DOI:
10.1039/C5RA07105D
(Paper)
RSC Adv., 2015,
5, 43780-43785
Synthesis, characterization and properties of heat-resistant explosive materials: polynitroaromatic substituted difurazano[3,4-b:3′,4′-e]pyrazines†
Received
20th April 2015
, Accepted 8th May 2015
First published on 8th May 2015
Abstract
The synthesis of three heat-resistant explosive materials: 4,8-di(2,4,6-trinitrophenyl)difurazano[3,4-b:3′,4′-e]pyrazine (1), 4,8-di(2,4-dinitrophenyl)difurazano[3,4-b:3′,4′-e]pyrazine (2), 4-amino-8-(2,4,6-trinitrophenyl)difurazano[3,4-b:3′,4′-e]pyrazine (3),were reported and characterized by 1H NMR,13C NMR, IR as well as elemental analysis. X-ray crystallographic analysis confirmed the structure of 2, as well as displaying intermolecular hydrogen bonding. The thermal behaviors of these compounds were studied with differential scanning calorimetry (DSC) and thermal gravity-differential thermal gravity analysis (TG-DTG) methods. All the compounds showed good thermal stability with exothermic decomposition peaks in the range of 283 °C to 415 °C, on DSC. The sensitivities and calculated explosive performances were also reported for these energetic materials. All the results showed that polynitroaromatic substituted difurazano[3,4-b:3′,4′-e]pyrazines have the potential to be useful heat-resistant explosive materials.
Introduction
The synthesis and development of new heat-resistant explosive materials with improved thermal stability and performance are of interest in modern military and civilian usage, such as in the space industry or deep oil-well drilling.1 Benzene compounds which are polysubstituted with nitro and amino groups have played an important role in the area of high-temperature resistant explosives.2 A representative compound of such explosives is 1,3,5-triamino-2,4,6-trinitrobenzene (TATB),3 which exhibits a density of 1.94 g cm−3 with a decomposition temperature of 360 °C.4 It is often used as the benchmark for comparison of thermal stable and insensitive explosives. The aminonitro heterocyclic compounds are also proved to be useful as thermally stable energetic materials.5 Pagoria et al. reported the synthesis of 2,6-diamino-3,5-dinitropyrazine-1-oxide (LLM-105), having a density of 1.92 g cm−3 and decomposition temperature of 354 °C.6 Other thermally stable explosives include 2,2′,4,4′,6,6′-hexanitrostilbene (HNS), tetranitrodibenzotetraazapentalene (TACOT) and 3,5-dinitro-2,6-bispicrylamino pyridine (PYX) (Fig. 1).7–9 However, new heat-resistant explosives with enhanced thermal stability and explosive performance, as well as lower sensitivity, are still in high demand.
 |
| Fig. 1 Structures of common heat-resistant explosives. | |
The introduction of certain group to aromatic nitro compounds is one of the simplest approaches to impart thermal stability to explosive molecules. For instance, Agrawal et al. reported the synthesis of two thermally stable explosives, 1,3-bis(1,2,4-triazol-3-amino)-2,4,6-trinitrobenzene (BTATNB) and 4,4′-bis(1,2,4-triazol-3-ylamino)-2,2′,3,3′,5,5′,6,6′-octanitroazobenzene (BTDAONAB), by condensing triazole ring with aromatic nitro compounds.10,11 Furazanopyrazine derivatives have been demonstrated their ability to form energetic materials as a result of their high nitrogen contents, high energy density and good oxygen balance.12 On the other hand, furazanopyrazines have scarcely been studied as heat-resistant energetic materials for the low thermal stability. 4H,8H-Difurazano[3,4-b:3′,4′-e]pyrazine (DFP), reported by Sheremetev et al. and Starchenkov et al. respectively,13 exhibit moderate thermal stability with decomposition temperature of 279 °C.14 In our idea, by incorporating polynitro aromatic or amino group to DFP, new generation heat-resistant materials can be derived. 4,8-Di(2,4,6-trinitrophenyl)difurazano[3,4-b:3′,4′-e]pyrazine (1) first reported by Tselinskii et al., who have published its synthesis and some analytical data (IR spectroscopy and element analysis).15 Nevertheless, no data about thermal performance, sensitivity and explosive properties of 1 has been published in the literature.
