DOI:
10.1039/C6QO00045B
(Research Article)
Org. Chem. Front., 2016,
3, 603-608
Palladium-catalyzed ring contraction reaction of naphthoquinones upon reaction with alkynes†
Received
29th January 2016
, Accepted 4th March 2016
First published on 7th March 2016
Abstract
An unprecedented palladium catalysed ring contraction reaction of naphthoquinones with alkynes has been developed. A wide range of phthalides are synthesized in good to high yields and with high regioselectivities. These synthesized phthalides are versatile synthetic intermediates toward many other useful synthetic building blocks.
Introduction
The significance of phthalide skeletons1 is recognized by their wide distribution in natural products and medicines with a wide range of biological activities,2 from enhancing flavors to slowing the memory loss.2a In particular, phthalide-containing polymers possess outstanding electrophysical and optical characteristics and high heat resistance.3 Although diverse in functions, these phytochemicals share a common structure: a benzene ring fused to a γ-lactone. Significant synthetic efforts have been made to construct phthalides,4 but most of them involved non-catalytic multistep synthesis.2a–d,5,6 Toward addressing this challenge, herein we report an efficient and complementary route to a new class of phthalides with a fulvene moiety at the C3 position (Scheme 1a) by catalytic ring contraction and multi-component annulation (Scheme 1c).
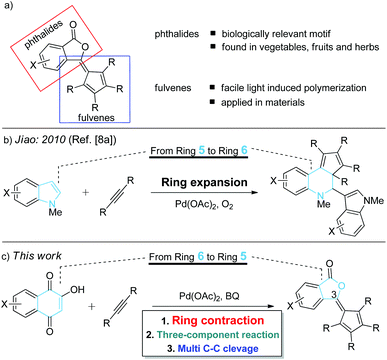 |
| Scheme 1 (a) Structure of phthalide–fulvene complex; (b) palladium-catalyzed ring expansion reaction of alkynes; (c) palladium-catalyzed ring contraction reaction of alkynes. | |
It should be noted that fulvene, an isomer of benzene, is known to undergo facile light- or heat-induced polymerization.7 Although the Jiao group8a reported an elegant example of the palladium-catalyzed ring-expansion8 reaction of indoles with alkynes (Scheme 1b), to the best of our knowledge, the example of ring contraction reactions of naphthoquinones with alkynes has not yet been disclosed. This chemistry not only offers a new approach to functionalized phthalides, but also provides valuable mechanistic insights into this novel ring contraction reaction.9
Recently, we have developed the direct annulation reaction of simple oxindoles or coumarins with internal alkynes to generate benzazepines10a or spirocyclopentadienes,10e respectively, catalyzed by palladium.10,11 Our continued efforts in metal-catalyzed C–H functionalization and alkyne chemistry12 prompted us to explore the reaction of naphthoquinones and alkynes. Our study began with evaluation of reaction conditions for the model reactions of 2-hydroxyl-1,4-naphthoquinone 1a with diphenylacetylene 2a in the presence of AgOAc as an oxidant (Table 1, entries 1–4). First, Pd(OAc)2 and Pd(TFA)2 were found to facilitate the generation of the desired product 3a (entries 1 and 3, 47% and 38%, respectively). Next, Pd(OAc)2 as an effective catalyst was selected for further investigation. After extensive screening of a large array of oxidants (entries 5–13), we found that the addition of 2.0 equiv. of 1,4-benzoquinone (BQ) played a pivotal role for a higher reaction conversion (entry 13, 65%). After further surveying a variety of reaction media, the best results were obtained using a mixed solvent of MeCN/HOAc (v/v = 3/1) (entry 14). The effects of temperature are summarized in Table 1 (entries 15 and 16), which indicated that an increased temperature did not significantly improve the chemical yield (entry 15, 120 °C, 79%). However, lowering the temperature led to a slow conversion (entry 16, 53%). Although the catalyst loading could reduce to 5 mol%, a long reaction time was required (entry 17, 73%, 96 h).
