DOI:
10.1039/C4RA13752C
(Paper)
RSC Adv., 2015,
5, 5032-5037
Efficient synthesis of quinazoline-2,4(1H,3H)-diones from CO2 catalyzed by N-heterocyclic carbene at atmospheric pressure
Received
4th November 2014
, Accepted 11th December 2014
First published on 11th December 2014
Abstract
Under atmospheric pressure, quinazoline-2,4(1H,3H)-diones were obtained from the reaction of 2-aminobenzonitriles with carbon dioxide (0.1 MPa) with a catalytic amount of N-heterocyclic carbene in DMSO. It was found that various electron-donating and electron-withdrawing groups such as –OMe, –F, –Cl, –Br, –CH3, –CF3 and –CN were well tolerated to give the products in almost quantitative yields.
Introduction
Efficient transformation of CO2, one of the major green house gases, into value-added chemicals and materials has gained much interest from the viewpoints of sustainable and green chemistry. Carbon dioxide (CO2) is a renewable, abundant, low cost, easily available and nontoxic source of a functional carbon unit.1 Recently, utilization of CO2 as a substrate to prepare a variety of organic compounds, such as cyclic carbonates,2 dimethyl carbonate,3 polycarbonates,4 urethanes,5 formic acids,6 methanol,7 ureas,8 esters9 and others,10 has been reported.
Quinazoline-2,4(1H,3H)-diones and their derivatives, as an important heterocyclic compounds, have been widely applied in the pharmaceutical and biotechnology industries.11 Many strategies for the synthesis of this class of compounds have been developed;12 however, as an environmentally benign process with 100% atom efficiency, the transformation of CO2 into quinazoline-2,4(1H,3H)-diones with 2-aminobenzonitriles has received much attention and a wide range of catalysts have been explored for this transformation, such as DBU, Cs2CO3, MgO–ZrO2, [Bmim]OH, [Bmim]OAc, guanidine and MCM-41 etc.13 However, most of synthetic protocols reported have many drawbacks such as long reaction times, high pressures of CO2, expensive catalysts, high reaction temperature, and poor generality, so development of an efficient and general approach for this transformation under mild conditions with cheap and readily available catalyst is still highly desired.
In the recent years, N-heterocyclic carbenes (NHCs) have been successfully applied in catalysis and in coordination chemistry.14 We have developed an interest in exploring the utility of N-heterocyclic carbenes in CO2 fixation, and observed that NHC is a highly active catalyst for the activation of CO2 to synthesize cyclic carbonates with epoxides due to its strong σ-donor character to capture CO2.15 Herein, we report that NHC as a cheap, readily available and highly active catalyst for the efficient synthesis of quinazoline-2,4(1H,3H)-diones from CO2 and 2-aminobenzonitriles under mild reaction conditions. The excellent yield, wide substrate scope and various functional group tolerance were found in this catalytic protocol.
Results and discussion
To test the feasibility of our envisaged approach, we initially examined the reaction of 2-amino-4-chlorobenzonitrile with CO2 catalyzed by NHC generated from imidazolium chloride (e) in the presence of the base K2CO3 in DMSO. When the reaction was carried out at 120 °C and atmospheric pressure of CO2 (0.1 MPa) for 10 hours, 65% of 7-chloroquinazoline-2,4(1H,3H)-dione was isolated (Table 1, entry 5). To investigate how the steric and electronic properties of NHCs affect the results of the syntheses of quinazoline derivatives, the different types of NHCs (a–h) were chosen and a survey of their catalytic activities was performed under the identical conditions (Table 1, entries 1–8). The results showed the most effective catalyst was found to be a leading to the highest 93% yield of the product, whereas, the least effective catalysts are f and g leading to the lowest 37–39% yield. The reactivity of d is moderate and comparable to that of e (∼65%). Furthermore, the reactivity of b and c is very similar, but slightly lower (∼50%) than that of d and e. The results suggested that combination the steric hindrance and electron-donating ability plays a role in the reactivity, however, the real reasons behind the difference are not very obvious at this time.
