DOI:
10.1039/C5QO00348B
(Research Article)
Org. Chem. Front., 2016,
3, 145-149
Rapid access to bicyclic δ-lactones via carbene-catalyzed activation and cascade reaction of unsaturated carboxylic esters†
Received
4th November 2015
, Accepted 3rd December 2015
First published on 3rd December 2015
Abstract
Carboxylic esters are an excellent choice of substrates in organic synthesis. Here we demonstrate that carbene-catalyzed activation of α,β-unsaturated ester can be developed to synthesize sophisticated multi-cyclic molecules in a single step cascade process. The iridoid-type lactone products are obtained with high stereo-selectivities, and can readily undergo further transformations.
Introduction
Iridoids are a class of secondary metabolites found in a wide variety of plants and in some animals.1 This class of molecules and their natural or synthetic analogs usually consist of a cyclopentane ring fused to a six-membered oxygen heterocycle (Fig. 1a). Significantly, these molecules have been found to exhibit useful bioactivity, such as a neuroprotective effect,2a and anti-cancer,2b anti-tumour,2c anti-inflammatory,2d anti-oxidant,2e and anti-bacterial2f activity. Considerable effort has been spent in the synthesis of this class of molecules via both a biosynthetic approach3 and organic synthesis.4,5 However, the majority of methods involve relatively long synthetic steps, sometimes harsh conditions and often result in racemic products.5 Our laboratory is interested in catalytically activating readily available substrates for rapid (ideally single-step) asymmetric synthesis of functional molecules.6 We have demonstrated that stable esters7 and α,β-unsaturated esters8 can be activated by carbene organic catalysts for efficient and selective reactions.9 Here we report a single step access to optically enriched iridoids by using our catalytic ester activation approach (Fig. 1b). Mechanistically, the reaction starts via the addition of a carbene catalyst to an α,β-unsaturated ester followed by a cascade process10,11 involving two Michael additions and a lactonization step. The bicyclic products were obtained in excellent yields, diastereoselectivities and enantioselectivities. It is of special note that during the preparation of the manuscript Ye11a and Studer11b groups independently reported similar cascade reactions using enals as the substrates via oxidative NHC catalysis.
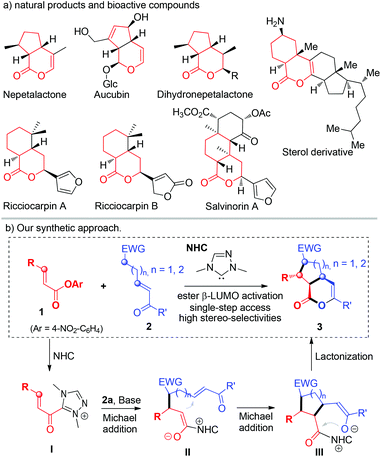 |
| Fig. 1 (a) Iridoid derivatives in natural products, (b) our synthetic approach. Detailed proposed mechanism and stereochemical modes are provided in the ESI† (page S7) (Glc = β-glucopyranosyl; EWG = electron-withdrawing group). | |
Results and discussion
We started by using unsaturated ester 1a and enone malonate 2a as model substrates to search for suitable conditions, as shown in Table 1. Achiral triazolium pre-catalyst A12 in the presence of DBU as a base and LiCl13,14 as an additive could mediate this reaction to afford the proposed cascade product 3a in 90% (entry 1) yield. The use of chiral triazolium pre-catalyst (e.g.B15) based on the scaffold of A led to 3a with poor er (entry 2). We also found that catalyst C16 could promote this reaction with much improved er (entry 3). Replacing of the two hydrogen substituents in C with methyl units (catalyst D17) could enhance the product er from 77
:
23 to 90
:
10, albeit with a lower yield (entry 4). The low yield in the reactions (entries 2–4) was due to the competing ester hydrolysis. We reasoned that increasing the nucleophilicity of the carbene catalyst could likely promote the desired reaction between the ester and the carbene. Thus we next replaced the methyl substituents on the N-mesityl group of catalysts D with methoxyl units to give catalyst E.18 By using catalyst E with DABCO (0.3 equivalent) and DBU (1.0 equivalent) as the base, the reaction proceeded smoothly to give 3a in 88% yield, 11
:
1 dr and 91
:
9 er (entry 5). Replacing the methyl units in E with ethyl substituents (to give catalyst F) could further improve the reaction stereoselectivities (entry 6). The reaction yield (with catalyst F) could be further improved by using a catalytic amount of HOBt as an additive without affecting the product er (85% yield, 12
:
1 dr, 96
:
4 er; entry 7).
