DOI:
10.1039/C5FO00924C
(Paper)
Food Funct., 2016,
7, 417-424
Formulation and characterization of nanoencapsulated curcumin using sodium caseinate and its incorporation in ice cream
Received
28th July 2015
, Accepted 13th October 2015
First published on 15th October 2015
Abstract
In the present investigation, the preparation and characterization of a curcumin nanoemulsion with milk protein (sodium caseinate) and its incorporation into ice cream were undertaken. Among the different combinations, the most stable formulation was observed using milk fat (8%), medium chain triglycerides (2%), curcumin (0.24%) and sodium caseinate (6%) with a mean particle size of 333.8 ± 7.18 nm, a zeta potential of −44.1 ± 0.72 mV and an encapsulation efficiency of 96.9 ± 0.28%. The effect of different processing conditions (heating, pH and ionic strength) on the particle size distribution and zeta potential of the nanoemulsion was evaluated. During heat treatment, the particle size of the nanoemulsion was increased from 333.8 ± 7.18 to 351.1 ± 4.04 nm. The nanoemulsion was destabilized at pH 4.6 and the particle size increased above and below pH 5.0. However, there was a slight increase in the particle size with a change in the ionic concentration. The release kinetics data suggested that in simulated gastro-intestinal digestion, the nanoemulsion was stable against pepsin digestion (a 5.25% release of curcumin), while pancreatic action led to a 16.12% release of curcumin from the nanoemulsion. Finally, our formulation was successfully incorporated into ice cream and the sensory attributes were evaluated. No significant difference was observed in the scores of the sensory attributes between the control and ice cream prepared with a curcumin nanoemulsion. Moreover, the encapsulation efficiency of the curcumin incorporated into the ice cream was 93.7%, which indicates that it can withstand the processing conditions. The findings suggest that ice cream is a suitable dairy product for the delivery of lipophilic bioactive components (curcumin) which can be used for therapeutic purposes.
1. Introduction
Nowadays, treatments available for human diseases (e.g. obesity, diabetes and cancer) are very cost effective. Alternatively, people are looking towards naturally occurring bioactive components in plants. The bioactive components (e.g. curcumin) can be incorporated into food systems for the development of health promoting functional foods and this has been a growing area recently.1 Curcumin (diferuloylmethane) is a natural hydrophobic yellow coloured pigment extracted from turmeric (Curcuma longa) and widely used as a dietary spice or colouring agent in India and Asian countries.2Curcuma longa contains three different types of curcuminoids viz., curcumin (80%), demethoxycurcumin (15%) and bisdemethoxycurcumin (5%).3,4 It has also been used as a remedy since ancient times because of several pharmacological properties such as its anti-oxidative, anti-inflammatory, anti-parasitic, anti-mutagenic, anticancer, chemoprotective, hepatoprotective, antimicrobial and antiviral activities.5–8 High doses (8.0 g per day) of curcumin used in phase I human clinical trials are associated with limiting toxicity.9 However its application as a functional ingredient is currently limited because of its poor water-solubility, extremely low absorption & bioavailability, and rapid degradation under neutral and alkaline pH conditions.4 To improve the bioavailability of curcumin, several researchers have been investigating different carriers like albumin,10,11 β-lactoglobulin,12 phospholipids,13 chitosan,14 cyclodextrins,15 polyvinyl alcohol/polyvinyl alcohol hydrogels,16 bovine whole casein micelles17 and whey protein concentrates.18
Different methods (the amorphous solid form, solid dispersions, melt extrusion, liposomes and nanocarriers) are available to overcome the difficulties with hydrophobic compounds.19,20 The micro/nanoencapsulation of bioactive molecules helps their easy incorporation into food and beverage systems, improving their poor solubility and sensitivity to processing conditions and masking the colour and adverse effects on the sensory attributes. However, there are currently limit restrictions on food grade surfactants because they are synthetic in nature and because of economic or sensory issues, and they are not acceptable in all countries.21 Nanoemulsions are transparent heterogeneous mixtures of oil in water (O/W) and are stabilized by emulsifiers. Compared to conventional microemulsions, nanoemulsions are thermodynamically stable due to their nanosized structures.22 These are predominately produced by either high energy emulsification (high pressure homogenization micro-fluidization and ultrasonication) or by low energy emulsification.23
Milk proteins such as casein are used as emulsifiers in the stabilization of emulsions and for the encapsulation of lipophilic components. Casein can form a thicker interfacial layer (10 nm) around the lipid droplets compared to whey proteins (1–2 nm). In addition, it is easily degraded by gastric enzymes, because of its open tertiary structure (due to a high proline content), to release the encapsulated materials.24 Moreover, in the food industry sodium caseinate (NaCas) is widely used as an emulsifier because of its emulsification, water & fat binding, thickening, gelation, increased viscosity and stability properties.25 The pharmaceutical and food sectors are focusing great attention on developing suitable delivery systems for the incorporation of curcumin O/W emulsions and these need to be stable in food products. In view of the above, in the present investigation an attempt has been made to formulate and characterize an O/W curcumin nanoemulsion with NaCas and subsequently incorporate this in ice cream because it is an ideal food system – a nutritious dessert widely consumed by all age-groups.
