by 1H NMR using PhSiMe3 as the internal standard (Table 1). Initially, 1a and 2a were heated on water at 80 °C for 2 h in the presence of RuH2(CO)(PPh3)3; this led to 100% conversion and 86% yield of 1,4-disubstituted 1,2,3-triazole 3a with 100% regioselectivity (entry 1). Encouraged by these results, we optimized the reaction by adding phase transformation catalyst (PTC), which can solubilize organic materials or form emulsions with them on water. In the presence of Bu4NBr, catalyst loading could be reduced from 5 mol% to 0.2 mol% while maintaining a 100% conversion and generating the 1,4-disubstituted 1,2,3-triazole 3a in >86% yield with 100% regioselectivity (entries 2–6). Lowering catalyst loading below 0.1 mol% led to incomplete substrate conversion (entry 7).
Table 1 RuH2(CO)(PPh3)3-catalyzed click reaction of 1a and 2a on water under various conditionsa

|
Entry |
S/C |
PTC |
Conv.b (%) |
Yieldc (%) |
Reactions were performed in sealed tubes containing 1a (0.5 mmol), 2a (1.0 mmol), PTC (0.025 mmol) and water (0.5 mL) under N2 for 2 hours, unless noted otherwise. Conversions were estimated by integrating the area under the peaks for triazole and unreacted azide in 1H NMR spectra. Based on the integrated area of the peak for unreacted azide (1a) in 1H NMR spectra, using PhSiMe3 as the internal standard. 1a (0.5 mmol) and 2a (0.6 mmol) were used. Isolated yield is shown in parentheses. |
1 |
20 |
— |
100 |
86 |
2 |
20 |
Bu4NBr |
100 |
95 |
3 |
50 |
Bu4NBr |
100 |
95 |
4 |
100 |
Bu4NBr |
100 |
94 |
5 |
200 |
Bu4NBr |
100 |
86 |
6 |
500 |
Bu4NBr |
100 |
92 |
7 |
1000 |
Bu4NBr |
69 |
63 |
8 |
500 |
CTAB |
100 |
95 |
9 |
500 |
Bu4NI |
99 |
87 |
10 |
500 |
PEG2000 |
66 |
28 |
11 |
500 |
Cyclodextrin |
64 |
47 |
12 |
500 |
Tween-80 |
54 |
37 |
13 |
500 |
— |
74 |
57 |
14d |
500 |
Bu4NBr |
100 |
94 (89)e |
Various other PTCs were then tested. Although CTAB gave 100% conversion and generated the desired 1,4-product in 95% yield, it also generated the 1,5-product as by-product in 3% yield (entry 8). Bu4NI, PEG2000, cyclodextrin or Tween-80 were inferior to Bu4NBr, giving either lower conversion or yields of products and selectivity (entries 9–12). Eliminating the PTC entirely led to poor conversion and yield (entry 13). Changing the reactant ratio (1a
:
2a) from 1
:
2 to 1
:
1.2 gave the desired 1,4-product in 89% isolated yield (entry 14).
Encouraged by the reaction efficiency, we examined its scope using the following optimized conditions: 1a
:
2a, 1
:
1.2; RuH2(CO)(PPh3)3, 0.2 mol%; Bu4NBr, 5 mol%; H2O, 0.5 mL; 80 °C; 2 h. These conditions worked well for a variety of terminal alkynes and azides (Table 2). All reactions of benzyl azide 1a with aromatic alkynes containing electron-donating or electron-withdrawing groups proceeded smoothly to afford 1,4-substituted triazole products 3b–3g in 71–88% yield. The results illustrate that the electronic properties of substituents on the benzene ring of alkynes does not appreciably affect the aqueous click reaction. Ferrocenylacetylene and alkyl alkyne were also effective in this ruthenium complex-catalyzed click reaction, producing the corresponding triazoles 3h and 3i in respective isolated yields of 80% and 82%. The reaction also proceeded with 3-ethynylpyridine, giving 3j in 62% yield, while using 2-ethynylthiophene gave 3k in 52% yield.