Herein, we synthesize 1 and two new derivatives 4,8-di(2,4-dinitrophenyl)difurazano[3,4-b:3′,4′-e]pyrazine (2) and 4-amino-8-(2,4,6-trinitrophenyl)difurazano[3,4-b:3′,4′-e]pyrazine (3), characterize the structures and compare the properties of these materials. These substances display outstanding thermal stability and high energy performance comparable with common heat-resistant explosives.
Results and discussion
Synthesis aspects
As illustrated in Scheme 1, the targeted three compounds were obtained by the reactions of the respective nitrobenzene derivatives with DFP or 4-amino-difurazano[3,4-b:3′,4′-e]pyrazine (ADFP) in acetonitrile. The synthetic route was according to the previously reported procedure by Tselinskii et al.15 It was observed that the reactivity of DFP and 1-chloro-2,4-dinitrobenzene was lower than that of 2-chloro-1,3,5-trinitrobenzene. A supposed reason is that the electrophilicity of 2-chloro-1,3,5-trinitrobenzene is stronger than 1-chloro-2,4-dinitrobenzene. ADFP can also be used as an appropriate nucleophile to give the corresponding product in high yield. However, 3 was not able to separate from acetonitrile, the pure compound was obtained by column chromatography.
 |
| Scheme 1 Synthesis of compounds 1, 2 and 3. | |
X-ray crystallography of 2
The molecular structure of the crystalline 2 is shown in Fig. 2. 2 crystallizes in the space group P2(1)/n with four formula units in the unit cell and a density of 1.734 g cm−3. The analytical results indicate that the whole molecule presents mirror symmetry. The bond distances of C8–N3 [1.391(4) Å] and C8–N5 [1.290(3) Å] are close to typical C–N double bond, which are close to the value of DFP.16 The torsion angle of C7–N3–C8–N5 is −179.22°, which indicates that furazanopyrazine ring is coplanar as a result of conjugation. However, the benzene ring are twisted out of the plane, which can be seen from the torsion angle of C7–N3–C6–C5 (−134.92°). Further investigation on hydrogen bonds within the packing arrangement of 2 reveals that the existence of substantial intermolecular interactions contribute to an increase in density (Fig. 3). Relevant data and parameters of the X-ray measurement and refinement are given in Table 1.
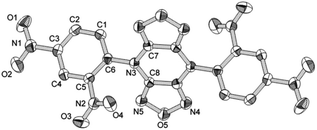 |
| Fig. 2 X-ray structure of 2 with thermal ellipsoids at 50% probability. | |
 |
| Fig. 3 Packing diagram of 2 viewed down the a axis. | |
Table 1 Crystal data and structure refinement parameters of 2
Empirical formula |
C8H3N5O5 |
Formula weight |
249.15 |
T/K |
296(2) |
λ/Å |
0.71073 |
Crystal system |
Monoclinic |
Space group |
P2(1)/n |
Unit cell dimensions/Å, ° |
a = 5.8796(12), α = 90, b = 8.9227(18), β = 98.193, c = 18.378(4), γ = 90 |
V/Å3 |
954.3(3) |
Z |
4 |
Dc/g cm−3 |
1.734 |
Absorption coefficient/mm−1 |
0.149 |
F (000) |
504 |
Goodness-of-fit on F2 |
1.044 |
Final R indices (I > 2σ(I)) |
R1 = 0.0314, wR2 = 0.0786 |
R indices (all data) |
R1 = 0.0347, wR2 = 0.0810 |
Largest diff. peak and hole/e. Å−3 |
0.199 and −0.220 |
Thermal behavior
The typical DSC curves (Fig. 4) indicated that all three compounds exhibit excellent thermal stability. It can be seen from the figure that exothermic decomposition for these nitro compounds occurred between 280 and 420 °C. Among these materials, 1 has the highest thermal stability with exotherm peak at 415.4 °C, which is far superior to those of common heat-resistant materials (TATB: 360 °C; TACOT: 401 °C; PYX: 385 °C). For each compound, no melting event was observed prior to decomposition.