2. Materials and methods
2.1. Materials
Curcumin (purity ∼97%, from Curcuma longa L.) was obtained from Plant Lipids Pvt. Ltd (Kerala, India). Medium chain triglycerides-60 (MCT-60) was procured from Kamani Oil Industries Pvt. Ltd (Mumbai, India). Sodium caseinate was the product of Thomas Baker Pvt. Ltd (Mumbai, India). Pepsin and pancreatin were purchased from Sisco Research Laboratories Pvt. Ltd and Hi Media laboratories Pvt. Ltd (Mumbai, India), respectively. All other reagents and chemicals were of analytical grade.
2.2. Solubility of curcumin in different oils
In the solubility studies of curcumin at room temperature, five different oils such as butter, palm, olive, sunflower and MCT-60 were used. Different concentrations (0.12–0.6%) of curcumin were added to 5 mL of each oil in test tubes and mixed using a vortex mixer to dissolve the curcumin properly. The mixture was then centrifuged at 1300g for 10 min in order to check for visible undissolved particles. The maximum concentration at which no undissolved sediments of curcumin appeared after centrifugation was termed as the solubility (mg mL−1).
2.3. Preparation of O/W curcumin nanoemulsion
A two-step nanoemulsion was prepared according to Jafari et al. (2007),26 with slight modification. Among the different formulations we tried, the most stable O/W curcumin emulsion was observed with curcumin (0.12–0.6%), MCT-60 (1–5%), and milk fat (5–9%) with NaCas (1–7%) alone and was used further in this study (Fig. 1). To formulate the O/W emulsion, an aqueous phase containing the emulsifier NaCas (6%) was dissolved completely in milk fat (8%) by stirring at 60 °C, and curcumin (0.24%) which was previously solubilized in MCT-60 (2%) was added into it and termed as the oil phase. The entire formula was magnetically stirred for 15 minutes to obtain a coarse emulsion and further it was passed through the homogenizer (200 kg cm−2, GOMA Engineering Pvt. Ltd, Mumbai) with a two-stage cycle. The final emulsion was lyophilized (−80 °C and <10 mm mercury pressure, LYPHLOCK, Labconco, Kansas City, MO) to get the freeze dried powder.
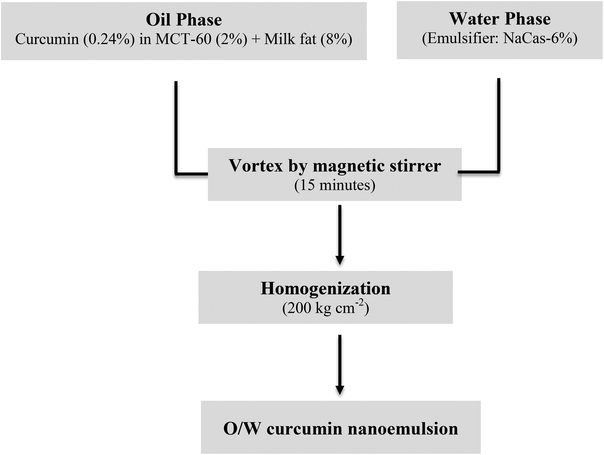 |
| Fig. 1 Preparation of oil in water (o/w) curcumin nanoemulsion: MCT = medium chain triglycerides; NaCas = sodium caseinate. | |
2.4. Characterization of the nanoemulsion
2.4.1. Particle size and zeta potential measurement.
The particle size distribution and polydispersity index (PDI) of the nanoemulsion were determined using dynamic light scattering (Malvern Instruments, Worcestershire, UK) measurements at ambient temperature using a scattering angle of 90° with an average of 12 runs. To avoid multiple scattering, the emulsion was diluted ten times with deionized water. The zeta potential was also measured in a similar way. All the measurements were recorded in triplicate.
2.4.2. Stability and solubility of the nanoemulsion.
The stability of the curcumin nanoemulsion was measured by the method of Dalev and Simenova (1995).27 Briefly, the emulsion (1 mL) was centrifuged at 1300g for 10 minutes in order to check for visible undissolved particles. For the solubility measurements, equal quantities (1 mg mL−1) of curcumin alone and freeze dried nanoencapsulated curcumin were dissolved in phosphate buffered saline (PBS-0.01 M, pH 7.4).