Table 2 RuH2(CO)(PPh3)3-catalyzed cycloaddition of various alkynes and organic azides on waterab

|
Reaction conditions: azide (0.5 mmol), alkyne (0.6 mmol), RuH2CO(PPh3)3 (0.001 mmol), Bu4NBr (0.025 mmol), 80 °C, 2 h, 0.5 mL of water. Isolated yields are reported. |
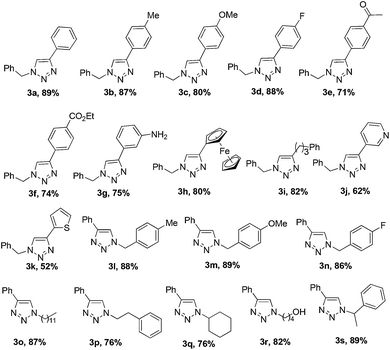 |
Next we examined the substrate scope of organic azides. Benzyl azide bearing methyl, methoxy, or fluoride groups underwent this transformation efficiently, giving products 3l–3n in 86–89% isolated yield. Alkyl organic azides also reacted efficiently, giving the desired products 3o–3q with high isolated yields of 76–87%. The hydroxyl-functionalized azide was a good reaction partner, generating triazole 3r with phenylacetylene in 82% yield. The reaction tolerated a substitution of the benzylic methylene of benzyl azide with a methyl group, leading to formation of 3s in 89% yield. This suggests that the reaction is insensitive to steric hindrance of the azide.
Based on the above results, the RuH2(CO)(PPh3)3-catalyzed click reactions on water gave yields similar to those of the corresponding reactions in organic solvent. At the same time, the use of aqueous solvent allowed us to reduce the catalyst loading from 5 mol% to 0.2 mol%.
Multicomponent reactions (MCRs) involve connecting three or more starting materials in a single synthetic operation with high atom economy and bond-forming efficiency.23 This allows the construction of high molecular diversity and complexity in a relatively rapid and straightforward manner.24 One-pot MCRs often involve shorter reaction times and higher overall yields than multi-step syntheses, thereby reducing energy and manpower requirements.25 Given the desirability of eliminating the need to store or manipulate organic azides, we envisaged a one-pot MCR involving an alkyne, sodium azide and bromide. Our plan was to generate organic azides in situ from suitable precursors, which would then undergo RuH2(CO)(PPh3)3-catalyzed azide–alkyne cycloaddition on water, thereby forming 1,2,3-triazoles. In this one-pot approach, we wished to avoid the need for interim purification of potentially unstable organic azide intermediates.
First, we screened various conditions for this one-pot MCR by taking as our model reaction the standard three-component click reaction of benzyl bromide and sodium azide with phenylacetylene. As we envisaged, the ruthenium complex RuH2(CO)(PPh3)3 was amenable to this one-pot MCR on water, displaying high activity towards this multicomponent reaction to generate 1,4-disubstituted 1,2,3-triazoles from simple substrates. After screening various catalyst loadings and PTCs, we obtained 3a in 84% isolated yield in the presence of 2 mol% catalyst after incubating the reaction for 2 h at 80 °C (Table 3).
Table 3 Optimization of conditions for the RuH2(CO)(PPh3)3-catalyzed, one-pot click reaction of benzyl bromide, sodium azide, and phenylacetylene on watera

|
Entry |
S/C |
PTC |
Conv.b (%) |
Yieldc (%) |
Reactions were performed in sealed tubes containing benzyl bromide (0.5 mmol), sodium azide (0.55 mmol), phenylacetylene (0.6 mmol), PTC (0.025 mmol) and water (0.5 mL) under N2 for 2 hours. Conversion rates were estimated by integrating the area under the peaks for triazole and unreacted benzyl bromide in 1H NMR spectra. Based on the integrated area of the peak for unreacted benzyl bromide in 1H NMR spectra, using PhSiMe3 as the internal standard. Isolated yield is shown in parentheses. |
1 |
20 |
Bu4NI |
100 |
63 |
2 |
50 |
Bu4NI |
100 |
79 (84)d |
3 |
100 |
Bu4NI |
71 |
49 |
4 |
200 |
Bu4NI |
22 |
22 |
5 |
1000 |
Bu4NI |
21 |
22 |
6 |
50 |
Bu4NBr |
75 |
54 |
7 |
50 |
— |
81 |
42 |
Then we tested the scope of this one-pot RuAAC MCR (Table 4). A broad range of aromatic alkynes containing electron-donating or electron-withdrawing groups and heterocyclic alkynes were compatible with this reaction, affording the desired products 3a–3k in 50–88% isolated yield. Various bromides including aromatic and alkyl substrates were also compatible with the reaction, providing 71–88% yields of the desired products 3l–3s. These results demonstrate that the one-pot, three-component click reactions were comparable to the click reactions of alkynes and azides, although the one-pot format required increasing the catalyst loading of RuH2(CO)(PPh3)3 to 2 mol%.