Table 1 Screening of reaction conditions for the reaction of 1a with 2aa
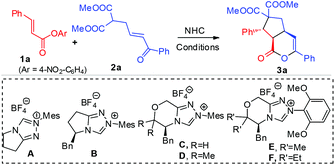
|
Entry |
NHC |
Yieldb [%] |
drb |
erc |
Standard conditions: NHC precursor (20 mol%), 1a (0.1 mmol), 2a (0.1 mmol), DBU (1.0 equiv.), LiCl (1.0 equiv.), THF (1.0 mL), 4 Å MS, rt, 3 h.
Yields and diastereomeric ratio of 3a, determined via1H NMR analysis of unpurified reaction mixtures. 1,3,5-Trimethoxybenzene was used as an internal standard. Absolute configuration of product was determined via X-ray of 4o (Table 3).
Determined via chiral phase HPLC analysis.
0.3 eq. DABCO was added, then 1.0 eq. DBU was added after 20 min.
0.2 eq. HOBt was added and 1.2 eq. 2a and 1.4 eq. DBU were used instead.
Isolated yield in parenthesis. DBU = 1,8-diazabicyclo[5.4.0]undec-7-ene; Mes = mesityl (2,4,6-trimethylphenyl); DABCO = 1,4-diazabicyclo[2.2.2]octane; HOBt = Hydroxybenzotriazole.
|
1 |
A
|
90 |
20 : 1 |
— |
2 |
B
|
55 |
20 : 1 |
57 : 43 |
3 |
C
|
43 |
10 : 1 |
77 : 23 |
4 |
D
|
25 |
12 : 1 |
90 : 10 |
5d |
E
|
88 |
11 : 1 |
91 : 9 |
6d |
F
|
74 |
12 : 1 |
96 : 4 |
7d,e |
F
|
92 (85)f |
12 : 1 |
96 : 4 |
With the acceptable conditions in hand (Table 1, entry 7), we next studied the generality of the ester substrate in our reaction by using β-enone malonate 2a as a model substrate (Table 2). Installation of various substituents (1b–h) at the para-carbon of the beta-phenyl group of unsaturated ester 1 was all tolerated, except the strong electron-releasing group (CH3O, 1b) where a lower yield was observed under standard conditions. Placing the substituent at different positions of the β-phenyl group of 1 has no apparent effect on the reaction outcome (3e and 3h). The β-phenyl unit of 1 could be replaced by naphthathyl (1i) or heteroaryl (1j–k) units. The β-aryl unit of unsaturated ester could also be replaced by an alkenyl (1l) or ester (1m) substituent. Esters with the β-alkyl substituent (1n) and acetic ester (1o) could also undergo the desired reactions, albeit with a dropped yield and er (Table 1).
Table 2 Scope of ester 1a
Conditions as in Table 1, entry 7; isolated yields were based on ester 1.
The reaction at 2.0 mmol scale gave 86% yield, 12 : 1 dr, 96 : 4 er.
20% yield, 71 : 29 er was obtained using the indanol derived NHC in Ye's11a work.
|
|
The scope of the β-enone malonate was then evaluated (Table 3). Different (hetero)aryl substituents on the enone part were all tolerated (4a–h). Remarkably, substituting on the α-carbon of the enone part did not affect the reaction outcome (4i–j). The tolerance of substituent on the enone α-carbon allows us to prepare the multi-cyclic steroid derivative194j in 76% yield, 10
:
1 dr and 97
:
3 er. Moreover, enone derived with the alkyl group (Me) can also give a good result (4m). Not surprisingly, the two methyl alcohol ester groups of the malonate substrates could be changed to ethyl (4n) or benzyl (4o) alcohol ester units.
Table 3 Scope of β-enone malonate 2a
Conditions as in Table 1, entry 7; yields (after SiO2 chromatography purification) were based on ester 1.