2.4.3. Effect of processing conditions on physico-chemical characteristics.
The effect of different processing conditions, such as the method of heat treatment, the pH and the ionic strength, on the O/W curcumin nanoemulsion was examined. The emulsion was subjected to different heat treatments i.e. pasteurization (63 °C for 30 minutes), boiling (95 °C for 10 minutes) and sterilization (121 °C for 15 minutes). To measure the effect of pH of the nanoemulsion, a pH range (3–7) was used. In the case of the ionic strength, different salt concentrations (0.1–1 M NaCl) were added to the emulsion. For all the above processing conditions, the mean particle size, PDI and zeta potential were evaluated.
2.4.4. Entrapment efficiency.
The encapsulation efficiency was determined and calculated using the method of Surassmo et al. (2010),28 with modifications. Our formulation was passed through the Amicon® Ultra-15 sample concentrator (molecular weight cut off, 100
000 Da; Merck Life science Pvt. Ltd, Mumbai, India) and centrifuged at 1300g for 30 minutes. After centrifugation, the permeate was collected to calculate the encapsulation efficiency by measuring its total phenolic content using the Folin-Ciocalteu method given by Zheng and Wang (2001).29
2.4.5.
In vitro release of curcumin under simulated digestion.
The release of the curcumin nanoemulsion under gastro-intestinal conditions was carried out using simulated gastric fluid (SGF)30 and simulated intestinal fluid (SIF)31 methods, respectively.
Simulated gastric fluid.
The SGF mixture (NaCl-125 mM, KCl-7 mM, NaHCO3-45 mM and pepsin-0.32%) was added to the curcumin emulsion in the ratio of 3
:
1 and adjusted to pH 1.5. The sample was kept in a shaking water bath at 37 °C for 2 hours. Every 1 hour, a sample was removed from the water bath and the enzyme was deactivated at 90 °C for 10 minutes.
Simulated intestinal fluid.
SIF was prepared by adding pancreatin (4 mg mL−1) to bile salts (25 mg mL−1) and adjusted to pH 7.5. After 2 hours, the SGF mixture was added to the SIF and further incubated at 37 °C in a water bath for another 2.5 hours. Each hour, a sample was removed and the enzyme was deactivated at 90 °C for 10 minutes. A gallic acid phenolic compound was used as a standard curve. For the release (%) of curcumin after the SGF and SIF methods, the total phenolic content was calculated according to the calibration curve and mentioned in section 2.4.4.
2.4.6. Morphological characterization using scanning electron microscopy.
The curcumin nanoemulsion morphology was evaluated using scanning electron microscopy (SEM). The powder was sprinkled on double-sided adhesive tape mounted on SEM stubs and coated with gold in a vacuum evaporator. Then it was examined under SEM with a 20KX magnification (Electron Microscopy Ltd, Cambridge, UK).
2.5. Incorporation of the nanoemulsion into ice cream
For the suitable delivery of the nanoencapsulated curcumin, a dairy product i.e. ice cream was prepared and this is presented in Fig. 2. All the ingredients (skim milk powder, stabilizer, emulsifier, milk and cream) were mixed together using a plunger and further heated to 70–72 °C. After that, the prepared emulsion with or without curcumin was added into the ice cream mix and subjected to homogenization at a two stage cycles. Further, the entire mixture was pasteurized at 80 ± 2 °C for 5 minutes and cooled by placing it under cold conditions at 5 ± 2 °C overnight. The next day, flavour (mango) was added to the ice cream mix and it was transferred to a batch freezer (GOMA Engineering Pvt. Ltd Mumbai, India) to collect the ice cream product in sterile plastic cups. The samples were stored in a freezer at −18 ± 2 °C and used for further analysis.