Table 1 Palladium-catalyzed ring-contraction reactions of 1a with 2aa
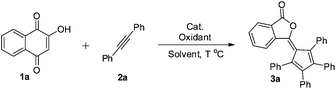
|
Entry |
Catalyst |
Oxidant |
T (°C) |
Yieldb (%) |
Reaction conditions: MeCN (2 mL), 1a (0.2 mmol, 1.0 equiv.), 2a (1.0 mmol, 3.0 equiv.), 10 mol% catalyst, oxidant (0.4 mmol, 2.0 equiv.) at 100 °C, and 24 h.
MeCN/HOAc = 3 : 1 (v/v) as the solvent.
BQ = 1,4-benzoquinone.
5 mol% catalyst loading and 96 h.
|
1 |
Pd(OAc)2 |
AgOAc |
100 |
47 |
2 |
Pd(PPh3)2Cl2 |
AgOAc |
100 |
<10 |
3 |
Pd(TFA)2 |
AgOAc |
100 |
38 |
4 |
PdCl2 |
AgOAc |
100 |
<10 |
5 |
Pd(OAc)2 |
AgSbF6 |
100 |
<10 |
6 |
Pd(OAc)2 |
FeCl2 |
100 |
<10 |
7 |
Pd(OAc)2 |
Fe(acac)3 |
100 |
No reaction |
8 |
Pd(OAc)2 |
CuCl |
100 |
<10 |
9 |
Pd(OAc)2 |
Cu(OAc)2 |
100 |
21 |
10 |
Pd(OAc)2 |
PhI(OAc)2 |
100 |
No reaction |
11 |
Pd(OAc)2 |
K2S2O8 |
100 |
No reaction |
12 |
Pd(OAc)2 |
(NH4)2S2O8 |
100 |
No reaction |
13 |
Pd(OAc)2 |
BQ |
100 |
65 |
14
|
Pd(OAc)
2
|
BQ
|
100
|
81
|
15b |
Pd(OAc)2 |
BQ |
120 |
79 |
16b |
Pd(OAc)2 |
BQ |
80 |
53 |
17b,d |
Pd(OAc)2 |
BQ |
100 |
73
|
With the optimal conditions in hand, we examined the scope of 2-hydroxyl-1,4-naphthoquinone 1. Table 2 illustrates the substitution pattern of 2-hydroxyl-1,4-naphthoquinones. Electron-withdrawing, neutral and electron-donating substituents at the C-8 position were tolerated and gave moderate to high yields (3a–g). However, electron-withdrawing substituents led to slight diminishment in the chemical yield (3e–g). Substrates with electron-withdrawing or electron-donating substituents at both the C-7 and C-8 positions also showed moderate to good yields (Table 2, 3h–j).
Reaction conditions: HOAc/MeCN = 1 : 3 (v/v, 2 mL), 1 (0.2 mmol, 1.0 equiv.), 2 (1.0 mmol, 3.0 equiv.), Pd(OAc)2 (10 mol%), BQ (2.0 equiv.), and 100 °C.
Combined yield of regioisomers.
The ratio of regioisomers is determined by crude NMR and listed in brackets.
|
|
In addition, these optimal conditions were compatible with a wide range of symmetric diaryl internal alkyne substrates (Table 2). Diphenylacetylene derivatives worked well and afforded phthalides 3k–3w. Specifically, alkynes bearing an electron-withdrawing group (F, Cl) or an electron-donating group (Me, tBu, OMe) were successfully employed in this reaction. It was noteworthy that halogen and silicon functionalities remained intact after reaction completion, thus offering flexibility for other valuable manipulations. In the event that unsymmetric internal alkynes (2r–2t) were tested, two major isomers were regularly formed by following Markovnikov's rule in both alkyne additions (3r–3t).13 The minor isomer formed differed from the major isomer as a result of anti-Markovnikov addition in the second alkyne insertion step.14 Exceptionally, when unsymmetric diaryl alkyne (2u) reacted with 1a, 3u was formed as the only major isomer in high regioselectivity (rr > 19
:
1) due to the potential π–π stacking of two naphthalene rings. Alkynyl esters (2v, 2w) were also applicable to this protocol and provided major isomers through a Markovnikov–anti-Markovnikov alkyne addition sequence (3v and 3w, rr > 19
:
1).15 However, alkyl alkyne (2x) exhibited poor reactivity in this kind of ring contraction reaction (3x).