Table 1 Synthesis of 7-chloroquinazoline-2,4(1H,3H)-dione under different reaction conditions
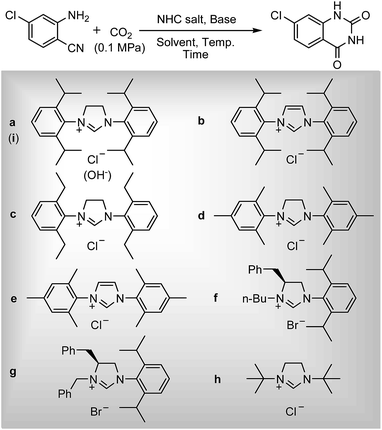
|
Entrya |
NHC salt |
Base |
Solvent |
Temp. (°C) |
Time (h) |
Yieldb (%) |
Reaction conditions: 2-amino-4-chlorobenzonitrile (2 mmol), NHC salt (0.4 mmol), base (2 mmol), CO2 (0.1 MPa) and solvent (3 mL). Isolated yield on average of two runs. |
1 |
a |
K2CO3 |
DMSO |
120 |
10 |
93 |
2 |
b |
K2CO3 |
DMSO |
120 |
10 |
53 |
3 |
c |
K2CO3 |
DMSO |
120 |
10 |
50 |
4 |
d |
K2CO3 |
DMSO |
120 |
10 |
62 |
5 |
e |
K2CO3 |
DMSO |
120 |
10 |
65 |
6 |
f |
K2CO3 |
DMSO |
120 |
10 |
37 |
7 |
g |
K2CO3 |
DMSO |
120 |
10 |
39 |
8 |
h |
K2CO3 |
DMSO |
120 |
10 |
59 |
9 |
a |
KOH |
DMSO |
120 |
10 |
40 |
10 |
a |
K3PO4 |
DMSO |
120 |
10 |
30 |
11 |
a |
KOtBu |
DMSO |
120 |
10 |
52 |
12 |
a |
— |
DMSO |
120 |
10 |
0 |
13 |
i |
— |
DMSO |
120 |
10 |
0 |
14 |
— |
K2CO3 |
DMSO |
120 |
10 |
28 |
15 |
a |
K2CO3 |
DMF |
120 |
10 |
45 |
16 |
a |
K2CO3 |
Dioxane |
120 |
10 |
10 |
17 |
a |
K2CO3 |
CH3CN |
120 |
10 |
3 |
18 |
a |
K2CO3 |
H2O |
120 |
10 |
3 |
19 |
a |
K2CO3 |
DMF |
120 |
8 |
37 |
20 |
a |
K2CO3 |
DMSO |
40 |
10 |
5 |
21 |
a |
K2CO3 |
DMSO |
60 |
10 |
5 |
22 |
a |
K2CO3 |
DMSO |
80 |
10 |
10 |
23 |
a |
K2CO3 |
DMSO |
100 |
10 |
41 |
24 |
a |
K2CO3 |
DMSO |
130 |
10 |
80 |
25 |
a |
K2CO3 |
DMSO |
120 |
4 |
77 |
26 |
a |
K2CO3 |
DMSO |
120 |
6 |
89 |
27 |
a |
K2CO3 |
DMSO |
120 |
8 |
93 |
The high activity of a prompted us to optimize the reaction further using this catalyst. It is known that the base and solvent are very important parameters determining the reaction efficiency. Firstly, the effect of base was investigated using K2CO3, KOH, K3PO4, and KOtBu (Table 1, entries 1 and 9–11). The results revealed that only 52% of product was obtained by using stronger base potassium tert-butoxide (entry 11), whereas the reaction of using weaker base potassium carbonate occurred in the highest 93% yield (entry 1). The lowest yield of 30% was obtained using K3PO4 as base (entry 10) and slightly higher yield (40%) was observed for using KOH (entry 9).
It is known that the reaction could be catalyzed by ionic liquid, such as 1-butyl-3-methylimidazolium hydroxide ([Bmim]OH) as a catalyst under high pressure of CO2 (3 MPa)13l or [Bmim]OAc under atmospheric pressure.13g To understand if the reaction would be catalyzed by imidazolium chloride or imidazolium hydroxide resulting from anion exchange, the hydroxide analogue i of imidazolium chloride a was prepared. The control reactions were run and the results revealed that no product was observed for both of them without base (Table 1, entries 12 and 13), implying that NHC generated in situ is an active specie in the reaction, not the precursor imidazolium salt. In addition, the activity of K2CO3 in the absence of imidazolium salt was checked as the base used as the catalyst has been reported for the process. The result was illustrated in Table 1 entry 14, showing only 28% of product isolated. The effect of solvents on the reaction was also investigated (Table 1, entries 1 and 15–18). The reaction in DMF gave relatively low yield (45%) comparing to that in DMSO (93%), whereas dioxane, CH3CN, and water all led to even worse yields (below 10%). To clarify that the lower yield in DMF was not caused by the longer reaction time (10 h), the controlled reaction was carried out in DMF for 8 h (Table 1, entry 19). The result showed shorter reaction time leading to lower yield (37%). Furthermore, the effect of reaction temperature and time on the reaction was investigated (Table 1, entries 20–27). The choice of the reaction temperature is very crucial, both lower and higher temperatures would lead to lower yield, and 120 °C is the optimal reaction temperature. The lower yield caused by higher temperature is probably due to the decomposing of NHC–CO2 adduct, which is known in literature.16 By studying the influence of reaction time on the reaction, it was found the reaction actually completed within 8 h when the reaction was carried out at 120 °C under 0.1 MPa CO2 (Table 1, entries 25–27). Hence in the following studies, all reactions were carried with 20 mol% of a as catalyst, K2CO3 as base, and DMSO as a solvent at 120 °C and 0.1 MPa CO2 pressure for 8 h.