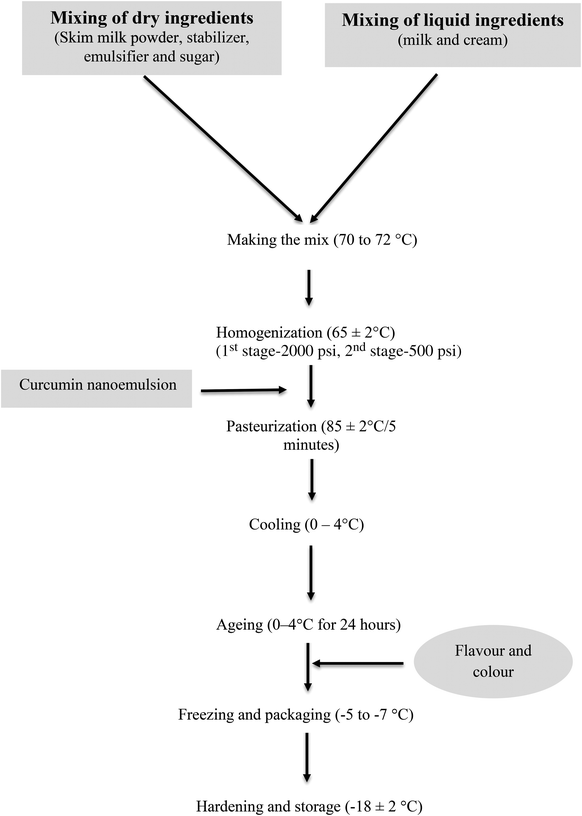 |
| Fig. 2 Flow diagram for the incorporation of the nanoemulsion into ice cream. | |
2.5.1. Sensory attributes of curcumin encapsulated into ice cream.
Samples of the control (without curcumin) and of encapsulated curcumin incorporated in the ice cream were studied for their sensory evaluation (colour & appearance, body & texture, melting quality and flavour) using a 9-point hedonic scale.32 The samples were drawn from the refrigerator just before serving score cards to the panel. Finally, the encapsulation efficiency of the curcumin nanoemulsion in the product was determined. To ensure impartiality and minimize subjective bias the samples were labelled as A (without curcumin) and B (with curcumin), respectively.
2.6. Statistical analysis
All the data in this study were represented as mean values ± the standard errors of the mean (S.E.M). Statistical analysis was performed using Student's t-test and oneway ANOVA using Tukey's test (GraphPad Software, version 5.01) and a p value <0.05 was considered to be a significant difference.
3. Results and discussion
3.1. Curcumin solubility in oil phase
Different concentrations of curcumin (0.12–0.6%) were dissolved in butter, palm, olive, MCT-60 and sunflower oil. We found that curcumin showed the maximum solubility in MCT-60 (0.40 ± 0.01 mg mL−1, mean ± SEM) followed by palm (0.35 ± 0.06), butter (0.30 ± 0.04), olive (0.20 ± 0.08) and sunflower oil (0.2 ± 0.03 mg mL−1). Our results were corroborated by Ahmed et al. (2012),33 who reported that the maximum solubility of curcumin was found in MCT compared to long chain triglycerides. The higher solubility in MCT-60 may be due to the molecular characteristics (molecular weight, polarity and interactions) of the oil. Based on these results, MCT was used as the lipid phase in this study for the preparation of the curcumin nanoemulsion.
3.2. Physico-chemical characterization of O/W curcumin nanoemulsion
3.2.1. Effect of NaCas on droplet size and zeta potential.
The mean particle size (Z-average diameter) and PDI of our formulation were found to be 333.8 ± 7.28 nm and 0.15 ± 0.01, respectively. The zeta potential was recorded as −44.1 ± 0.72 mV. To improve the storage stability, freeze dried nanoemulsions are used widely and are easily incorporated into products. As shown in Table 1, the freeze dried nanoemulsion was reconstituted based on the total solids and was further analysed for its mean particle size, PDI and zeta potential. After reconstitution, the curcumin nanoemulsion mean particle size was significantly (p < 0.05) increased due to particle aggregation, whereas, the zeta potential was decreased. Using MCT, a small particle size with a narrow distribution was observed as compared to having short chain triglycerides as the lipid phase.33 An increase in the NaCas concentration (0.5 to 5 wt%) in the emulsion results in a decrease in the average droplet diameter due to the wide coverage of the protein concentrated on the oil droplet preventing its aggregation, which is in agreement with our results.34,35
Table 1 Mean particle size, zeta potential and PDI of fresh and reconstituted curcumin nanoemulsion
Characteristics |
Fresh |
Reconstituted |
Values mentioned are the means ± SEM (n = 3). Mean values within a row with unlike superscript letters differ significantly (p < 0.05). PDI = polydispersity index. |
Mean particle size (nm) |
333.8 ± 7.8a |
492.3 ± 12.4b |
Zeta potential (mV) |
−44.1 ± 0.72a |
−42.4 ± 0.2a |
PDI |
0.15 ± 0.01a |
0.21 ± 0.09a |
3.2.2. Stability and solubility of nanoemulsion.
For the stability, we observed no phase separation after centrifugation. As mentioned above, at physiological pH (7.2 to 7.4) curcumin was unstable and degraded immediately. To challenge the use of a curcumin nanoemulsion in drug delivery in pharmacological therapy, we solubilized curcumin alone or the nanoemulsion (1 mg mL−1) in PBS (pH 7.4) and observed the complete solubilization of the freeze dried curcumin nanoemulsion compared to native curcumin which is less soluble with undissolved flakes (Fig. 3).