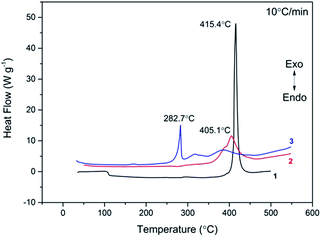 |
| Fig. 4 The DSC curves of 1, 2 and 3. | |
The TG-DTG curves of these compounds are shown in Fig. 5–7, respectively. For 1 and 2, the total weight loss are 95.41% and 85.10% over one decomposition phase. For 3, there are three continuous decomposition processes with the DTG peaks from 297.7 to 376.2 °C, and the total weight loss is 88.57%. In this decomposition temperature range there are three exothermic processes suggested by DSC curve. In contrast to DFP,14 the structures of the employed polynitro aromatic group displayed significant effects upon the thermal stability.
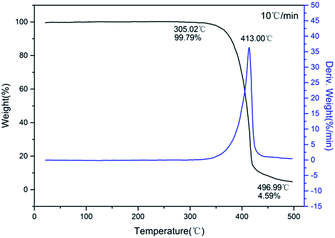 |
| Fig. 5 The TG-DTG curves of 1. | |
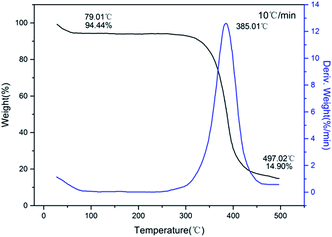 |
| Fig. 6 The TG-DTG curves of 2. | |
 |
| Fig. 7 The TG-DTG curves of 3. | |
Energetic characterization
As shown in Table 2, the furazanopyrazine derivatives exhibit energetic properties. The densities of all the compounds were measured by a gas pycnometer, falling in the range of 1.72–1.82 g cm−3, which equals or exceeds that of common heat-resistant explosives. In addition, 1 possesses the highest density (1.82 g cm−3) in these compounds. This result proves that the increase of nitro group has a positive effect on the molecular density. The sensitivities of all explosives were determined experimentally according to standard BAM methods.17 All of the compounds, with impact sensitivities range from 10 J to 20 J, are less sensitive than HNS (5 J) and PYX (9 J). The friction sensitivity is 240 N for compound 1 and drops to >360 N for compound 3. Thus, according to the range of sensitivities from UN recommendations,18 1 and 2 are to be classified as sensitive towards impact and friction, whereas 3 is sensitive towards impact and insensitive towards friction.
Table 2 Energetic properties of heat-resistant explosives
Compound |
Tda (°C) |
ρb (g cm−3) |
ΔHfc (kJ mol−1) |
Pd (GPa) |
De (m s−1) |
ISf (J) |
FSg (N) |
ESDh (J) |
Decomposition temperature. Density measured by gas pycnometer (25 °C). Heat of formation. Detonation pressure (calculated with K–J equation). Detonation velocity (calculated with K–J equation). Impact sensitivity. Friction sensitivity. Electrostatic discharge sensitivity. Crystal density at 298 K. |
1 |
415 |
1.82 |
894.1 |
28.2 |
7874 |
10 |
240 |
1 |
2 |
405 |
1.74 (1.73)i |
845.6 |
23.0 |
7200 |
16 |
300 |
1.5 |
3 |
283 |
1.72 |
782.6 |
25.7 |
7641 |
20 |
>360 |
3 |
TATB |
360 |
1.94 |
−139.8 |
29.1 |
7867 |
50 |
>360 |
11 |
HNS |
324 |
1.73 |
57.7 |
21.8 |
7170 |
5 |
240 |
5 |
TACOT |
401 |
1.76 |
536.0 |
24.5 |
7200 |
28 |
>360 |
7 |
PYX |
385 |
1.77 |
80.3 |
24.2 |
7448 |
9 |
280 |
1 |
The heats of formation of furazanopyrazine compounds were calculated using the Gaussian 09 quantum chemistry package by designing appropriate isodesmic reactions (see ESI†). All compounds exhibit high positive heats of formation (782.6 kJ mol−1 to 894.1 kJ mol−1), which are even far more than that of common heat-resistant explosives. Detonation parameters were calculated by the Kamlet–Jacobs equation by using the calculated values for the heats of formation and the experimental values for the densities.19 The calculated detonation pressures and velocities lie in the range of 23.0–28.2 GPa and 7200–7874 m s−1, respectively. In terms of detonation velocity, 1 is superior to those of thermally stable explosives. The calculated properties coupled with the rather high thermal stabilities suggest that these furazanopyrazine compounds may be attractive candidates for heat-resistant energetic applications.