To further assess the generality and scope of the reaction, various substituted 2-aminobenzonitrile were chosen and examined in the synthesis of quinozoline-2,4(1H,3H)-diones (Table 2). The reactions of 2-aminobenzonitriles bearing the electron-donating group such as OMe group (Table 2, entry 4) went smoothly with the yield of up to 99%. In addition, the reaction of 2-aminobenzonitriles bearing the electron-withdrawing group such as CF3 (Table 2, entry 8) went well with yields of up to 98%. Various groups, such as –OMe, –F, –Cl, –Br, –CH3, –CF3 and –CN, were well tolerated to give the product in excellent yields. The reactions were very clean and the workup and purification is very simple. The pure quinazoline products were obtained by simply filtration in almost quantitative yields.
Table 2 Synthesis of quinazoling-2,4(1H,3H)diones compounds
From the established mechanism for the reaction of 2-aminobenzonitriles with CO2 and our current experimental results, the proposed mechanism is shown in Scheme 1. We suggest that NHC is the catalytic active species, NHC generated in situ directly reacts with CO2 to form the NHC–CO2 adduct. Then the nucleophilic addition of zwitterionic adduct to the benzonitrile to generate intermediate A. Subsequent intramolecular cyclization regenerates NHC. Finally, quinazoling-2,4(1H,3H)diones is produced via a series of proton transfer, ring opening, and intramolecular nucleophilic addition.
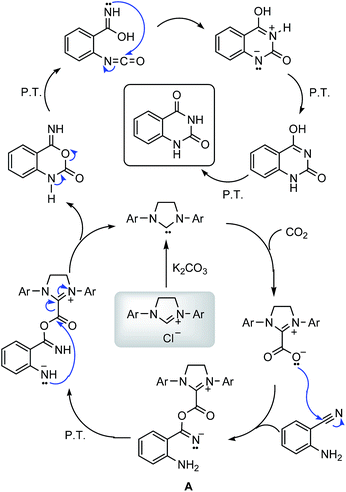 |
| Scheme 1 Plausible reaction mechanism for the reaction of 2-aminobenzonitriles with CO2. | |
Conclusions
In summary, we have developed an efficient method for the reaction of CO2 with 2-aminobenzomitriels to synthesize quinazoling-2,4(1H,3H)diones. In the present work, the fixation of CO2 can be carried out using readily available NHC as catalyst under atmospheric pressure of CO2 (0.1 MPa). High conversions and excellent yield were achieved using this catalytic system under mild conditions, and the workup and purification was very simple. Various electron-donating and electron-withdrawing groups such as –OMe, –F, –Cl, –Br, –CH3, –CF3 and –CN were well tolerated in the reaction.
Experimental section
General information
Imidazolinium bromides f and g were prepared using literature procedures.17 Imidazolinium hydroxide i was prepared by modified procedure.18 All other chemicals were commercially available and used as received unless otherwise stated. DMSO and acetonitrile were distilled from calcium hydride, and dioxane was distilled from sodium benzophenone ketyl prior to use. DMF was stirred over anhydrous MgSO4 overnight, filtered, and then distilled over 4 Å molecular sieves under nitrogen atmosphere. CO2 with a purity of 99.95% was used. 1H and 13C spectra were recorded on a Bruker AV 400 MHz spectrometer at room temperature and referenced to the residual signals of the solvent. Melting points were detected by microscope melting point apparatus. IR spectra were recorded on KBr pellets on a FTIR-Tensor 27 spectrometer.
Synthesis of 1,3-bis[2,6-bis(1-methylethyl)phenyl]-4,5-dihydro-1H-imidazolium hydroxide (i)
To 15 mL of DCM in a 25 mL round bottom flask, was added imidazolinium chloride a (0.854 g, 2 mmol) and KOH (0.56 g, 10 mmol). The reaction was stirred at room temperature for 24 h, after which time the solids were removed by filtration. The filtrate was concentrated and the resulting crude was purified by chromatography to give 0.752 g of the product in 92% yield as white solid. (PE
:
EtOAc = 5
:
1). IR (KBr) 3647, 3379, 2966, 2869, 2359, 1661, 1590, 1487, 1461, 1403, 1384, 1344, 1267, 1120, 1097, 1054, 992, 930, 861, 843, 811, 799, 758, 676, 621 cm−1; 1H NMR (400 MHz, CDCl3) δ = 8.09 (s, 1H), 7.37 (t, J = 7.7 Hz, 1H), 7.22 (d, J = 7.8 Hz, 2H), 7.07–7.00 (m, 3H), 3.87 (t, J = 7.3 Hz, 2H), 3.22–3.01 (m, 6H), 1.25–1.13 (m, 24H); 13C NMR (100 MHz, CDCl3) δ = 164.0, 147.7, 142.4, 135.6, 129.6, 124.6, 123.9, 123.6, 49.0, 48.8, 28.4, 27.9, 25.5, 24.3, 23.6.