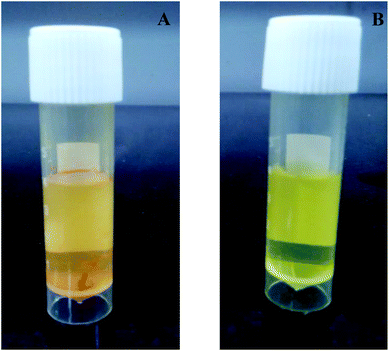 |
| Fig. 3 Solubility of curcumin alone (A) and nanoencapsulated curcumin (B) in phosphate buffered saline. | |
3.2.3. Effect of processing conditions on physico-chemical characteristics.
Our formulation was tested by changing various processing parameters like the temperature, pH and ionic strength (Table 2). The curcumin nanoemulsion prepared with NaCas was analysed with respect to its mean particle size and zeta potential for different temperature conditions. The particle size distribution of the nanoemulsion was increased from 340.0 ± 7.18 to 351.1 ± 4.04 nm with an increase in temperature (63 °C to 95 °C). The zeta potential of the nanoemulsion was increased towards a more negative charge with a change in the temperature from −45.5 ± 0.38 mV at 63 °C to −47.8 ± 0.35 mV at 95 °C but it decreased to −42.5 ± 0.62 mV at 121 °C. There was no linear relation between the temperature and mean particle size of the nanoemulsion and these were stable to physical separation/aggregation at all temperature ranges. The stability of the heated emulsions at 121 °C for 15 minutes increased almost linearly with an increase in the caseinate concentration (1 to 4%) and there was no further increase in the particle size at higher concentrations compared to the unheated emulsions.36
Table 2 Effect of NaCas on different processing conditions
|
Particle size (nm) |
Zeta potential (mV) |
Value mentioned is the mean ± S.E.M (n = 3). Mean values within a column with unlike superscript letters differ significantly (p < 0.05). NaCas = sodium caseinate. |
Heat treatment |
63 °C/30 min |
340.0 ± 7.18a |
−45.5 ± 0.38a |
80 °C/30 min |
349.4 ± 3.26b |
−46.3 ± 0.41a |
95 °C/10 min |
351.1 ± 4.04b |
−47.8 ± 0.35a |
121 °C/15 min |
336.2 ± 5.90a |
−42.5 ± 0.62a |
pH |
3 |
340.9 ± 8.04a |
40.9 ± 0.46a |
4 |
338.5 ± 7.89a |
28.6 ± 0.57b |
5 |
292.9 ± 1.61b |
−28.1 ± 0.31c |
6 |
311.8 ± 9.43a |
−35.4 ± 0.47d |
7 |
328.6 ± 7.01ab |
−43.4 ± 0.60e |
Ionic strength |
0.1 M |
346.0 ± 6.46a |
−39.7 ± 0.30a |
0.5 M |
354.0 ± 5.56a |
−34.1 ± 0.33b |
1.0 M |
357.9 ± 9.60a |
−31.6 ± 0.68b |
The determination of pH value is a very important factor for the emulsion stability because of chemical reactions that compromise the quality of the product. The pH of emulsions prepared with oils was decreased due to the hydrolysis of fatty acid esters into free fatty acids.37 As presented in Table 2, the mean particle size and zeta potential was increased from 292.9 ± 1.61 to 328.6 ± 7.01 nm and −28.1 ± 0.31 to −43.4 ± 0.60 mV with the increase in pH value from 5 to 7, while decreased in the pH value from 4 to 3, the particle size and zeta potential was increased from 338.5 ± 7.89 to 340.9 ± 8.04 nm and 28.6 ± 0.57 to 40.9 ±0.46 mV, respectively. Liu and co-workers (2008)38 reported that the size of casein molecules increased with increasing pH values from 6.0 to 12.0. The individual casein molecules become more negatively charged and separate from each other due to electrostatic repulsion which results in looser casein molecules and contributes towards the increase in the droplet size of casein at an alkaline pH. In addition, the size of the particles was decreased towards acidic pH and may result in compact structured casein molecules. In the case of the zeta potentials, particles at pH 3 have a positive net charge compared to at pH 7, due to the fact that the surface charges on the NaCas shift to the positive side under acidic conditions.