Transformations of the present phthalides were briefly examined as shown in Scheme 2. Treatment of 3a with NaOH in ethanol/H2O furnished cyclopentadiene 4 in high yield. The FeCl3-catalyzed cleavage reaction of 3a in the presence of NaOMe furnished tetraphenylcyclopentadienone 5, which was structurally characterized by single crystal X-ray diffraction analysis.16 Under epoxidation conditions (m-CPBA), 3a converted into unanticipated functionalized 1,4-diol 6 in 51% yield, which was also structurally confirmed by X-ray diffraction analysis.173a could also be reacted in the presence of pyridinium chlorochromate (PCC) to construct cyclohexanone fused cyclopentadiene 7.18 Notably, 3a could be smoothly converted into densely functionalized cyclopentadiene 8 in the presence of NaN3.19
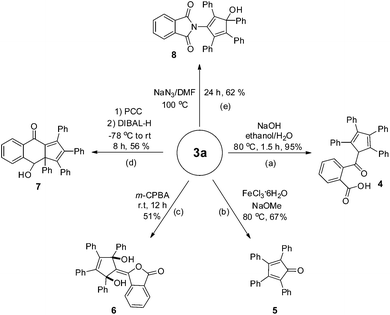 |
| Scheme 2 Synthetic transformations of 3a. Reaction conditions: (a) 3a (0.2 mmol), NaOH (10 mol L−1, 0.4 mL), ethanol/H2O (2.0 mL, v/v = 1 : 1), 80 °C, 1 h, 95%; (b) 3a (0.1 mmol), FeCl3·6H2O (0.2 mmol), NaOMe (0.2 mmol), EtOH (2.0 mL), 80 °C, 24 h, 67%; (c) 3a (0.1 mmol), m-CPBA (0.2 mmol), DCM (2.0 mL), r.t., 0.5 h, 51%; (d) 3a (0.1 mmol), DIBAL-H (0.2 mmol), NaOMe (0.06 mmol), DCM (3.0 mL), −78 °C to r.t., 3 h, PCC (0.3 mmol), r.t. 12 h, 56%; (e) 3a (0.1 mmol), NaN3 (0.5 mmol), 100 °C, 24 h, 62%. | |
On the basis of the known ring contraction reaction, we proposed a plausible mechanism to account for this chemical process (Scheme 3). The envisioned catalytic cycle would be initiated by an intermolecular insertion of 2-hydroxyl-1,4-naphthalenedione 1b to diphenyl acetylene 2a in the presence of Pd(OAc)2. The subsequent carbopalladation of second diphenyl acetylene 2a gives vinylpalladium species 11, which then undergoes intramolecular annulation leading to a palladacycle 12, followed by reduction elimination. A final pericyclic cheletropic rearrangement of intermediate 13 would deliver the phthalide structure and liberate CO gas.20 Furthermore, Pd(0) is reoxidized to Pd(II) by the oxidant BQ, thus closing the catalytic cycle. To ascertain whether the anticipated spiro cyclopentadiene 13 is formed in this process, we designed an experiment to obtain the plausible spiro intermediate. In view of the typical cyclic keto–enol tautomerism structure of 1a, a similar molecule 14 was finally selected as the replacement and applied to react with diphenyl acetylene 2a. Gratifyingly, the desired spiro cyclopentadiene product 1521 was formed by the reaction of 4-hydroxylcoumarin 14 and diphenyl acetylene 2a, which is in agreement with the obtained X-ray single crystal structure (eqn (1)). Furthermore, two mechanistically insightful results based on 13C-labeling experiments were obtained (eqn (2) and (3)). First, 13C-labeled (at C–4) 2-hydroxyl-1,4-naphthalenedione 1a′ was used to react with diphenyl acetylene 2a, resulting in 13C-labeled 3a′a (eqn (2), δ = 151.66 ppm, single peak).22 Second, 13C-labeled (at C–3 and C–4) 2-hydroxy-1,4-naphthalenedione 1a′′ was used to react with diphenyl acetylene 2a, leading to the 13C-labeled 3a′′a (eqn (3), δ1 = 151.19 ppm, double peak; δ2 = 129.50 ppm, double peak). These two 13C-labeled experimental results indicate that the C-3 and C-4 from starting material 1a are utilized to assemble the desired product through the construction of the new C
C bond (eqn (3)). These data are also in agreement with the proposed mechanism, which suggests the liberation of CO based on C-2 carbon.