General procedure of synthesis of quinazoline
In a typical run, a 10 mL of high pressure autoclave with a stir bar was charged with 2-aminobenzonitrile (2 mmol, 305 mg), a (0.4 mmol, 171 mg), K2CO3 (2 mmol, 276 mg) in glove box, and the capped autoclave was move out of glove box. DMSO (3 mL) was injected into the autoclave, and it was connected to a CO2 container. The autoclave was purged with CO2 for 3 times and its pressure was adjusted to 0.1 MPa. After the autoclave was heated at 120 °C for 8 h with stirring, it was cool to room temperature. The reaction mixture was poured into the water (10 mL), and the solid was collected by filtration and rinsed with water and ether, then dried under vacuum to give the pure product.
Quinazoline-2,4(1H,3H)-dione (Table 2, entry 1). White solid, m.p. > 300 °C, IR (KBr) 3252, 3054, 2845, 1701, 1670, 1617, 1442, 754 cm−1; 1H NMR (400 MHz, DMSO-d6) δ = 11.30 (s, 1H, NH), 11.16 (s, 1H, NH), 7.88 (d, J = 7.6 Hz, 1H, CH), 7.63 (t, J = 8.0 Hz, 1H, CH), 7.17 (t, J = 8.4 Hz, 2H, 2CH); 13C NMR (100 MHz, DMSO-d6) δ = 162.8, 150.3, 140.9, 134.9, 126.9, 122.3, 115.3, 114.3.
7-Chloroquinazoline-2,4(1H,3H)-dione (Table 2, entry 2). Light yellow solid, m.p. > 300 °C, IR (KBr) 3305, 3046, 1744, 1682, 1616, 1430, 1283, 862 cm−1; 1H NMR (400 MHz, DMSO-d6) δ = 11.41 (s, 1H, NH), 11.26 (s, 1H, NH), 7.88 (d, J = 8.4 Hz, 1H, CH), 7.22 (d, J = 8.4 Hz, 1H, CH), 7.18 (d, J = 1.6 Hz, 1H, CH); 13C NMR (100 MHz, DMSO-d6) δ = 162.1, 150.2, 141.9, 139.3, 129.0, 122.4, 114.7, 113.3.
6-Chloroquinazoline-2,4(1H,3H)-dione (Table 2, entry 3). Light yellow solid, m.p. > 300 °C, IR (KBr) 3199, 3056, 1712, 1668, 1482, 1428, 1283, 876 cm−1; 1H NMR (400 MHz, DMSO-d6) δ = 11.43 (s, 1H, NH), 11.27 (s, 1H, NH), 7.79 (s, 1H, CH), 7.66 (d, J = 8.8 Hz, 1H, CH), 7.17 (d, J = 8.4 Hz, 1H, CH); 13C NMR (100 MHz, DMSO-d6) δ = 162.0, 150.2, 139.8, 134.9, 126.5, 126.0, 117.6, 115.8.
6,7-Dimethoxyquinazoline-2,4(1H,3H)-dione (Table 2, entry 4). Light yellow solid, m.p. > 300 °C, IR (KBr) 3470, 3378, 3294, 1708, 1652, 1624, 1466, 1436, 1264, 1098 cm−1; 1H NMR (400 MHz, DMSO-d6) δ = 11.09 (s, 1H, NH), 10.92 (s, 1H, NH), 7.25 (s, 1H, CH), 6.67 (s, 1H, CH), 3.82 (s, 3H, CH3), 3.78 (s, 3H, CH3); 13C NMR (100 MHz, DMSO-d6) δ = 162.4, 154.9, 150.4, 145.0, 136.5, 107.2, 106.2, 97.8, 55.8, 55.7.
6-Fluoroquinazoline-2,4(1H,3H)-dione (Table 2, entry 5). Light yellow solid, m.p. > 300 °C, IR (KBr) 3198, 3058, 1714, 1676, 1634, 1500, 1438, 1288, 884 cm−1; 1H NMR (400 MHz, DMSO-d6) δ = 11.43 (s, 1H, NH), 11.22 (s, 1H, NH), 7.53–7.59 (m, 2H, 2CH), 7.18–7.21 (m, 1H, CH); 13C NMR (100 MHz, DMSO-d6) δ = 165.9 (d, J = 249.4 Hz), 162.0, 150.4, 143.0 (d, J = 13.1 Hz), 130.2 (d, J = 11.2 Hz), 111.4 (d, J = 1.8 Hz), 110.4 (d, J = 23.0 Hz), 101.6 (d, J = 26.0 Hz).