In the food and cosmetic industries, salts are used as additives. Salts are also present in the form of bile salts in the gastrointestinal tract. So, they affect the functional properties of the emulsion. In this regard, different salt concentrations (0.1 to 1.0 M NaCl) were used in the present study (Table 2). We observed that the mean particle size of the curcumin nanoemulsion was slightly increased with an increase in the ionic concentration without any significant difference. The zeta potential of the prepared nanoemulsion was decreased with a change in the ionic strength from −39.7 ± 0.30 mV at 0.1 M to −31.6 ± 0.68 mV at 1.0 M. Srinivasan et al. (2000),39 found that with emulsions prepared with 1 and 3% NaCas before or after emulsification, there was no difference in the particle size with a 0 to 1 M NaCl concentration. On the contrary, the droplet diameter decreased with the addition of a 0 to 20 mM concentration of NaCl to the prepared emulsion with calcium caseinate.40
3.2.4. Encapsulation efficiency.
The encapsulation efficiency of the curcumin nanoemulsion was determined by means of the total phenolic content and found to be 96.9 ± 0.28%. The high encapsulation of curcumin may be due to better entrapment in the hydrophobic core and NaCas as a hydrophilic environment. Several researchers reported that the encapsulation efficiency was found to be >85%, 83% and 97% for curcumin zein, casein and poly lactide-co-glycolide (PLGA) nanoemulsions, respectively.41–43
3.2.5.
In vitro release of curcumin under simulated digestion.
The release of curcumin from oil in water curcumin nanoemulsions was determined by SGF and SIF methods (Table 3). In SGF, after 2 hours, only a 5% release of curcumin was observed. This is due to the resistance of NaCas to pepsin action and was followed by a 16% release in intestinal fluid after 2.5 hours digestion. This finding was in agreement with studies showing the biphasic release of encapsulated curcumin with glycerol monooleate that found a 46% release over 24 hours and a sustained release of about 66% after 10 days.44 Similarly, PLGA loaded curcumin nanoparticles also exhibited a biphasic releasing pattern and a 59% release occurred after 12 hours, increasing to 89% at the end of 6 days.45 The slow release of lipophilic curcumin upon digestion in the intestine is incorporated into the bile salts or phospholipids (mixed micelles). These mixed micelles along with the curcumin then enter into the systemic circulation through enterocytes present in the intestinal cell membrane.46
Table 3 Release of curcumin from nanoemulsion under simulated gastro-intestinal digestion
Time (h) |
Gastric digestion (% release) |
Intestinal digestion (% release) |
Value mentioned is the mean ± S.E.M (n = 3). |
0 |
3.46 ± 0.12 |
10.94 ± 0.21 |
1 |
4.69 ± 0.43 |
13.36 ± 0.25 |
2 |
5.25 ± 0.34 |
15.33 ± 0.35 |
2.5 |
— |
16.12 ± 0.42 |
3.2.6. Morphological characterization using scanning electron microscopy.
As shown in Fig. 4, the surface morphology of the freeze dried O/W curcumin nanoemulsion was confirmed by SEM observation. It is evident from SEM that the particles are spherical and have a smooth surface structure. The nanoemulsions which are spherical in shape will easily enter into the cells as compared to the rod shape structures.
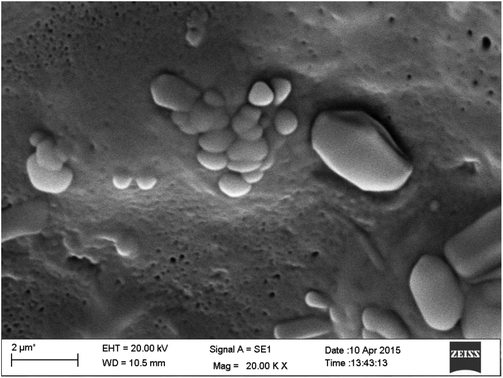 |
| Fig. 4 Scanning electron micrograph of the curcumin nanoemulsion. | |
3.3. Sensory attributes of curcumin encapsulated into ice cream
Our formulation was successfully incorporated into the ice cream mix and the sensory parameters viz., colour & appearance, body & texture, melting quality and flavour were evaluated. No significant difference was observed between the control (without curcumin) and encapsulated curcumin nanoemulsion for all the sensory attributes (Table 4). However, a significant difference (p < 0.05) was observed in the encapsulation efficiency before and after the preparation of the ice cream, found to be 96% and 93%, respectively.