Reactions run with 30 mol% NHC and 1.6 equivalents of enone.
|
|
Our strategy of forming the bicyclic products (3 and 4) with fused five and six-membered rings could be directly extended to prepare fused six and six-ring products. When γ-enone malonate 5 was used to react with the unsaturated ester substrate, the fused bicyclic product 6 could be obtained with excellent yield, and er under the above conditions without further optimizations (Table 4). Interestingly, γ-nitro enone was also an effective substrate, leading to products 6e–g bearing the synthetically useful nitro group. Notably, the use of the related β-nitro enone substrate could not give the corresponding five-membered ring product due to the elimination of HNO2 (to form the corresponding γ,δ-unsaturated enone) under basic conditions. The reaction of γ-nitro enone was performed at −78 °C to give 6e in 87
:
13 er (99
:
1 er after a single recrystallization).
Table 4 Scope of ester 1 and enone 4a
Conditions as in Table 1, entry 7; yields (after SiO2 chromatography purification) were based on ester 1.
Reactions run at −78 °C.
After one recrystallization.
Reactions run with 30 mol% NHC and 1.5 equivalents of ester. Yield (after SiO2 chromatography purification) was based on enone 5.
|
|
The optically enriched products from our catalytic reactions could undergo further transformations under simple conditions. For example, the enol ester carbon–carbon double bond of 3a could be reduced to give 7 or oxidized to give 8, bearing a significant core structure in many biologically active natural products.20 The nitro group of 6g could be removed to give 9 (with a ring opening ester exchange of the lactone) (Scheme 1).
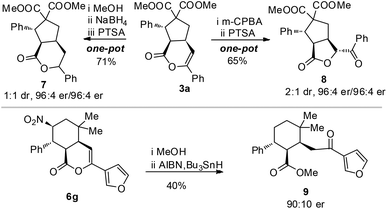 |
| Scheme 1 Synthetic transformations of the cascade product. | |
Conclusions
In summary, we have developed a highly efficient single-step approach for the preparation of multi-cyclic lactone products with excellent optical purity. Our reactions proceed through carbene-catalyzed activation of unsaturated esters as the key step, followed by a highly selective cascade process. The multi-cyclic products from our catalytic reaction can readily undergo further transformations. Our study demonstrates the synthetic power in turning readily available and stable ester substrates to sophisticated products via an extremely short route, and will encourage further development and application of carbene catalysis and ester activation.
Experimental
A dry Schlenk tube with a stir bar was charged with ester 1 (0.10 mmol, 1.0 equiv.), NHC F (9.9 mg, 20 mol%), LiCl (5.0 mg, 1.0 equiv.), HOBt (2.7 mg, 20 mol%), DABCO (3.4 mg, 30 mol%) and molecular sieves (50 mg). The tube was evacuated, and refilled with nitrogen. Then the enone 2 (0.12 mmol, 1.2 equiv.) was added and the mixture was dissolved with the newly distilled solvent THF (1.0 mL). After stirring at rt for 20 min, DBU (21 μL, 1.4 equiv.) was added. Then the mixture was continued to be stirred at room temperature for another 3 h when the substrate was consumed completely (monitored by TLC). The mixture was concentrated under vacuum and purified by column chromatography on silica gel (hexane/EtOAc) to afford the desired product 3 as yellow oil.
[α]23D (c 1.70, CHCl3) = −16.8; 1H NMR (400 MHz, CDCl3): δ = 7.59 (dd, J1 = 8.0 Hz, J2 = 2.4 Hz, 2 H); 7.24–7.39 (m, 8 H); 5.56 (d, J = 3.2 Hz, 1 H); 4.70 (d, J = 8.8 Hz, 1 H); 3.71 (s, 3 H); 3.52–3.61 (m, 2 H); 3.13 (m, 4 H); 2.27 (dd, J1 = 12.0 Hz, J2 = 4.0 Hz, 1 H). 13C NMR (100 MHz, CDCl3): δ = 171.6, 170.2, 168.4, 149.2, 137.3, 132.2, 129.2, 128.7, 128.5, 128.3, 127.8, 124.8, 102.2, 65.1, 53.7, 53.1, 52.3, 47.6, 41.3, 36.7 ppm. IR (film): νmax 2952, 1731, 1515, 1425, 1272, 1211, 1008 cm−1. HRMS (ESI): C24H23O6 [M + H]+ calcd: 407.1495, found: 407.1492; 96
:
4 er as determined by HPLC (Chiralcel IB, 90
:
10 hexanes/i-PrOH, 0.7 mL min−1), tr (major) = 13.4 min, tr (minor) = 16.6 min.