7-Fluoroquinazoline-2,4(1H,3H)-dione (Table 2, entry 6). White solid, m.p. > 300 °C, 1H NMR (400 MHz, DMSO-d6) δ = 11.38 (s, 1H, NH), 11.28 (s, 1H, NH), 7.93-7.96 (m, 1H, CH), 7.03 (t, J = 8.8 Hz, 1H, CH), 6.89 (dd, J = 1.6 Hz, J = 4.8 Hz, 1H, CH); 13C NMR (100 MHz, DMSO-d6) δ = 162.2 (d, J = 3.0 Hz), 157.3 (d, J = 238.2 Hz), 150.1, 137.6 (d, J = 1.4 Hz), 123.0 (d, J = 24.1 Hz), 117.6 (d, J = 7.8 Hz), 115.4 (d, J = 7.6 Hz), 112.0 (d, J = 23.6 Hz).
6-Bromoquinazoline-2,4(1H,3H)-dione (Table 2, entry 7). Yellow solid, m.p. > 300 °C; 1H NMR (400 MHz, DMSO-d6) δ = 11.45 (s, 1H, NH), 11.30 (s, 1H, NH), 7.93 (s, 1H, CH), 7.79 (dd, J = 2.0 Hz, J = 8.8 Hz, 1H, CH), 7.13 (d, J = 8.4 Hz, 1H, CH); 13C NMR (100 MHz, DMSO-d6) δ = 161.8, 150.1, 140.1, 137.5, 128.9, 117.8, 116.2, 113.9.
6-(Trifluoromethyl)quinazoline-2,4(1H,3H)-dione (Table 2, entry 8). White solid, m.p. > 300 °C, IR (KBr): 3311, 3187, 3066, 2897, 2832, 1702, 1634, 1508, 1489, 1441, 1378, 1319, 1289, 1253, 1145, 1112, 1069, 1015, 930, 842, 785, 755, 693, 652, 609, 511 cm−1; 1H NMR (400 MHz, DMSO-d6) δ = 11.56 (s, 1H, NH), 11.52 (s, 1H, NH), 8.08 (s, 1H, CH), 7.95 (dd, J = 8.6 Hz, J = 2.0 Hz, 1H, CH), 7.31 (d, J = 8.6 Hz, 1H, CH); 13C NMR (100 MHz, DMSO-d6) δ = 162.0, 150.2, 143.8, 131.3 (d, J = 3.3 Hz), 124.2 (d, J = 4.1 Hz), 122.6 (d, J = 33 Hz), 122.5 (m), 116.7, 114.5.
2,4-Dioxo-1,2,3,4-tetrahydroquinazoline-6-carbonitrile (Table 2, entry 9). Light yellow solid, m.p. > 300 °C, IR (KBr): 3224, 3082, 2349, 2229, 1700, 1623, 1505, 1456, 1384, 1340, 1294, 1238, 1193, 1157, 1099, 1018, 943, 861, 806, 723, 670 cm−1; 1H NMR (400 MHz, DMSO-d6) δ = 11.58 (s, 1H, NH), 11.57 (s, 1H, NH), 8.24 (d, J = 2.0 Hz, 1H, CH), 8.00 (dd, J = 8.4 Hz, J = 2.0 Hz, 1H, CH), 7.27 (d, J = 8.4 Hz, 1H, CH); 13C NMR (100 MHz, DMSO-d6) δ = 161.5, 150.0, 144.1, 137.6, 132.1, 118.3, 116.6, 115.0, 104.6.
7-Methylquinazoline-2,4(1H,3H)-dione (Table 2, entry 10). White solid, m.p. decomposing at 285 °C, IR (KBr): 3464, 3252, 3042, 2822, 1702, 1630, 1482, 1429, 1380, 1294, 1180, 1147, 1106, 1036, 954, 866, 824, 764, 722, 700, 685, 622 cm−1; 1H NMR (400 MHz, DMSO-d6) δ = 11.13 (s, 1H, NH), 11.01 (s, 1H, NH), 7.71 (d, J = 8.0 Hz, 1H, CH), 6.93 (d, J = 8.0 Hz, 1H, CH), 6.89 (s, 1H, CH), 2.30 (s, 3H, CH3); 13C NMR (100 MHz, DMSO-d6) δ = 162.7, 150.5, 145.6, 141.0, 126.9, 123.7, 115.1, 112.1, 21.5.