Conclusions
In summary, the syntheses and subsequent characterizations of nitrophenyl derivatives of DFP were carried out. Single-crystal X-ray measurement was accomplished for 2 and deliver insight into structural characteristic. The thermodynamic analysis (DSC and TG-DTG analysis) results indicate these compounds have excellent thermal stability. Compound 3 was the most insensitive derivative, with an impact sensitivity 20 J and friction sensitivity >360 N. As a result of the experimental densities and calculated high positive heats of formation, the calculated detonation pressures and velocities fall in the range of 23.0–28.2 GPa and 7200–7874 m s−1. Worth mentioning is compound 1, which has a remarkably high decomposition temperature of 415 °C and good performance value, make it an interesting candidate for applications in heat-resistant explosive.
Experimental section
General information
All reagents and solvents were used as received. 4H,8H-Difurazano[3,4-b:3′,4′-e]pyrazine (DFP) and 4-amino-difurazano[3,4-b:3′,4′-e]pyrazine (ADFP) were prepared by the literature procedure.13b,16 1H NMR and 13C NMR were obtained on a Bruker AV500 NMR spectrometer. Infrared spectra were obtained from KBr pellets on a Nicolet NEXUS870 infrared spectrometer in the range of 4000–400 cm−1. Elemental analyses (C, H and N) were performed on a VARI-El-3 elemental analyzer. Differential scanning calorimetry (DSC) were carried out in a platinum sample container using a Shimadzu DSC-60 at a heating rate of 10 °C min−1. The TG-DTG experiments were performed with a SDT-Q600 apparatus (TA, USA) operating at a heating rate of 10 °C min−1 in a flow of dry oxygen-free nitrogen at 10 mL min−1. For all materials, the impact sensitivity were determined with a ZBL-B impact sensitivity instrument, the friction sensitivity were determined with a FSKM 10 friction sensitivity instrument, the electrostatic discharge sensitivity were determined with a ESD 2008A electric spark tester.
X-ray crystallography of 2
A thin plate of dimensions 0.38 × 0.31 × 0.24 mm2 was mounted on a MiteGen MicroMesh using a small amount of Cargille Immersion Oil. Diffraction data were collected at 296(2) K on a Oxford diffraction Gemini S Ultra diffractometer using Mo-Kα radiation (λ = 0.71073 Å). The structure was solved by direct methods using SHELXS-97 program20 and refined against F2 by full-matrix least-squares using SHELXL-97 program.21
Theoretical study—computational details
Computations were performed with the Gaussian 09 (Revision B. 01) suite of programs.22 The geometric optimization of the structures and frequency analyses were carried out using B3LYP functional with the 6-31+G** basis set, and single-point energies were calculated at the MP2(full)/6-311++G** level. All of the optimized structures were characterized to be true local energy minima on the potential-energy surface without imaginary frequencies. According to the method of isodesmic reactions, the gas phase enthalpies of formation were computed and the enthalpy of reaction is obtained by combining the MP2/6-311++G**energy difference for the reactions, the scaled zero point energies, and other thermal factors. The detonation velocity (D) and detonation pressure (P) were evaluated by the empirical Kamlet–Jacobs (K–J) equations as shown in eqn (1)–(3). |
D = 1.01Φ1/2(1.011 + 1.312ρ)
| (2) |
D is the predicted detonation velocity (m s−1), P is the detonation pressure (GPa), and ρ is the compound density (g cm−3). Φ, N, M and Q are characteristic parameters of an explosive; Q is the chemical energy of detonation (kJ g−1). The densities and the calculated heats of formation were used to compute the D and P values.