Acknowledgements
We acknowledge the support from Singapore's National Research Foundation (NRF), the Ministry of Education (MOE), Nanyang Technological University (NTU), and China's National Key Program for Basic Research (No. 2010CB 126105), the Thousand Talent Plan, the National Natural Science Foundation (No. 21132003; No. 21472028), the Guizhou Province Returned Overseas Student Science and Technology Activity Program, the Department of Science and Technology of Guizhou Province and Guizhou University.
Notes and references
-
(a) L. J. Elnaggar and J. L. Beal, J. Nat. Prod., 1980, 43, 649–707 CrossRef CAS PubMed;
(b) E. L. Ghisalberti, Phytomedicine, 1998, 5, 147–163 CrossRef CAS PubMed;
(c) B. Dinda, S. Debnath and Y. Harigaya, Chem. Pharm. Bull., 2007, 55, 159–222 CrossRef CAS PubMed;
(d) B. Dinda, S. Debnath and R. Banik, Chem. Pharm. Bull., 2011, 59, 803–833 CrossRef CAS PubMed.
-
(a) J. Xu, P. Zhao, Y. Q. Guo, C. F. Xie, D. Q. Jin, Y. G. Ma, W. B. Hou and T. J. Zhang, Fitoterapia, 2011, 82, 1133–1136 CrossRef CAS PubMed;
(b) S. Pandeti, K. Sharma, S. R. Bathula and N. Tadigoppula, Phytomedicine, 2014, 21, 333–339 CrossRef CAS PubMed;
(c) H. K. Hamdi and R. Castellon, Biochem. Biophys. Res. Commun., 2005, 334, 769–778 CrossRef CAS PubMed;
(d) W. J. Zhu, M. Q. Pang, L. Y. Dong, X. Y. Huang, S. M. Wang and L. L. Zhou, Life Sci., 2012, 91, 369–376 CrossRef CAS PubMed;
(e) E. J. Jeong, K. Y. Lee, S. H. Kim, S. H. Sung and Y. C. Kim, Eur. J. Pharmacol., 2008, 588, 78–84 CrossRef CAS PubMed;
(f) M. Modaressi, A. Delazar, H. Nazemiyeh, F. Fathi-Azad, E. Smith, M. M. Rahman, S. Gibbons, L. Nahar and S. D. Sarker, Phytother. Res., 2009, 23, 99–103 CrossRef CAS PubMed.
- F. Geu-Flores, N. H. Sherden, V. Courdavault, V. Burlat, W. S. Glenn, C. Wu, E. Nims, Y. H. Cui and S. E. O'Connor, Nature, 2012, 492, 138–142 CrossRef CAS PubMed.
-
(a) E. M. Phillips, M. Wadamoto, A. Chan and K. A. Scheidt, Angew. Chem., Int. Ed., 2007, 46, 3107–3110 CrossRef CAS PubMed;
(b) Y. Li, X. Q. Wang, C. Zheng and S. L. You, Chem. Commun., 2009, 5823–5825 RSC;
(c) L. Candish and D. W. Lupton, Org. Lett., 2010, 12, 4836–4839 CrossRef CAS PubMed;
(d) D. Belmessieri, L. C. Morrill, C. Simal, A. M. Z. Slawin and A. D. Smith, J. Am. Chem. Soc., 2011, 133, 2714–2720 CrossRef CAS PubMed;
(e) A. Biswas, S. De Sarkar, R. Frohlich and A. Studer, Org. Lett., 2011, 13, 4966–4969 CrossRef CAS PubMed.
-
(a) S. H. Lim, M. D. Curtis and P. Beak, Org. Lett., 2001, 3, 711–714 CrossRef CAS PubMed;
(b) A. Srikrishna and M. S. Rao, Tetrahedron Lett., 2002, 43, 151–154 CrossRef CAS;
(c) T. I. Richardson, J. A. Dodge, G. L. Durst, L. A. Pfeifer, J. Shah, Y. Wang, J. D. Durbin, V. Krishnan and B. H. Norman, Bioorg. Med. Chem. Lett., 2007, 17, 4824–4828 CrossRef CAS PubMed;
(d) J. H. Joo and S. W. Youn, Adv. Synth. Catal., 2013, 355, 559–568 CAS;
(e) B. Zhou, Z. Luo and Y. C. Li, Chem. – Eur. J., 2013, 19, 4428–4431 CrossRef CAS PubMed;
(f) X. W. Fan and Y. Cheng, Org. Biomol. Chem., 2014, 12, 123–131 RSC.