6,8-Dichloroquinazoline-2,4(1H,3H)-dione (Table 2, entry 11). Yellow solid, m.p. > 300 °C, IR (KBr): 3482, 3177, 3072, 2830, 2361, 1695, 1609, 1492, 1461, 1417, 1368, 1315, 1285, 1236, 1164, 1088, 1028, 912, 896, 880, 840, 777, 758, 733, 683, 616 cm−1; 1H NMR (400 MHz, DMSO-d6) δ = 11.66 (s, 1H, NH), 10.87 (s, 1H, NH), 7.94 (d, J = 2.4 Hz, 1H, CH), 7.81 (d, J = 2.4 Hz, 1H, CH); 13C NMR (100 MHz, DMSO-d6) δ = 161.1, 149.7, 137.0, 134.2, 126.3, 125.3, 120.2, 117.4.
Acknowledgements
We are grateful to NSFC (21071121, 21172188 and 21104064), SRF for ROCS, SEM, PAPD, Provincial Program of Innovative Research for graduates (KYLX_1428) and the State Key Laboratory of Inorganic Synthesis and Preparative Chemistry at Jilin University (2013-03) for financial support.
Notes and references
-
(a) M. Aresta, in Carbon Dioxide as Chemical Feedstock, Wiley-VCH, Weinheim, Germany, 2010 Search PubMed;
(b) T. Yu, R. Cristiano and R. G. Weiss, Chem. Soc. Rev., 2010, 39, 1435 RSC;
(c) T. Sakakura, J. C. Choi and H. Yasuda, Chem. Rev., 2007, 107, 2365 CrossRef CAS PubMed;
(d) X. Han and M. Poliakoff, Chem. Soc. Rev., 2012, 41, 1428 RSC;
(e) D. J. Darensbourg, R. M. Mackiewicz, A. L. Phelps and D. R. Billodeaux, Acc. Chem. Res., 2004, 37, 836 CrossRef CAS PubMed;
(f) P. G. Jessop, F. Joó and C.-C. Tai, Coord. Chem. Rev., 2004, 248, 2425 CrossRef CAS PubMed;
(g) P. G. Jessop, T. Ikariya and R. Noyori, Chem. Rev., 1995, 95, 259 CrossRef CAS;
(h) A. G. Shaikh and S. Sivaram, Chem. Rev., 1996, 96, 951 CrossRef CAS PubMed;
(i) I. Omae, Coord. Chem. Rev., 2012, 256, 1384 CrossRef CAS PubMed.
-
(a) H. S. Kim, J. J. Kim, B. G. Lee, O. S. Jung, H. G. Jang and S. O. Kang, Angew. Chem., Int. Ed., 2000, 39, 4096 CrossRef CAS;
(b) H. S. Kim, J. J. Kim, S. D. Lee, M. S. Lah, D. Moon and H. G. Jang, Chem.–Eur. J., 2003, 9, 678 CrossRef CAS PubMed;
(c) S.-S. Wu, X.-W. Zhang, W.-L. Dai, S.-F. Yin, W.-S. Li, Y.-Q. Ren and C.-T. Au, Appl. Catal., A, 2008, 341, 106 CrossRef CAS PubMed;
(d) Y. Xiong, H. Wang, R. Wang, Y. Yan, B. Zheng and Y. Wang, Chem. Commun., 2010, 46, 3399 RSC.
-
(a) J. S. Tian, C. X. Miao, J. Q. Wang, F. Cai, Y. Du, Y. Zhao and L. N. He, Green Chem., 2007, 9, 566 RSC;
(b) K. Kohno, J. C. Choi, Y. Ohshima, H. Yasuda and T. Sakakura, ChemSusChem, 2008, 1, 186 CrossRef CAS PubMed;
(c) M. A. Casadei, S. Cesa and L. Rossi, Eur. J. Org. Chem., 2000, 2445 CrossRef CAS.
-
(a) D. J. Darensbourg and S. J. Wilson, Green Chem., 2012, 14, 2665 RSC;
(b) X. B. Lu, W. M. Ren and G. P. Wu, Acc. Chem. Res., 2012, 45, 1721 CrossRef CAS PubMed.
-
(a) R. N. Salvatore, S. I. Shin, A. S. Nagle and K. W. Jung, J. Org. Chem., 2001, 66, 1035 CrossRef CAS;
(b) C. Y. Wu, H. Y. Cheng, R. X. Liu, Q. Wang, Y. F. Hao, Y. C. Yua and F. Y. Zhao, Green Chem., 2010, 12, 1811 RSC;
(c) M. Honda, S. Sonehara, H. Yasuda, Y. Nakagawa and K. Tomishige, Green Chem., 2011, 13, 3406 RSC.