4,8-Di(2,4,6-trinitrophenyl)difurazano[3,4-b:3′,4′-e]pyrazine (1)
To a solution of DFP (7 g, 42 mmol) in anhydrous acetonitrile (100 mL) was added NEt3 (8.48 g, 84 mmol). After stirring for 20 min at room temperature, a solution of 2-chloro-1,3,5-trinitrobenzene (22.28 g, 90 mmol) in anhydrous acetonitrile (50 mL) was added. The reaction mixture was held for 5 h at room temperature and was left stand for 24 h. The solid was filtered and washed with acetonitrile, hot water and methanol to give 19.70 g (79.8%) of the desired product as yellow powder. 1H NMR (DMSO-d6, 500 MHz, ppm): δ = 9.47 (s, 4H, C6H2). 13C NMR (DMSO-d6, 125 MHz, ppm): δ = 149.08, 146.44, 145.89, 126.91, 123.44. IR (KBr, cm−1): ν = 3100, 2887, 1613, 1584, 1549, 1478, 1409, 1386, 1341, 1191, 1083, 914, 846. Anal. calcd for C16H4N12O14: C 32.67, H 0.69, N 28.57; found C 32.49, H 0.47, N 28.56%.
4,8-Di(2,4-dinitrophenyl)difurazano[3,4-b:3′,4′-e]pyrazine (2)
To a solution of DFP (1 g, 6 mmol) in anhydrous acetonitrile (15 mL) was added NEt3 (1.21 g, 12 mmol). After stirring for 20 min at room temperature, a solution of 1-chloro-2,4-dinitrobenzene (2.43 g, 12 mmol) in anhydrous acetonitrile (5 mL) was added. The reaction mixture was refluxed for 8 h, cooled to room temperature, and was left stand for 24 h at −18 °C. The solid was filtered and washed with acetonitrile, hot water and methanol to give 1.87 g (62.3%) of the desired product as red crystal. 1H NMR (acetone-d6, 500 MHz, ppm): δ = 9.13 (d, 1H, C6H3), 8.90 (s, 1H, C6H3), 8.50 (d, 1H, C6H3). 13C NMR (acetone-d6, 125 MHz, ppm): δ = 149.40, 147.94, 145.70, 133.61, 132.22, 131.98, 123.38. IR (KBr, cm−1): ν = 3092, 1707, 1651, 1612, 1588, 1550, 1340, 1086, 1055, 922, 830. Calcd for C16H6N10O10: C 38.57, H 1.21, N 28.11; found C 38.52, H 1.25, N 28.39%.
4-Amino-8-(2,4,6-trinitrophenyl)difurazano[3,4-b:3′,4′-e]pyrazine (3)
To a solution of ADFP (1.36 g, 7.5 mmol) in anhydrous acetonitrile (20 mL) was added NEt3 (0.75 g, 7.5 mmol). After stirring for 10 min at room temperature, a solution of 2-chloro-1,3,5-trinitrobenzene (1.86 g, 7.5 mmol) in anhydrous acetonitrile (10 mL) was added. The reaction mixture was held for 4 h at room temperature. The solvent was evaporated under vacuum, and the residue was purified by column chromatography (silica gel, eluted using 20% CH3COOEt–petroleum ether) to give 2.48 g (84.4%) of the desired product as brown powder. 1H NMR (DMSO-d6, 500 MHz, ppm): δ = 9.38 (s, 2H, C6H2), 6.03 (s, 2H, NH2). 13C NMR (DMSO-d6, 125 MHz, ppm): δ = 149.67, 148.66, 146.46, 145.82, 126.50, 124.49. IR (KBr, cm−1): ν = 3367, 3095, 1613, 1547, 1551, 1406, 1386, 1342, 1085, 941, 827. Calcd for C10H4N10O8: C 30.62, H 1.03, N 35.71; found C 30.57, H 1.08, N 35.91%.
Acknowledgements
We gratefully acknowledge financial support by the National Natural Science Foundation of China (grant no. 21373157).