-
(a) J. M. Mo, L. Shen and Y. R. Chi, Angew. Chem., Int. Ed., 2013, 52, 8588–8591 CrossRef CAS PubMed;
(b) J. M. Mo, R. J. Yang, X. K. Chen, B. Tiwari and Y. R. Chi, Org. Lett., 2013, 15, 50–53 CrossRef CAS PubMed;
(c) M. Wang, Z. J. Huang, J. F. Xu and Y. R. Chi, J. Am. Chem. Soc., 2014, 136, 1214–1217 CrossRef CAS PubMed;
(d) T. S. Zhu, P. C. Zheng, C. L. Mou, S. Yang, B. A. Song and Y. R. Chi, Nat. Commun., 2014, 5, 5027 CrossRef CAS PubMed;
(e) Y. X. Zhang, Y. Du, Z. J. Huang, J. F. Xu, X. X. Wu, Y. H. Wang, M. Wang, S. Yang, R. D. Webster and Y. R. Chi, J. Am. Chem. Soc., 2015, 137, 2416–2419 CrossRef CAS PubMed.
-
(a) L. Hao, Y. Du, H. Lv, X. Chen, H. Jiang, Y. Shao and Y. R. Chi, Org. Lett., 2012, 14, 2154–2157 CrossRef CAS PubMed;
(b) L. Hao, S. Chen, J. Xu, B. Tiwari, Z. Fu, T. Li, J. Lim and Y. R. Chi, Org. Lett., 2013, 15, 4956–4959 CrossRef CAS PubMed;
(c) L. Hao, C. W. Chuen, R. Ganguly and Y. R. Chi, Synlett, 2013, 1197–1200 CAS;
(d) Z. Fu, J. Xu, T. Zhu, W. W. Y. Leong and Y. R. Chi, Nat. Chem., 2013, 5, 835–839 CrossRef CAS PubMed;
(e) S. Chen, L. Hao, Y. Zhang, B. Tiwari and Y. R. Chi, Org. Lett., 2013, 15, 5822–5825 CrossRef CAS PubMed;
(f) Z. Fu, K. Jiang, T. S. Zhu, J. Torres and Y. R. Chi, Angew. Chem., Int. Ed., 2014, 53, 6506–6510 CrossRef CAS PubMed.
-
(a) J. Cheng, Z. Huang and Y. R. Chi, Angew. Chem., Int. Ed., 2013, 52, 8592–8596 CrossRef CAS PubMed;
(b) J. Xu, Z. Jin and Y. R. Chi, Org. Lett., 2013, 15, 5028–5031 CrossRef CAS PubMed; Also see:
(c) S. J. Ryan, L. Candish and D. W. Lupton, J. Am. Chem. Soc., 2009, 131, 14176–14177 CrossRef CAS PubMed.