| 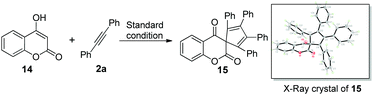 | (1) |
| 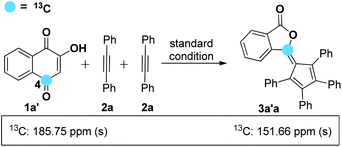 | (2) |
| 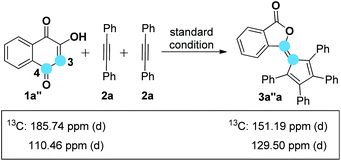 | (3) |
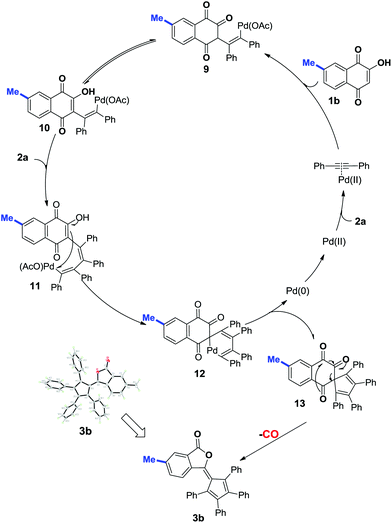 |
| Scheme 3 Proposed mechanism. | |
Conclusions
In conclusion, the ability to transform common starting materials into synthetic useful products in catalytic annulation reactions offers a widely useful tool in the late-stage diversification of molecules and the generation of compound library. In this study, we have developed new palladium-catalyzed ring contraction reactions which allow 2-hydroxyl-1,4-naphthoquinones to convert into various phthalides. The significance of phthalide and fulvene scaffolds as structural units should render this method attractive for both medicinal chemistry and synthetic chemistry, paving the way for efficient synthesis of other complex cyclic systems. Studies of additional transformations exploiting this underexplored activation mode of alkynes and synthetic applications of these methods are under way.
Acknowledgements
The project described was supported by a grant from the Tsinghua University, the “Thousand Plan” Youth program of China, the Bayer Investigator Fellowship, and the Tsinghua-Peking centre for life sciences (CLS).
Notes and references
-
(a) J. J. Beck and S.-C. Chou, J. Nat. Prod., 2007, 70, 891 CrossRef CAS PubMed
;
(b) J. Zhou, K. Gupta, J. Yao, K. Ye, D. Panda, P. Giannakakaou and H. C. Joshi, J. Biol. Chem., 2002, 277, 39777 CrossRef CAS PubMed
;
(c) B. Witulski, A. Zimmermann and N. D. Gowans, Chem. Commun., 2002, 2984 RSC
;
(d) U. Holler, J. B. Gloer and D. T. Wicklow, J. Nat. Prod., 2002, 65, 876 CrossRef PubMed
;
(e) K. Kobayashi, K. Maeda, T. Uneda, O. Morikawa and H. Konishi, J. Chem. Soc., Perkin Trans. 1, 1997, 443 RSC
.