Notes and references
-
(a) E. E. Kilmer, J. Spacecr. Rockets, 1968, 5, 1216 CrossRef CAS;
(b) T. Urbanski and S. K. Vasudeva, J. Sci. Ind. Res., 1978, 37, 250 CAS;
(c) T. Urbanski, Chemistry and Technology of Explosives, Pergamon, Oxford, 1984, vol. 4, pp. 202–204 Search PubMed;
(d) J. P. Agrawal, Cent. Eur. J. Energ. Mater., 2012, 9, 273 CAS;
(e) C. Li, L. Liang, K. Wang, C. Bian, J. Zhang and Z. Zhou, J. Mater. Chem. A, 2014, 2, 18097 RSC.
-
(a) J. P. Agrawal, Prog. Energy Combust. Sci., 1998, 24, 1 CrossRef CAS;
(b) J. P. Agrawal, Propellants, Explos., Pyrotech., 2005, 30, 316 CrossRef CAS PubMed;
(c) O. Siri and P. Braunstein, New J. Chem., 2005, 29, 75 RSC.
- C. L. Jackson and J. F. Wing, Am. Chem. J., 1888, 10, 283 Search PubMed.
-
(a) F. Taylor, Synthesis of New High Explosives II. Derivatives of 1,3,5-Tribromo-2,4,6-trinitrobenzene, U. S. Naval Ordnance Laboratory Report NAVORD 4405, 1956 Search PubMed;
(b) Y. A. Aminov, M. M. Gorshkov, V. T. Zaikin, G. V. Kovalenko, Y. R. Nikitenko and G. N. Rykovanov, Combust., Explos. Shock Waves, 2004, 38, 235 CrossRef.
- R. W. Millar, S. P. Philbin, R. P. Claridge and J. Hamid, Propellants, Explos., Pyrotech., 2004, 29, 81 CrossRef CAS PubMed.
-
(a) P. F. Pagoria, A. R. Mitchell, R. D. Schmidt, R. I. Simpson, F. Gracia, J. Forbes, J. Cutting, R. Lee, R. Swansiger and D. M. Hoffmann, Presented at the Insensitive Munitions and Energetic Materials Technology Symposium, San Diego, CA, 1998;
(b) L. E. Fried, M. R. Manaa, P. F. Pagoria and R. L. Simpson, Annu. Rev. Mater. Res., 2001, 31, 291 CrossRef CAS;
(c) Z. Chaoyang, S. Yuanjie, Z. Xiaodong, D. Haishan and W. Xinfeng, J. Mol. Struct.: THEOCHEM, 2005, 728, 129 CrossRef PubMed.
- K. G. Shipp, J. Org. Chem., 1964, 29, 2620 CrossRef CAS.
- M. L. Trudell, G. Subramanian, G. Eck and J. H. Boyer, Mater. Res. Soc. Symp. Proc., 1996, 418, 37 CrossRef CAS PubMed.
- H. S. Jadhav, M. B. Talwar, D. D. Dhavale, S. N. Asthana and V. N. Krishnamurthy, Indian J. Heterocycl. Chem., 2006, 15, 383 CAS.
- J. P. Agrawal, Mehilal, U. S. Prasad and R. N. Surve, New J. Chem., 2000, 24, 583 RSC.
- Mehilal, N. Sikder, A. K. Sikder and J. P. Agrawal, Indian J. Eng. Mater. Sci., 2004, 11, 516 CAS.
-
(a) A. B. Sheremetev and I. L. Yudin, Russ. Chem. Rev., 2003, 72, 87 CrossRef CAS PubMed;
(b) V. Thottempudi, P. Yin, J. Zhang, D. A. Parrish and J. M. Shreeve, Chem.–Eur. J., 2014, 20, 542 CrossRef CAS PubMed.
-
(a) A. B. Sheremetev and I. L. Yudin, Mendeleev Commun., 1996, 6, 247 CrossRef PubMed;
(b) I. B. Starchenkov and V. G. Andrianov, Chem. Heterocycl. Compd., 1996, 32, 618 CrossRef.
- N. Liu, B. Wang, H. Li, Y. Li and W. Lai, Huozhayao Xuebao, 2014, 37, 12 CAS.