- For selected examples:
(a) B. E. Maki, A. Chan, E. M. Phillips and K. A. Scheidt, Org. Lett., 2007, 9, 371–374 CrossRef CAS PubMed;
(b) J. Guin, S. De Sarkar, S. Grimme and A. Studer, Angew. Chem., Int. Ed., 2008, 47, 8727–8730 CrossRef CAS PubMed;
(c) B. E. Maki and K. A. Scheidt, Org. Lett., 2008, 10, 4331–4334 CrossRef CAS PubMed;
(d) S. De Sarkar, S. Grimme and A. Studer, J. Am. Chem. Soc., 2010, 132, 1190–1191 CrossRef CAS PubMed;
(e) S. De Sarkar and A. Studer, Angew. Chem., Int. Ed., 2010, 49, 9266–9269 CrossRef CAS PubMed;
(f) Z. Q. Rong, M. Q. Jia and S. L. You, Org. Lett., 2011, 13, 4080–4083 CrossRef CAS PubMed;
(g) F. G. Sun, L. H. Sun and S. Ye, Adv. Synth. Catal., 2011, 353, 3134–3138 CrossRef CAS;
(h) B. Wanner, J. Mahatthananchai and J. W. Bode, Org. Lett., 2011, 13, 5378–5381 CrossRef CAS PubMed;
(i) A. G. Kravina, J. Mahatthananchai and J. W. Bode, Angew. Chem., Int. Ed., 2012, 51, 9433–9436 CrossRef CAS PubMed;
(j) J. Mahatthananchai, J. Kaeobamrung and J. W. Bode, ACS Catal., 2012, 2, 494–503 CrossRef CAS PubMed;
(k) R. C. Samanta, B. Maji, S. De Sarkar, K. Bergander, R. Frohlich, C. Muck-Lichtenfeld, H. Mayr and A. Studer, Angew. Chem., Int. Ed., 2012, 51, 5234–5238 CrossRef CAS PubMed;
(l) S. De Sarkar, A. Biswas, R. C. Samanta and A. Studer, Chem. – Eur. J., 2013, 19, 4664–4678 CrossRef CAS PubMed;
(m) J. Mahatthananchai and J. W. Bode, Acc. Chem. Res., 2014, 47, 696–707 CrossRef CAS PubMed;
(n) Z. J. Wu, F. Y. Li and J. Wang, Angew. Chem., Int. Ed., 2015, 54, 1629–1633 CrossRef CAS PubMed. For selected recent reviews on NHC catalysis, also see:
(o) X. Bugaut and F. Glorius, Chem. Soc. Rev., 2012, 41, 3511–3522 RSC;
(p) S. J. Ryan, L. Candish and D. W. Lupton, Chem. Soc. Rev., 2013, 42, 4906–4917 RSC;
(q) J. Mahatthananchai and J. W. Bode, Acc. Chem. Res., 2014, 47, 696–707 CrossRef CAS PubMed;
(r) D. M. Flanigan, F. R. Michailidis, N. A. White and T. Rovis, Chem. Rev., 2015, 15, 9307–9387 CrossRef PubMed.
- For reviews, see: C. Grondal, M. Jeanty and D. Enders, Nat. Chem., 2010, 2, 167–178 CrossRef CAS PubMed . For selected examples of NHC-catalyzed cascade reactions, see:
(a) V. Nair, S. Vellalath, M. Poonoth and E. Suresh, J. Am. Chem. Soc., 2006, 128, 8736–8737 CrossRef CAS PubMed;
(b) P. C. Chiang, J. Kaeobamrung and J. W. Bode, J. Am. Chem. Soc., 2007, 129, 3520–3521 CrossRef CAS PubMed;
(c) M. He and J. W. Bode, J. Am. Chem. Soc., 2008, 130, 418–419 CrossRef CAS PubMed;
(d) B. Cardinal-David, D. E. A. Raup and K. A. Scheidt, J. Am. Chem. Soc., 2010, 132, 5345–5347 CrossRef CAS PubMed;
(e) D. T. Cohen, B. Cardinal-David and K. A. Scheidt, Angew. Chem., Int. Ed., 2011, 50, 1678–1682 CrossRef CAS PubMed;
(f) E. Sanchez-Larios, J. M. Holmes, C. L. Daschner and M. Gravel, Synthesis, 2011, 1896–1904 CAS;
(g) L. Candish and D. W. Lupton, J. Am. Chem. Soc., 2013, 135, 58–61 CrossRef CAS PubMed;
(h) L. Candish, C. M. Forsyth and D. W. Lupton, Angew. Chem., Int. Ed., 2013, 52, 9149–9152 CrossRef CAS PubMed;
(i) Z. J. Wu, X. Wang, F. Y. Li, J. C. Wu and J. Wang, Org. Lett., 2015, 17, 3588–3591 CrossRef CAS PubMed.
-
(a) Z. Q. Liang, D. L. Wang, H. M. Zhang and S. Ye, Org. Lett., 2015, 17, 5140–5143 CrossRef CAS PubMed;
(b) S. Bera, C. G. Daniliuc and A. Studer, Org. Lett., 2015, 17, 4940–4943 CrossRef CAS PubMed.
- K. B. Ling and A. D. Smith, Chem. Commun., 2011, 47, 373–375 RSC.