-
(a) E. Graf and W. Leitner, J. Chem. Soc., Chem. Commun., 1992, 623 RSC;
(b) Z. F. Zhang, Y. Xie, W. J. Li, S. Q. Hu, J. L. Song, T. Jiang and B. X. Han, Angew. Chem., Int. Ed., 2008, 47, 1127 CrossRef CAS PubMed;
(c) Z. F. Zhang, S. Q. Hu, J. L. Song, W. J. Li, G. Y. Yang and B. X. Han, ChemSusChem, 2009, 2, 234 CrossRef CAS PubMed.
-
(a) S. Wesselbaum, T. Vom Stein, J. Klankermayer and W. Leitner, Angew. Chem., Int. Ed., 2012, 51, 7499 CrossRef CAS PubMed;
(b) C. A. Huff and M. S. Sanford, J. Am. Chem. Soc., 2011, 133, 18122 CrossRef CAS PubMed;
(c) F. L. Liao, Z. Y. Zeng, C. Eley, Q. Lu, X. L. Hong and S. C. E. Tsang, Angew. Chem., Int. Ed., 2012, 51, 5832 CrossRef CAS PubMed;
(d) M.-A. Courtemanche, M.-A. Legare, L. Maron and F.-G. Fontaine, J. Am. Chem. Soc., 2013, 135, 9326 CrossRef CAS PubMed.
- F. Shi, Y. Q. Deng, T. L. SiMa, J. J. Peng, Y. L. Gu and B. T. Qiao, Angew. Chem., Int. Ed., 2003, 42, 3257 CrossRef CAS PubMed.
- S. G. Liang, H. Z. Liu, T. Jiang, J. L. Song, G. Y. Yang and B. X. Han, Chem. Commun., 2011, 47, 2131 RSC.
-
(a) S. Kikuchi, K. Sekine, T. Ishida and T. Yamada, Angew. Chem., Int. Ed., 2012, 124, 7095 CrossRef;
(b) R. A. Watile, D. B. Bagal, K. M. Deshmukh, K. P. Dhake and B. M. Bhanage, J. Mol. Catal. A: Chem., 2011, 351, 196 CrossRef CAS PubMed;
(c) R. A. Watile, D. B. Bagal, Y. P. Patil, K. P. Dhake and B. M. Bhanage, Tetrahedron Lett., 2011, 52, 6383 CrossRef CAS PubMed;
(d) D. B. Nale, S. Rana, K. M. Parida and B. M. Bhanage, Appl. Catal., A, 2014, 469, 340 CrossRef CAS PubMed;
(e) A. Ueno, Y. Kayaki and T. Ikariya, Green Chem., 2013, 15, 425 RSC.
-
(a) J. Wagner, P. von Matt, B. Faller, N. G. Cooke, R. Albert, R. Sedrani, H. Wiegand, C. Jean, C. Beerli, G. Weckbecker, J.-P. Evenou, G. Zenke and S. Cottens, J. Med. Chem., 2011, 54, 6028 CrossRef CAS PubMed;
(b) T. P. Tran, E. L. Ellsworth, M. A. Stier, J. M. Domagala, H. D. H. Showalter, S. J. Gracheck, M. A. Shapiro, T. E. Joannides and R. Singh, Bioorg. Med. Chem. Lett., 2004, 14, 4405 CrossRef CAS PubMed;
(c) M. B. Andrus, S. N. Mettath and C. J. Song, J. Org. Chem., 2002, 67, 8284 CrossRef CAS PubMed;
(d) P. A. Zunszain, C. Federico, M. Sechi, S. Al-Damluji and C. R. Ganellin, Bioorg. Med. Chem., 2005, 13, 3681 CrossRef CAS PubMed;
(e) F. Russo, G. Romeo, S. Guccione and A. De Blasi, J. Med. Chem., 1991, 34, 1850 CrossRef CAS;
(f) E. Mounetou, J. Legault, J. Lacroix and R. C. Gaudreault, J. Med. Chem., 2001, 44, 694 CrossRef CAS PubMed;
(g) M. B. Andrus, S. N. Mettath and C. Song, J. Org. Chem., 2002, 67, 8284 CrossRef CAS PubMed.