Table 4 RuH2(CO)(PPh3)3-catalyzed, one-pot click reaction of various bromides, sodium azide, and various alkynesa

|
The reaction was carried out using bromide (0.5 mmol), sodium azide (0.55 mmol), alkyne (0.6 mmol) and Bu4NI (0.025 mmol) in the presence of RuH2CO(PPh3)3 (0.01 mmol) on water (0.5 mL) at 80 °C for 2 h. |
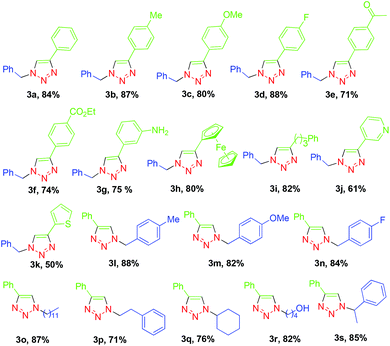 |
Conclusions
Using water as the reaction medium, we have developed a highly efficient RuH2(CO)(PPh3)3-catalyzed click reaction between terminal alkynes and organic azides to afford various 1,4-disubstituted triazoles in good to excellent yield. Catalyst loading (0.2 mol%) was much lower than that required in organic solvent (5 mol%). This catalytic system proved suitable for one-pot, three-component reactions of bromides, sodium azide, and alkynes, eliminating the need for interim purification of in situ-generated organic azides as well as significantly improving overall efficiency. We believe this protocol will offer a good option as an efficient click reaction and contribute substantially to the rapid growth in applications of click chemistry.
Experimental section
General information
All manipulations were carried out under a nitrogen atmosphere using standard Schlenk techniques, unless otherwise stated. RuH2(CO)(PPh3)3 was prepared as described.26 Freshly distilled water was used as solvent. Alkynes and other chemicals were purchased from Aldrich. Mass spectra were collected on an API QSTAR XLSystem (ESI) or GCT Premier™ Mass Spectrometer (CI). 1H and 13C{1H} NMR spectra were collected on a Bruker AV 400 MHz NMR spectrometer. 1H and 13C NMR chemical shifts were determined relative to TMS or residue of deuterium solvents.
Typical procedure for the RuH2(CO)(PPh3)3-catalyzed click reaction of various terminal alkynes and organic azides on water with low catalyst loading. To a mixture of azide (0.5 mmol), alkyne (0.6 mmol), and H2O (0.5 mL) were added catalyst RuH2(CO)(PPh3)3 (0.001 mmol) and phase transformation catalyst (PTC) Bu4NBr (0.025 mmol). The resulting solution was stirred at 80 °C for 2 h. Then the reaction mixture was extracted three times with 1 mL CHCl3. The organic phases were combined, the solvent was evaporated under reduced pressure, and the residue was subjected to flash column chromatography on silica gel to afford the desired product. All the compounds reported here are known, except for 3i and 3r (see ESI†).