-
(a)
G. Lin, S. S.-K. Chan, H.-S. Chung and S.-L. Li, Studies in Natural Products Chemistry, ed. A. Rahman, Elsevier, Amsterdam, 2005, vol. 32, pp. 611–671 Search PubMed
;
(b) K. Yoganathan, C. Rossant, S. Ng, Y. Huang, M. S. Butler and A. D. Buss, J. Nat. Prod., 2003, 66, 1116 CrossRef CAS PubMed
;
(c) F. O. Shode, A. S. Mahomed and C. B. Rogers, Phytochemistry, 2002, 61, 955 CrossRef CAS PubMed
;
(d) J. A. Palermo, M. V. R. Brasco, C. Spagnuolo and A. M. Seldes, J. Org. Chem., 2000, 65, 4482 CrossRef CAS PubMed
;
(e) C. Puder, A. Zeeck and W. Beil, J. Antibiot., 2000, 53, 329 CrossRef CAS PubMed
;
(f) G. Strobel, E. Ford, J. Worapong, J. K. Harper, A. M. Arif, D. M. Grant, P. C. W. Fung and R. M. W. Chau, Phytochemistry, 2002, 60, 179 CrossRef CAS PubMed
;
(g) D. Mal and P. Pahari, Chem. Rev., 2007, 107, 1892 CrossRef CAS PubMed
.
- Y. I. Puzin, T. V. Chebaeva, E. I. Galinurova, R. R. Muslukhov, Y. B. Monakov and A. M. Syrkin, Russ. J. Org. Chem., 2004, 40, 1129 CrossRef CAS
.
- For selected examples, see:
(a) M. Kosaka, S. Sekiguchi, J. Naito, M. Uemura, S. Kuwahara, M. Watanabe, N. Harada and K. Hiroi, Chirality, 2005, 17, 218 CrossRef CAS PubMed
;
(b) R. Pedrosa, S. Sayalero and M. Vincente, Tetrahedron, 2006, 62, 10400 CrossRef CAS
;
(c) P. V. Ramachandran, G.-M. Chen and H. C. Brown, Tetrahedron Lett., 1996, 37, 2205 CrossRef CAS
;
(d) K. Knepper, R. E. Ziegert and S. Brase, Tetrahedron, 2004, 60, 8591 CrossRef CAS
;
(e) M. Kitamura, T. Okhuma, S. Inoue, N. Sayo, H. Kumobayshi, S. Akutagawa, H. Takaya and R. Noyori, J. Am. Chem. Soc., 1998, 110, 629 CrossRef
;
(f) B. Witulski and A. Zimmermann, Synlett, 2002, 1855 CrossRef CAS
;
(g) K. Tanaka, G. Nishida, A. Wada and K. Noguchi, Angew. Chem., Int. Ed., 2004, 43, 6510 CrossRef CAS PubMed
;
(h) H. C. Chang, M. Jeganmohan and C.-H. Cheng, Chem. – Eur. J., 2007, 13, 4356 CrossRef CAS PubMed
;
(i) K. Everaere, A. Mortreux and J.-F. Carpentier, Adv. Synth. Catal., 2003, 345, 67 CrossRef CAS
.
-
(a) S. Hayat, A. Rahman, M. I. Choudhary, K. M. Khan and E. Bayer, Tetrahedron Lett., 2001, 42, 1647 CrossRef CAS
;
(b) J. D. Hall, N. W. Duncan-Gould, N. A. Siddiqi, J. N. Kelly, L. A. Hoeferlin, S. J. Morrisonb and J. K. Wyatt, Bioorg. Med. Chem., 2005, 13, 1409 CrossRef CAS PubMed
;
(c) R. Pedrosa, S. Sayalero and M. Vicente, Tetrahedron, 2006, 62, 10400 CrossRef CAS
.