- I. V. Tselinskii, S. F. Mel'nikova, T. V. Romanova, S. V. Pirogov, G. Kh. Khisamutdinov, T. A. Mratkhuzina, V. L. Korolev, I. Z. Kondyukov, I. Sh. Abdrakhmanov and S. P. Smirnov, Russ. J. Org. Chem., 1997, 33, 1656 CAS.
- I. B. Starchenkov, V. G. Andrianov and A. F. Mishnev, Chem. Heterocycl. Compd., 1997, 33, 216 CrossRef CAS.
-
(a) http://www.bam.de;
(b) NATO standardization agreement (STANAG) on Explosives, impact sensitivity tests, no. 4489, Ed. 1, Sept. 17, 1999;
(c) NATO standardization agreement (STANAG) on Explosives, friction sensitivity tests, no. 4487, Ed. 1, Aug. 22, 2002;
(d) NATO standardization agreement (STANAG) on Explosives, electrostatic discharge sensitivity tests, no. 4490, Ed. 1, Feb. 19, 2001.
- Test methods according to the UN Manual of Tests and Criteria, Recommendations on the Transport of Dangerous Goods, United Nations Publication, New York, Geneva, 4th revised ed., 2003: Impact: insensitive >40 J, less sensitive ≥35 J, sensitive ≥4 J, very sensitive ≥3 J; friction: insensitive >360 N, less sensitive 360 N, sensitive <360 N and >80 N, very sensitive ≤80 N and extremely sensitive ≤10 N.
-
(a) M. J. Kamlet and S. J. Jacobs, J. Chem. Phys., 1968, 48, 23 CrossRef CAS PubMed;
(b) M. J. Kamlet and J. E. Ablard, J. Chem. Phys., 1968, 48, 36 CrossRef CAS PubMed.
- G. M. Sheldrick, SHELXS-97, Program for Crystal Structure Solution, University of Göttingen, Germany, 1997 Search PubMed.
- G. M. Sheldrick, SHELXL-97, Program for Crystal Structure Refinement, University of Göttingen, Germany, 1997 Search PubMed.
- M. J. Frisch, G. W. Trucks, H. B. Schlegel, G. E. Scuseria, M. A. Robb, J. R. Cheeseman, G. Scalmani, V. Barone, B. Mennucci, G. A. Petersson, H. Nakatsuji, M. Caricato, X. Li, H. P. Hratchian, A. F. Izmaylov, J. Bloino, G. Zheng, J. L. Sonnenberg, M. Hada, M. Ehara, K. Toyota, R. Fukuda, J. Hasegawa, M. Ishida, T. Nakajima, Y. Honda, O. Kitao, H. Nakai, T. Vreven, J. A. Montgomery Jr, J. E. Peralta, F. Ogliaro, M. Bearpark, J. J. Heyd, E. Brothers, K. N. Kudin, V. N. Staroverov, R. Kobayashi, J. Normand, K. Raghavachari, A. Rendell, J. C. Burant, S. S. Iyengar, J. Tomasi, M. Cossi, N. Rega, J. M. Millam, M. Klene, J. E. Knox, J. B. Cross, V. Bakken, C. Adamo, J. Jaramillo, R. Gomperts, R. E. Stratmann, O. Yazyev, A. J. Austin, R. Cammi, C. Pomelli, J. W. Ochterski, R. L. Martin, K. Morokuma, V. G. Zakrzewski, G. A. Voth, P. Salvador, J. J. Dannenberg, S. Dapprich, A. D. Daniels, O. Farkas, J. B. Foresman, J. V. Ortiz, J. Cioslowski and D. J. Fox, Gaussian 09, Revision B. 01, Gaussian, Inc., Wallingford, CT, USA, 2009 Search PubMed.
Footnote |
† Electronic supplementary information (ESI) available: X-ray crystallography, theoretical study, 1H and 13C NMR spectra of compounds 1–3. CCDC 955441. For ESI and crystallographic data in CIF or other electronic format see DOI: 10.1039/c5ra07105d |
|
This journal is © The Royal Society of Chemistry 2015 |
Click here to see how this site uses Cookies. View our privacy policy here.