Table 4 Sensory acceptability of ice cream incorporated with nanoemulsion of curcumin
Characteristics |
Control (A) |
Encapsulated curcumin (B) |
Values mentioned are the means ± S.E.M (n = 8). Mean values within a row with unlike superscript letters differ significantly (p < 0.05). |
Colour and appearance |
7.6 ± 0.31a |
7.6 ± 0.20a |
Body and texture |
8.1 ± 0.18a |
7.4 ± 0.26a |
Melting quality |
7.7 ± 0.30a |
7.6 ± 0.35a |
Flavour |
7.7 ± 0.38a |
7.4 ± 0.43a |
4. Conclusion
Curcumin is a natural polyphenolic compound with wide therapeutic potential. The bioactive component (curcumin) can be incorporated into food systems for the development of health promoting functional foods. In the present study, the nanoencapsulation of lipophilic curcumin by using NaCas as an emulsifier was found to be stable under different processing conditions such as heat, pH and ionic strength. The release kinetics data also suggest that our formulation was stable in simulated gastro-intestinal conditions. Further, ice cream was selected as an ideal food system for the delivery of the curcumin nanoemulsion and was found to be stable under different processing conditions. In future, more studies are warranted in this field to study the functional attributes and use for therapeutic purposes.
Conflict of Interest
All authors declare that there is no conflict of interest.
Acknowledgements
We would like to thank the Director of the ICAR-National Dairy Research Institute, Haryana (India), for providing the necessary facilities.
References
- T. Arun and P. K. Tiku, Food Chem., 2012, 130, 960–965 CrossRef PubMed.
- A. Goel, A. B. Kunnumakkara and B. B. Aggarwal, Biochem. Pharmacol., 2008, 75, 787–809 CrossRef CAS PubMed.
- S. P. Weisberg, R. Leibel and D. V. Tortoriello, Endocrinology, 2008, 149, 3549–3558 CrossRef CAS PubMed.
- P. Anand, A. B. Kunnumakkara, R. A. Newman and B. B. Aggarwal, Mol. Pharm., 2007, 4, 807–818 CrossRef CAS PubMed.
- R. C. Srimal, Fitoterapia, 1997, 68, 483–493 Search PubMed.
- G. K. Jayaprakasha, L. Jagan Mohan Rao and K. K. Sakariah, Trends Food Sci. Technol., 2005, 16, 533–548 CrossRef CAS PubMed.
- P. Rojsitthisak, Y. Limpanon, N. Thipmongkolsilp, B. Kongtong and J. Wongtavatchai, Thai J. Pharm. Sci., 2005, 29, 165–177 Search PubMed.
- P. Anand, S. G. Thomas, A. B. Kunnumakkara, C. Sundaram, K. B. Harikumar, B. Sung, S. T. Tharakan, K. Misra, I. K. Priyadarsini, K. N. Rajasekharan and B. B. Aggarwal, Biochem. Pharmacol., 2008, 76, 1590–1611 CrossRef CAS PubMed.
- A. L. Cheng, C. H. Hsu, J. K. Lin, M. M. Hsu, Y. F. Ho, T. S. Shen, J. Y. Ko, J. T. Lin, B. R. Lin, W. Ming-Shiang, H. S. Yu, S. H. Jee, G. S. Chen, T. M. Chen, C. A. Chen, M. K. Lai, Y. S. Pu, M. H. Pan, Y. J. Wang, C. C. Tsai and C. Y. Hsieh, Anticancer Res., 2001, 21, 2895–2900 CAS.
- P. Bourassa, C. D. Kanakis, P. Tarantilis, M. G. Pollissiou and H. A. Tajmir-Riahi, J. Phys. Chem. B, 2010, 114, 3348–3354 CrossRef CAS PubMed.
- J. S. Mandeville, E. Froehlich and H. A. Tajmir-Riahi, J. Pharm. Biomed. Anal., 2009, 49, 468–474 CrossRef CAS PubMed.
- F. Mohammadi, A. K. Bordbar, A. Divsalar, K. Mohammadi and A. Saboury, Protein J., 2009, 28, 117–123 CrossRef CAS PubMed.
- A. Liu, H. Lou, L. Zhao and P. Fan, J. Pharm. Biomed. Anal., 2006, 40, 720–727 CrossRef CAS PubMed.
- R. Shelma and C. P. Sharma, J. Mater. Sci. Mater. Med., 2010, 21, 2133–2140 CrossRef CAS PubMed.
- K. Baglole, P. Boland and B. Wagner, J. Photochem. Photobiol., A, 2005, 173, 230e237 CrossRef PubMed.
- C. P. Shah, B. Mishra, M. Kumar, K. I. Priyadarsini and P. N. Bajaj, Curr. Sci., 2008, 95, 1426e–11432 Search PubMed.
- A. Sahu, N. Kasoju and U. Bora, Biomacromolecules, 2008, 9, 2905e–22912 CrossRef PubMed.
- T. P. Sari, B. Mann, R. Kumar, R. R. B. Singh, R. Sharma, B. Minaxi and S. Athira, Food Hydrocolloids, 2015, 43, 540–546 CrossRef CAS PubMed.
- S. Kotta, A. W. Khan, K. Pramod, S. H. Ansari, R. K. Sharma and J. Ali, Expert Opin. Drug Delivery, 2012, 9, 585 CrossRef CAS PubMed.