- For examples of transition metal catalyzed phthalide synthesis, see:
(a) M. Kuriyama, N. Ishiyama, R. Shimazawa, R. Shirai and O. Onomura, J. Org. Chem., 2009, 74, 9210 CrossRef CAS PubMed
;
(b) H. T. Chang, M. Jeganmohan and C. H. Cheng, Chem. – Eur. J., 2007, 13, 4356 CrossRef CAS PubMed
;
(c) D. H. T. Phan, B. Kim and V. M. Dong, J. Am. Chem. Soc., 2009, 131, 15608 CrossRef CAS PubMed
;
(d) M. C. Willis, Angew. Chem., Int. Ed., 2010, 49, 6026 CrossRef CAS PubMed
;
(e) S. Omura, T. Fukuyama, Y. Murakami, H. Okamoto and I. Ryu, Chem. Commun., 2009, 6741 RSC
;
(f) B. Zhang, M.-H. Xu and G. Qiang, Org. Lett., 2009, 11, 4712 CrossRef CAS PubMed
;
(g) L. Ackermann and J. Pospech, Org. Lett., 2011, 13, 4153 CrossRef CAS PubMed
;
(h) X. Shi and C.-J. Li, Adv. Synth. Catal., 2012, 16, 2933 CrossRef
;
(i) Y. Lian, R. G. Bergman and J. A. Ellman, Chem. Sci., 2012, 3, 3088 RSC
.
-
(a) R. D. Brown, F. R. Burden and J. E. Kent, J. Chem. Phys., 1968, 49, 5542 CrossRef CAS
;
(b) E. D. Bergmann, Chem. Rev., 1968, 68, 41 CrossRef CAS
;
(c)
M. Neuenschwander, in The Chemistry of Double-Bonded Functional Groups, ed. S. Patai, Wiley, Weinheim, 1989, pp. 1131–1268 Search PubMed
;
(d)
P. Yates, Advanced Alicyclic Chemistry, Academic, New York, NY, 1968, vol. 2, pp. 59–184 Search PubMed
;
(e) J. H. Day, Chem. Rev., 1963, 53, 167 CrossRef
;
(f)
K. P. Zeller, Pentafulvenes in Methoden der Organischen Chemie, 1985, vol. 5/2c, pp. 504–684 Search PubMed
. For selected recent examples, see:
(g) C. S. Bryan and M. Lautens, Org. Lett., 2010, 12, 2754 CrossRef CAS PubMed
;
(h) S. Ye, X. Yang and J. Wu, Chem. Commun., 2010, 2950 RSC
;
(i) S. M. Abdur Rahman, M. Sonoda, M. Ono, K. Miki and Y. Tobe, Org. Lett., 2006, 8, 1197 CrossRef CAS PubMed
;
(j) M. Schmittel and C. Vavilala, J. Org. Chem., 2005, 70, 4865 CrossRef CAS PubMed
;
(k) S. V. Kovalenko, S. Peabody, M. Manoharan, R. J. Clark and I. V. Alabugin, Org. Lett., 2004, 6, 2457 CrossRef CAS PubMed
;
(l) T. Bekele, C. F. Christian, M. A. Lipton and D. A. Singleton, J. Am. Chem. Soc., 2005, 127, 9216 CrossRef CAS PubMed
;
(m) N. J. Clegg, S. Paruthiyil, D. C. Leitman and T. S. Scanlan, J. Med. Chem., 2005, 48, 5989 CrossRef CAS PubMed
;
(n) H. Gao, J. A. Katzenellenbogen, R. Garg and C. Hansch, Chem. Rev., 1999, 99, 723 CrossRef CAS PubMed
;
(o) H. Ottosson, K. Kilså, K. Chajara, M. C. Piqueras, R. Crespo, H. Kato and D. Muthas, Chem. – Eur. J., 2007, 13, 6998 CrossRef CAS PubMed
. For catalytic fulvene synthesis, see:
(p) G. Wu, A. L. Rheingold, S. J. Geib and R. F. Heck, Organometallics, 1987, 6, 1941 CrossRef CAS
;