Typical procedure for RuH2(CO)(PPh3)3-catalyzed one-pot click reaction of benzyl bromide, sodium azide, and phenylacetylene on water. To a mixture of bromide (0.5 mmol), sodium azide (0.55 mmol), alkyne (0.6 mmol) and H2O (0.5 mL) were added catalyst RuH2(CO)(PPh3)3 (0.01 mmol) and PTC Bu4NI (0.025 mmol). The resulting solution was stirred at 80 °C for 2 h. Then the reaction mixture was extracted three times with 1 mL CHCl3. The organic phases were combined, the solvent was evaporated under reduced pressure, and the residue was subjected to flash column chromatography on silica gel to afford the desired product. All the compounds reported here are known (see ESI†), except for 3i and 3r.
1-Benzyl-4-(3-phenyl-propyl)-1H-1,2,3-triazole (3i). Mp: 60–62.5 °C; 1H NMR (400 MHz, CDCl3, 25 °C) δ 7.34–7.37 (m, 3H), 7.24–7.26 (m, 4H), 7.15–7.19 (m, 4H), 5.49 (s, 2H), 2.64–2.74 (dt, 4H), 1.94–2.02 (m, 2H); 13C NMR (100.6 MHz, CDCl3, 25 °C) δ 148.4, 141.9, 135.0, 129.1, 128.6, 128.5, 128.4, 128.0, 125.9, 120.7, 54.0, 35.4, 31.3, 25.3; HRMS (ESI, TOF) calcd for C18H20N3 [M + H]+ 278.1562, found 278.1567.
4-(4-Phenyl-1,2,3-triazol-1-yl)-butan-1-ol (3r). Mp: 88–90 °C; 1H NMR (400 MHz, CDCl3, 25 °C) δ 7.82 (m, 2H), 7.78 (s, 1H), 7.43 (t, J = 7.4 Hz, 2H), 7.34 (t, J = 7.4 Hz, 1H), 4.47 (t, J = 7.1 Hz, 2H), 3.71 (t, J = 6.1 Hz, 2H), 2.04–2.12 (m, 2H), 1.60–1.66 (m, 3H); 13C NMR (100.6 MHz, CDCl3, 25 °C) δ 147.8, 130.6, 128.9, 128.2, 125.7, 119.6, 61.9, 50.2, 29.3, 27.0; HRMS (ESI, TOF) calcd for C12H16N3O [M + H]+ 218.1288, found 218.1287.
Acknowledgements
This work was supported by NSFC/China, NCET (NCET-13-0798), the Basic Research Program of the Shanghai Committee of Sci. & Tech. (Project no. 13NM1400802), and the Fundamental Research Funds for the Central Universities.
Notes and references
- S. Narayan, J. Muldoon, M. G. Finn, V. V. Fokin, H. C. Kolb and K. B. Sharpless, Angew. Chem., Int. Ed., 2005, 44, 3275 CrossRef CAS PubMed.
-
(a) C.-J. Li, Chem. Rev., 1993, 93, 2023 CrossRef CAS;
(b) J. P. Genet and M. Savignac, J. Organomet. Chem., 1999, 576, 305 CrossRef;
(c) U. M. Lindström, Chem. Rev., 2002, 102, 2751 CrossRef PubMed;
(d) S. Kobayashi and K. Manabe, Acc. Chem. Res., 2002, 35, 209 CrossRef CAS PubMed;
(e) C.-J. Li, Chem. Rev., 2005, 105, 3095 CrossRef CAS PubMed;
(f) C.-J. Li and L. Chen, Chem. Soc. Rev., 2006, 35, 68 RSC;
(g) M. Raj and V. K. Singh, Chem. Commun., 2009, 6687 RSC;
(h) M.-O. Simon and C.-J. Li, Chem. Soc. Rev., 2012, 41, 1415 RSC;
(i) R. N. Butler and A. G. Coyne, Chem. Rev., 2010, 110, 6302 CrossRef CAS PubMed.
-
(a) R. Breslow, Acc. Chem. Res., 1991, 24, 159 CrossRef CAS;
(b) R. Breslow, Acc. Chem. Res., 2004, 37, 471 CrossRef CAS PubMed.
-
(a) J. E. Klijn and J. B. F. N. Engberts, Nature, 2005, 435, 746 CrossRef CAS PubMed;
(b) Y. Hayashi, Angew. Chem., Int. Ed., 2006, 45, 8103 CrossRef CAS PubMed;
(c) J. B. F. N. Engberts and M. J. Blandamer, Chem. Commun., 2001, 1701 RSC.