- In the absence of LiCl, the product was formed with 49% NMR yield.
- For selective studies using Lewis acids as additives in NHC catalysis, see:
(a) B. Cardinal-David, D. E. A. Raup and K. A. Scheidt, J. Am. Chem. Soc., 2010, 132, 5345–5347 CrossRef CAS PubMed;
(b) D. E. A. Raup, B. Cardinal-David, D. Holte and K. A. Scheidt, Nat. Chem., 2010, 2, 766–771 CrossRef CAS PubMed;
(c) A. M. ElSohly, D. A. Wespe, T. J. Poore and S. A. Snyder, Angew. Chem., Int. Ed., 2013, 52, 5789–5794 CrossRef CAS PubMed;
(d) J. Qi, X. G. Xie, R. F. Han, D. H. Ma, J. Yang and X. G. She, Chem. – Eur. J., 2013, 19, 4146–4150 CrossRef CAS PubMed;
(e) S. Bera, R. C. Samanta, C. G. Daniliuc and A. Studer, Angew. Chem., Int. Ed., 2014, 53, 9622–9626 CrossRef CAS PubMed;
(f) H. Y. Dang, Z. T. Wang and Y. Cheng, Org. Lett., 2014, 16, 5520–5523 CrossRef CAS PubMed;
(g) X. Zhao, D. A. DiRocco and T. Rovis, J. Am. Chem. Soc., 2011, 133, 12466–12469 CrossRef CAS PubMed;
(h) J.-L. Li, B. Sahoo, C.-G. Daniliuc and F. Glorius, Angew. Chem., Int. Ed., 2014, 53, 10515–10519 CrossRef CAS PubMed.
- P.-C. Chiang, M. Rommel and J. W. Bode, J. Am. Chem. Soc., 2009, 131, 8714–8718 CrossRef CAS PubMed.
- I. Piel, M. Steinmetz, K. Hirano, R. Frohlich, S. Grimme and F. Glorius, Angew. Chem., Int. Ed., 2011, 50, 4983–4987 CrossRef CAS PubMed.
- C. G. Goodman, M. M. Walker and J. S. Johnson, J. Am. Chem. Soc., 2015, 137, 122–125 CrossRef CAS PubMed.
- F. Liu, X. Bugaut, M. Schedler, R. Frohlich and F. Glorius, Angew. Chem., Int. Ed., 2011, 50, 12626–12630 CrossRef CAS PubMed . Related effects for NHC E, see:
(a) M. Schedler, R. Frohlich, C. G. Daniliuc and F. Glorius, Eur. J. Org. Chem., 2012, 4164–4171 CrossRef CAS;
(b) C. Guo, B. Sahoo, C. G. Daniliuc and F. Glorius, J. Am. Chem. Soc., 2014, 136, 17402–17405 CrossRef CAS PubMed.
-
(a) J. S. R. Hanson, Nat. Prod. Rep., 2010, 27, 887–899 RSC;
(b) J. P. Munafo Jr. and T. J. Gianfagna, Nat. Prod. Rep., 2015, 32, 454–477 RSC;
(c) A. Ali, M. Asif, H. Khanam, A. Mashrai, M. A. Sherwani, M. Owais and S. Shamsuzzaman, RSC Adv., 2015, 5, 75964–75984 RSC.
-
(a) R. Bandichhor, B. Nosse and O. Reiser, Top. Curr. Chem., 2005, 243, 43–72 CAS;
(b) R. R. A. Kitson, A. Millemaggi and R. J. K. Taylor, Angew. Chem., Int. Ed., 2009, 48, 9426–9451 CrossRef CAS PubMed;
(c) Y. Li and G. Pattenden, Nat. Prod. Rep., 2011, 28, 429–440 RSC;
(d) S. Lee, J. H. Jang, G.-S. Hwang and D. H. Ryu, J. Org. Chem., 2013, 78, 770–775 CrossRef CAS PubMed.
Footnotes |
† Electronic supplementary information (ESI) available: Experimental and spectra details. CCDC 1404951. For ESI and crystallographic data in CIF or other electronic format see DOI: 10.1039/c5qo00348b |
‡ These authors contributed equally. |
|
This journal is © the Partner Organisations 2016 |
Click here to see how this site uses Cookies. View our privacy policy here.