-
(a) M. C. Willis, R. H. Snell, A. J. Fletcher and R. L. Woodward, Org. Lett., 2006, 8, 5089 CrossRef CAS PubMed;
(b) L. N. Zhu, J. Jin, C. Liu, C. J. Zhang, Y. Sun, Y. S. Guo, D. C. Fu, X. G. Chen and B. L. Xu, Bioorg. Med. Chem., 2011, 19, 2797 CrossRef CAS PubMed;
(c) D. Q. Shi, G. L. Dou, Z. Y. Li, S. N. Ni, X. Y. Li, X. S. Wang, H. Wu and S. J. Jia, Tetrahedron, 2007, 63, 9764 CrossRef CAS PubMed;
(d) H. Vorbruggen and K. Krolikiewicz, Tetrahedron, 1994, 50, 6549 CrossRef CAS;
(e) L. Jiarong, C. Xian, S. Daxin, M. Shuling, L. Qing, Z. Qi and T. Jianhong, Org. Lett., 2009, 11, 1193 CrossRef PubMed;
(f) Z. G. Li, H. Huang, H. B. Sun, H. L. Jiang and H. Liu, J. Comb. Chem., 2008, 10, 484 CrossRef CAS PubMed;
(g) M. Akazome, J. Yamamoto, T. Kondo and Y. Watanabe, J. Organomet. Chem., 1995, 494, 229 CrossRef CAS.
-
(a) T. Mizuno, T. Iwai and Y. Ishino, Tetrahedron Lett., 2004, 45, 7073 CrossRef CAS PubMed;
(b) T. Mizuno, N. Okamoto, T. Ito and T. Miyata, Tetrahedron Lett., 2000, 41, 1051 CrossRef CAS;
(c) T. Mizuno, M. Mihara, T. Nakai, T. Iwai and T. Ito, Synthesis, 2007, 2524 CrossRef CAS PubMed;
(d) J. Gao, L. N. He, C. X. Miao and S. Chanfreau, Tetrahedron, 2010, 66, 4063 CrossRef CAS PubMed;
(e) T. Kimura, K. Kamata and N. Mizuno, Angew. Chem., Int. Ed., 2012, 51, 6700 CrossRef CAS PubMed;
(f) T. Kimura, H. Sunaba, K. Kamata and N. Mizuno, Inorg. Chem., 2012, 51, 13001 CrossRef CAS PubMed;
(g) W. Lu, J. Ma, J. Hu, J. Song, Z. Zhang, G. Yang and B. Han, Green Chem., 2014, 16, 221 RSC;
(h) J. Ma, B. Han, J. Song, J. Hu, W. Lu, D. Yang, Z. Zhang, T. Jiang and M. Hou, Green Chem., 2013, 15, 1485 RSC;
(i) Y. Zhao, B. Yu, Z. Yang, H. Zhang, L. Hao, X. Gao and Z. Liu, Angew. Chem., Int. Ed., 2014, 53, 5922 CrossRef CAS PubMed;
(j) H. Zheng, X. Cao, K. Du, J. Xu and P. Zhang, Green Chem., 2014, 16, 3142 RSC;
(k) D. B. Nale, S. Rana, K. Parida and B. M. Bhanage, Catal. Sci. Technol., 2014, 4, 1608 RSC;
(l) Y. P. Patil, P. J. Tambade, K. M. Deshmukh and B. M. Bhanage, Catal. Today, 2009, 148, 355 CrossRef CAS PubMed.
-
(a) G. C. Fortman and S. P. Nolan, Chem. Soc. Rev., 2011, 40, 5151 RSC;
(b) S. Diez-Gonzalez, N. Marion and S. P. Nolan, Chem. Rev., 2009, 109, 3612 CrossRef CAS PubMed;
(c) P. L. Arnold and I. J. Casely, Chem. Rev., 2009, 109, 3599 CrossRef CAS PubMed;
(d) D. Enders, O. Niemeier and A. Henseler, Chem. Rev., 2007, 107, 5606 CrossRef CAS PubMed;
(e) T. Dröge and F. Glorius, Angew. Chem., Int. Ed., 2010, 49, 6940 CrossRef PubMed;
(f) X. Bugaut and F. Glorius, Chem. Soc. Rev., 2012, 41, 3511 RSC;
(g) J. L. Moore and T. Rovis, Top. Curr. Chem., 2010, 291, 77 CrossRef CAS.
- X. Liu, C. Cao, Y. Li, P. Guan, L. Yang and Y. Shi, Synlett, 2012, 1343 CAS.
- H. Zhou, W.-Z. Zhang, C.-H. Liu, J.-P. Qu and X.-B. Lu, J. Org. Chem., 2008, 73, 8039 CrossRef CAS PubMed.
- L. Yang, P. Guan, P. He, Q. Chen, C. Cao, Y. Peng, Z. Shi, G. Pang and Y. Shi, Dalton Trans., 2012, 5020 RSC.
- A. Rouch, T. Castellan, I. Fabing, N. Saffon, J. Rodriguez, T. Constantieux, J.-C. Plaquevent and Y. Genisson, RSC Adv., 2013, 3, 413 RSC.
|
This journal is © The Royal Society of Chemistry 2015 |
Click here to see how this site uses Cookies. View our privacy policy here.