- P. G. Cozzi and L. Zoli, Angew. Chem., Int. Ed., 2005, 47, 4162 CrossRef PubMed ; and the references therein.
-
(a) M. C. Pirrung, Chem.–Eur. J., 2006, 12, 1312 CrossRef CAS PubMed;
(b) S. Otto and J. B. F. N. Engberts, Org. Biomol. Chem., 2003, 1, 2809 RSC;
(c) K. Aplander, R. Ding, U. M. Lindström, J. Wennerberg and S. Schultz, Angew. Chem., Int. Ed., 2007, 46, 4543 CrossRef CAS PubMed;
(d) M.-O. Simon and C.-J. Li, Chem. Soc. Rev., 2012, 41, 1415 RSC;
(e) P. N. Liu, J. G. Deng, Y. Q. Tu and S. H. Wang, Chem. Commun., 2004, 2070 RSC;
(f) P.-N. Liu, P.-M. Gu, J.-G. Deng, Y.-Q. Tu and Y.-P. Ma, Eur. J. Org. Chem., 2005, 3221 CrossRef CAS.
- J. B. F. N. Engberts and M. J. Blandamer, Chem. Commun., 2001, 1701 RSC For selected examples, see:
(a) E. Coutouli-Argyropoulou, P. Sarridis and P. Gkizis, Green Chem., 2009, 11, 1906 RSC;
(b) S. Tiwari and A. Kumar, Chem. Commun., 2008, 4445 RSC;
(c) U. M. Lindström and F. Andersson, Angew. Chem., Int. Ed., 2006, 45, 548 CrossRef PubMed;
(d) J. R. Nitschke, M. Hutin and G. Bernaardinelli, Angew. Chem., Int. Ed., 2004, 43, 6724 CrossRef CAS PubMed;
(e) R. Breslow, Acc. Chem. Res., 2004, 37, 471 CrossRef CAS PubMed;
(f) A. Ben-Naim, Hydrophobic Interactions, Plenum Press, New York, 1980 Search PubMed;
(g) C. Tanford, The Hydrophobic Effect, Wiley, New York, 2nd edn, 1980 Search PubMed.
- A. Chanda and V. V. Fokin, Chem. Rev., 2009, 109, 725 CrossRef CAS PubMed.
- For selected examples, see:
(a) C. Santi, B. Battistelli, L. Testaferri and M. Tiecco, Green Chem., 2012, 14, 1277 RSC;
(b) X.-P. Fu, L. Liu, D. Wang, Y.-J. Chen and C.-J. Li, Green Chem., 2011, 13, 549 RSC;
(c) Y. Jung and R. A. Marcus, J. Am. Chem. Soc., 2007, 129, 5492 CrossRef CAS PubMed.
- M. C. Pirrund, K. D. Sarma and J. Wang, J. Org. Chem., 2008, 73, 8723 CrossRef PubMed.
-
(a) K. Kumaravel and G. Vasuki, Curr. Org. Chem., 2009, 13, 1820 CrossRef CAS;
(b) V. Estévez, M. Villacampa and J. C. Menéndez, Chem. Soc. Rev., 2010, 39, 4402 RSC;
(c) Y. Gu, Green Chem., 2012, 14, 2091 RSC.
- H. C. Kolb, M. G. Finn and K. B. Sharpless, Angew. Chem., Int. Ed., 2001, 40, 2004 CrossRef CAS.
- C. W. Tornøe, C. Christensen and M. Meldal, J. Org. Chem., 2002, 67, 3057 CrossRef PubMed.
- V. V. Rostovtsev, L. G. Green, V. V. Fokin and K. B. Sharpless, Angew. Chem., Int. Ed., 2002, 41, 2596 CrossRef CAS.