- R. Parveen, S. Baboota, J. Ali, A. Ahuja, S. S. Vasudev and S. Ahmed, Int. J. Pharm., 2011, 413, 245 CrossRef CAS PubMed.
- I. Kralova and J. Sjoblom, J. Dis. Sci. Technol., 2009, 30, 1363–1383 CrossRef CAS PubMed.
- N. Anton, J. P. Beniot and P. Saulnier, J. Controlled Release, 2008, 128, 185 CrossRef CAS PubMed.
-
C. Solans, J. Esquena, A. Forgiarini, P. Izquierdo, D. Morales, N. Uson, N. Azemar and M. J. Garcia-Celma, in Surfactant Science Series, ed. K. L. Mittal, D. Shah and M. Dekker, 2002 Search PubMed.
- S. Ghasemi and S. Abbasi, Food Hydrocolloids, 2014, 42–47 CrossRef CAS PubMed.
-
D. M. Mulvihill, Casein and caseinates, ed. P. F. Fox, Elsevier, London, 1989, pp. 97–130 Search PubMed.
- S. Jafari, Y. He and B. Bhandari, J. Food Eng., 2007, 82, 478–488 CrossRef PubMed.
- P. G. Dalev and L. S. Simenova, J. Sci. Food Agric., 1995, 68, 203–206 CrossRef CAS PubMed.
- S. Surassmo, S. Min, P. Bejrapha and M. Cho, J. Food Res. Int., 2010, 43, 8–17 CrossRef CAS PubMed.
- W. Zheng and S. Y. Wang, J. Agric. Food Chem., 2001, 49, 5165–5170 CrossRef CAS PubMed.
- B. Sánchez, M. Fernández-García, A. Margolles, C. G. de los Reyes-Gavilán and P. Ruas-Madiedo, Int. Dairy J., 2010, 20, 800–805 CrossRef PubMed.
- L. Liang, X. Wu, T. Zhao, J. Zhao, F. Li, Y. Zou, G. Mao and L. Yang, Food Res. Int., 2012, 46, 76–82 CrossRef CAS PubMed.
- H. Stone, H. Sidel, S. Oliver, A. Woolsey and R. C. Singleton, Food Technol., 1974, 28, 24–34 Search PubMed.
- K. Ahmed, Y. Li, J. D. McClements and H. Xiao, Food Chem., 2012, 132, 799–807 CrossRef CAS PubMed.
- M. Srinivasan, H. Singh and P. A. Munro, Food Hydrocolloids, 2002, 16, 153–160 CrossRef CAS.
- M. Li, Y. Ma and J. Cui, Food Sci. Technol., 2014, 59, 49–58 CAS.
- M. Srinivasan, H. Singh and P. A. Munro, Food Chem., 2003, 80, 61–69 CrossRef CAS.
- D. S. Bernardi, T. A. Pereira, N. R. Maciel, J. Bortoloto, G. S. Viera, G. S. Oliveira and P. A. Rocha-Filho, J. Nanobiotechnol., 2011, 9, 44 CrossRef CAS PubMed.
- Y. Liu and R. Guo, Biophys. Chem., 2008, 136, 67–73 CrossRef CAS PubMed.
- M. Srinivasan, H. Singh and P. A. Munro, Food Hydrocolloids, 2000, 14, 497–507 CrossRef CAS.
- A. Ye, M. Srinivasan and H. Singh, Food Chem., 2000, 69, 237–244 CrossRef CAS.
- J. Gomez-Estaca, M. P. Balaguer, R. Gavara and P. Hernandez-Munoz, Food Hydrocolloids, 2012, 28, 82–91 CrossRef CAS PubMed.
- A. Patel, Y. Hu, J. K. Tiwariand and K. P. Velikov, Soft Matter, 2010, 6, 6192–6199 RSC.
- P. Anand, H. B. Nair, B. Sung, A. B. Kunnumakkara, V. R. Yadav, R. R. Tekmal and B. B. Aggarwal, Biochem. Pharmacol., 2010, 79, 330–338 CrossRef CAS PubMed.
- C. Mohanty and S. K. Sahoo, Biomaterials, 2010, 31, 6597–6611 CrossRef CAS PubMed.
- Y. M. Tsai, C. F. Chien, L. C. Lin and T. H. Tsai, Int. J. Pharm., 2011, 416, 331–338 CrossRef CAS PubMed.
- D. J. McClements and H. Xiao, Food Funct., 2012, 3, 202–220 CAS.
|
This journal is © The Royal Society of Chemistry 2016 |
Click here to see how this site uses Cookies. View our privacy policy here.