(q) G. C. M. Lee, B. Tobias, J. M. Holmes, D. A. Harcourt and M. E. Garst, J. Am. Chem. Soc., 1990, 112, 9330 CrossRef CAS
;
(r) L. J. Silverberg, G. Wu, A. L. Rheingold and R. F. Heck, J. Organomet. Chem., 1991, 409, 411 CrossRef CAS
;
(s) E. Negishi, L. S. Harring, Z. Owczarczyk, M. M. Mohamud and M. Ay, Tetrahedron Lett., 1992, 33, 3253 CrossRef CAS
;
(t) E. S. Johnson, G. J. Balaich, P. E. Fanwick and I. P. Rothwell, J. Am. Chem. Soc., 1997, 119, 11086 CrossRef CAS
;
(u) U. Radhakrishnan, V. Gevorgyan and Y. Yamamoto, Tetrahedron Lett., 2000, 41, 1971 CrossRef CAS
;
(v) M. Kotora, H. Matsumura, G. Gao and T. Takahashi, Org. Lett., 2001, 3, 3467 CrossRef CAS PubMed
;
(w) C. N. Iverson and W. D. Jones, Organometallics, 2001, 20, 5745 CrossRef CAS
;
(x) M. Uemura, Y. Takayama and F. Sato, Org. Lett., 2004, 6, 5001 CrossRef CAS PubMed
;
(y) F. W. Patureau, T. Besset, N. Kuhl and F. Glorius, J. Am. Chem. Soc., 2011, 133, 2154 CrossRef CAS PubMed
;
(z) Y. Shibata and K. Tanaka, Angew. Chem., Int. Ed., 2011, 50, 10917 CrossRef CAS PubMed
.
-
(a) Z. Shi, B. Zhang, Y. Cui and N. Jiao, Angew. Chem., Int. Ed., 2010, 49, 4036 CrossRef CAS PubMed
;
(b) M. Mentel and R. Breinbauer, Curr. Org. Chem., 2005, 9, 109 CrossRef
;
(c) G. L. Closs and G. M. Schwartz, J. Org. Chem., 1961, 26, 2609 CrossRef CAS
.
- For selected books and reviews on ring contraction reactions, see:
(a)
D. Redmore and C. D. Gutsche, in Advances in Alicyclic Chemistry, ed. H. Hart and G. J. Karabastos, Academic Press, New York and London, 1971, vol. 3, p. 1 Search PubMed
;
(b) L. F. Silva Jr., Tetrahedron, 2002, 58, 9137 CrossRef
;
(c) R. M. Scribner, Org. React. Steroid Chem., 1972, 2, 408 CAS
;
(d) L. F. Silva Jr., Synlett, 2014, 466 CrossRef
;
(e) H. Redlich, Angew. Chem., Int. Ed. Engl., 1994, 33, 1345 CrossRef
;
(f) I. Dubovyk, D. Pichugin and A. K. Yudin, Angew. Chem., Int. Ed., 2011, 50, 5924 CrossRef CAS PubMed
.
- For examples of our recently developed palladium or ruthenium catalyzed cross-coupling reactions of alkynes, see:
(a) L. Wang, J. Y. Huang, S. Y. Peng, H. Liu, X.-F. Jiang and J. Wang, Angew. Chem., Int. Ed., 2013, 52, 1768 CrossRef CAS PubMed
;
(b) L. Wang, S. Y. Peng and J. Wang, Chem. Commun., 2011, 47, 5422 RSC
;
(c) S. Y. Peng, L. Wang and J. Wang, Chem. – Eur. J., 2013, 19, 13322 CrossRef CAS PubMed
;
(d) S. Y. Peng, L. Wang, J. Y. Huang, S. F. Sun, H. B. Guo and J. Wang, Adv. Synth. Catal., 2013, 355, 2550 CrossRef CAS
;
(e) S. Y. Peng, T. Gao, S. F. Sun, M. H. Wu, H. B. Guo and J. Wang, Adv. Synth. Catal., 2014, 356, 319 CrossRef CAS
;
(f) J. C. Wu, W.-B. Xu, Z.-X. Yu and J. Wang, J. Am. Chem. Soc., 2015, 137, 9489 CrossRef CAS PubMed
.