-
(a) 1,3-Dipolar cycloaddition chemistry, ed. A. Padwa, Wiley-VCH, New York, 1984 Search PubMed;
(b) Synthetic applications of 1,3 dipolar cycloaddition chemistry toward heterocycles and natural products, ed. A. Padwa and W. H. Pearson, Wiley-VCH, New York, 2002 Search PubMed;
(c) W. Lwowski, in 1,3-Dipolar Cycloaddition Chemistry, ed. A. Padwa, Wiley-VCH, New York, 1984, vol. 1, p. 559 Search PubMed.
- Selected examples, see:
(a) M. J. Genin, D. A. Allwine, D. J. Anderson, M. R. Barbachyn, D. E. Emmert, S. A. Garmon, D. R. Graber, K. C. Grega, J. B. Hester, D. K. Hutchinson, J. Morris, R. J. Reischer, C. W. Ford, G. E. Zurenko, J. C. Hamel, R. D. Schaadt, D. Stapert and B. H. Yagi, J. Med. Chem., 2000, 43, 953 CrossRef CAS PubMed;
(b) R. Alvarez, S. Velazquez, A. San-Felix, S. Aquaro, E. D. Clercq, C. F. Perno, A. Karlsson, J. Balzarini and M. J. Camarasa, J. Med. Chem., 1994, 37, 4185 CrossRef CAS.
- Selected examples, see:
(a) S. Wacharasindhu, S. Bardhan, Z.-K. Wan, K. Tabei and T. S. Mansour, J. Am. Chem. Soc., 2009, 131, 4174 CrossRef CAS PubMed;
(b) Y. X. Liu, W. M. Yan, Y. F. Chen, J. L. Petersen and X. D. Shi, Org. Lett., 2008, 10, 5389 CrossRef CAS PubMed;
(c) A. R. Katritzky, S. Bobrov, K. Kirichenko, Y. Ji and P. J. Steel, J. Org. Chem., 2003, 68, 5713 CrossRef CAS PubMed.
-
(a) R. Manetsch, A. Krasiski, Z. Radi, J. Raushel, P. Taylor, K. B. Sharpless and H. C. Kolb, J. Am. Chem. Soc., 2004, 126, 12809 CrossRef CAS PubMed;
(b) M. Whiting, J. Muldoon, Y. C. Lin, S. M. Silverman, W. Lindstrom, A. J. Olson, H. C. Kolb, M. G. Finn, K. B. Sharpless, J. H. Elder and V. V. Fokin, Angew. Chem., Int. Ed., 2006, 45, 1435 CrossRef CAS PubMed;
(c) J. Wang, G. Sui, V. P. Mocharla, R. J. Lin, M. E. Phelps, H. C. Kolb and H.-R. Tseng, Angew. Chem., Int. Ed., 2006, 45, 5276 CrossRef CAS PubMed;
(d) G. C. Tron, T. Pirali, R. A. Billington, P. L. Canonico, G. Sorba and A. A. Genazzani, Med. Res. Rev., 2008, 28, 278 CrossRef CAS PubMed.
- Selected examples, see:
(a) H. Nandivada, X. W. Jiang and J. Lahann, Adv. Mater., 2007, 19, 2197 CrossRef CAS;
(b) C. F. Ye, G. L. Gard, R. W. Winter, R. G. Syvret, B. Twamley and J. M. Shreeve, Org. Lett., 2007, 9, 3841 CrossRef CAS PubMed;
(c) P. Wu, A. K. Feldman, A. K. Nugent, C. J. Hawker, A. Scheel, B. Voit, J. Pyun, J. M. J. Fréchet, K. B. Sharpless and V. V. Fokin, Angew. Chem., Int. Ed., 2004, 43, 3928 CrossRef CAS PubMed;
(d) V. Aucagne, K. D. Hänni, D. A. Leigh, P. J. Lusby and D. B. Walker, J. Am. Chem. Soc., 2006, 128, 2186 CrossRef CAS PubMed.
-
(a) M. Meldal and C. W. Tornøe, Chem. Rev., 2008, 108, 2952 CrossRef CAS PubMed;
(b) B. Dervaux and F. E. D. Prez, Chem. Sci., 2012, 3, 959 RSC;
(c) N. V. Sokolova and V. G. Nenajdenko, RSC Adv., 2013, 3, 16212 RSC.