- For selected recent reviews on C–H functionalizations, see:
(a) C. Zhu, R. Wang and J. R. Falck, Chem. – Asian J., 2012, 7, 1502 CrossRef CAS PubMed
;
(b) G. Song, F. Wang and X. Li, Chem. Soc. Rev., 2012, 41, 3651 RSC
;
(c) J. F. Hartwig, Chem. Soc. Rev., 2011, 40, 1992 RSC
;
(d) J. Wencel-Delord, T. Dröge, F. Liu and F. Glorius, Chem. Soc. Rev., 2011, 40, 4740 RSC
;
(e) L. Ackermann, Chem. Rev., 2011, 111, 1315 CrossRef CAS PubMed
;
(f) R. Jazzar, J. Hitce, A. Renaudat, J. Sofack-Kreutzer and O. Baudoin, Chem. – Eur. J., 2010, 16, 2654 CrossRef CAS PubMed
;
(g) T. Satoh and M. Miura, Chem. – Eur. J., 2010, 16, 11212 CrossRef CAS PubMed
;
(h) D. A. Colby, R. G. Bergman and J. A. Ellman, Chem. Rev., 2010, 110, 624 CrossRef CAS PubMed
;
(i)
J.-Q. Yu and Z.-J. Shi, Topics in Current Chemistry, C–H Activation, Springer, Berlin, 2010, vol. 292 Search PubMed
;
(j) C.-J. Li, Acc. Chem. Res., 2009, 42, 335 CrossRef CAS PubMed
;
(k) I. V. Seregin and V. Gevorgyan, Chem. Soc. Rev., 2007, 36, 1173 RSC
;
(l) K. R. Campos, Chem. Soc. Rev., 2007, 36, 1069 RSC
.
- For recent reviews of palladium catalyzed heterocyclic synthesis using alkynes, see: R. Chinchilla and C. Nájera, Chem. Rev., 2014, 114, 1783 CrossRef CAS PubMed
, and references therein.
- Ratio of regioisomers (rr) was determined by crude NMR.
- The assignment of major isomers was referred in Tanaka's report [ref. 7a]: Y. Shibata and K. Tanaka, Angew. Chem., Int. Ed., 2011, 50, 10917 CrossRef CAS PubMed
, and our previous ref. 10a.
- CCDC 975848 (the structure of compound 3w was determined by X-ray crystal analysis) contains the supplementary crystallographic data for this paper.
- CCDC 975850 (the structure of compound 5 was determined by X-ray crystal analysis) contains the supplementary crystallographic data for this paper.
- CCDC 925572 (the structure of compound 6 was determined by X-ray crystal analysis) contains the supplementary crystallographic data for this paper.
- CCDC 915903 (the structures of compound 7 were determined by X-ray crystal analysis) contains the supplementary crystallographic data for this paper.
- CCDC 925571 (the structures of compound 8 were determined by X-ray crystal analysis) contains the supplementary crystallographic data for this paper.
- CCDC 975849 (the structures of compound 3b were determined by X-ray crystal analysis) contains the supplementary crystallographic data for this paper.
- CCDC 875492 (the structures of compound 15 were determined by X-ray crystal analysis) contains the supplementary crystallographic data for this paper.
- For the details of 13C-labeled experiments and NMR data, see the ESI.†.
|
This journal is © the Partner Organisations 2016 |
Click here to see how this site uses Cookies. View our privacy policy here.