-
(a) L. Zhang, X. Chen, P. Xue, H. H. Y. Sun, I. D. Williams, K. B. Sharpless, V. V. Fokin and G. Jia, J. Am. Chem. Soc., 2005, 127, 15998 CrossRef CAS PubMed;
(b) B. C. Boren, S. Narayan, L. K. Rasmussen, L. Zhang, H. Zhao, Z. Lin, G. Jia and V. V. Fokin, J. Am. Chem. Soc., 2008, 130, 8923 CrossRef CAS PubMed;
(c) B. C. Boren and V. V. Fokin, Org. Lett., 2007, 9, 5337 CrossRef PubMed;
(d) S. Oppilliart, G. Mousseau, L. Zhang, G. Jia, P. Thuéry, B. Rousseau and J. C. Cintrat, Tetrahedron, 2007, 63, 8094 CrossRef CAS PubMed;
(e) Other studies on RuAAC, see: D. R. Hou, T. C. Kuan, Y. K. Li, R. Lee and K. W. Huang, Tetrahedron, 2010, 66, 9415 CrossRef CAS PubMed;
(f) A. H. Yap and S. M. Weinreb, Tetrahedron Lett., 2006, 47, 3035 CrossRef CAS PubMed;
(g) M. M. Majireck and S. M. Weinreb, J. Org. Chem., 2006, 71, 8680 CrossRef CAS PubMed;
(h) J. R. Johansson, P. Lincoln, B. Norden and N. Kann, J. Org. Chem., 2011, 76, 2355 CrossRef CAS PubMed.
-
(a) P. N. Liu, H. X. Siyang, L. Zhang, S. K. S. Tse and G. Jia, J. Org. Chem., 2012, 77, 5844 CrossRef CAS PubMed;
(b) P. N. Liu, J. Li, F. H. Su, K. D. Ju, L. Zhang, C. Shi, H. H. Y. Sung, I. D. Williams, V. V. Fokin, Z. Lin and G. Jia, Organometallics, 2012, 31, 4904 CrossRef CAS.
-
(a) R. V. A. Orru and M. de Greef, Synthesis, 2003, 1471 CrossRef CAS PubMed;
(b) D. Tejedor, D. González-Cruz, A. Santos-Expósito, J. J. Marrero-Tellado, P. de Armas and F. García-Tellado, Chem.–Eur. J., 2005, 11, 3502 CrossRef CAS PubMed;
(c) A. Dömling, Chem. Rev., 2006, 106, 17 CrossRef PubMed;
(d) B. B. Touré and D. G. Hall, Chem. Rev., 2009, 109, 4439 CrossRef PubMed.
-
(a) C. Hulme and V. Gore, Curr. Med. Chem., 2003, 10, 51 CrossRef CAS;
(b) For a monograph on MCRs, see; Multicomponent Reactions, ed. J. Zhu and H. Bienaymé, Wiley-VCH, 2005 Search PubMed.
-
(a) J. D. Sunderhaus and S. F. Martin, Chem.–Eur. J., 2009, 15, 1300 CrossRef CAS PubMed;
(b) B. Ganem, Acc. Chem. Res., 2009, 42, 463 CrossRef CAS PubMed;
(c) N. Isambert and R. Lavilla, Chem.–Eur. J., 2008, 14, 8444 CrossRef CAS PubMed;
(d) D. M. D'Souza and T. J. J. Müller, Chem. Soc. Rev., 2007, 36, 1095 RSC.
- N. Ahmad, J. J. Levison, S. D. Robinson, M. F. Uttley, E. R. Wonchoba and G. W. Parshall, Inorg. Synth., 1974, 15, 45 CAS.
Footnote |
† Electronic supplementary information (ESI) available: Analytical data for all known products (melting point, 1H and 13C NMR, MS), copies of 1H NMR spectra of all products, copies of 13C NMR spectra of 3i and 3r. See DOI: 10.1039/c4ra12960a |
|
This journal is © The Royal Society of Chemistry 2015 |
Click here to see how this site uses Cookies. View our privacy policy here.