DOI:
10.1039/C6QO00313C
(Research Article)
Org. Chem. Front., 2016,
3, 1430-1434
Iodine-promoted sequential dual oxidative Csp3–H amination/Csp3–H iodination reactions: efficient synthesis of 1-iodoimidazo[1,5-a]pyridines†
Received
29th June 2016
, Accepted 29th August 2016
First published on 30th August 2016
Abstract
An iodine-promoted sequential dual oxidative Csp3–H amination of aryl methyl ketones and Csp3–H iodination of pyridin-2-ylmethylamines have been realized for the first time. This simple and efficient approach constructed 1-iodoimidazo[1,5-a]pyridines in moderate to good yields. Based on the preliminary experimental results, a self-sequenced process featuring the iodination/Kornblum oxidation/dual amination/oxidation/iodination mechanism was proposed.
Direct oxidative Csp3–H amination has received intensive research interest since it represents an atom-economical strategy to build a C–N bond.1,2 Among them, transition-metal-catalyzed oxidative amination of Csp3–H to access C–N bonds has been well established.3 In parallel, the development of metal-free Csp3–H amination, especially an iodine/iodide-mediated dual Csp3–H-amination toward the synthesis of nitrogen-containing compounds including quinazolines and quinazolinones,4 has recently captured intensive attention of organic chemists.5 As a typical example of nitrogen-containing compounds, imidazo[1,5-a]pyridines have drawn much attention for their wide applications in both materials chemistry and the pharmaceutical industry.6 Besides the traditional Vilsmeier-type cyclizations,7 the direct oxidative Csp3–H amination/cyclization strategy has been developed for efficient and atom-economical synthesis of imidazo[1,5-a]pyridines. Notably, an NBS/TBHP or copper-catalyzed oxidative amination of Csp3–H bonds for the synthesis of imidazo[1,5-a]-pyridines under ambient conditions has been described by Wang,8a Adimurthy8b and Zhu8c (Scheme 1a). Moreover, Zeng,9a Xu9b and Wang9c have independently presented the copper-catalyzed direct construction of imidazo[1,5-a]-pyridines from N-heteroaryl aldehydes or ketones with alkylamines via Csp3–H amination (Scheme 1b). Recently, an efficient copper-catalyzed aerobic oxidative synthesis of imidazo[1,5-a]pyridines has also been developed by Adimurthy (Scheme 1c).10 Despite these advances, direct dual Csp3–H amination via employing alkylamines and N-heteroarenes as nitrogen sources to sequentially couple with the same sp3-carbon has not been developed for the construction of imidazo[1,5-a]-pyridines. Herein, we describe our progress in the I2-mediated sequential dual Csp3–H amination of aryl methyl ketones with pyridin-2-ylmethylamines to build the imidazo[1,5-a]pyridine skeleton (Scheme 1d).
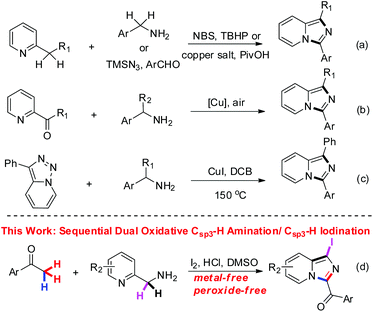 |
| Scheme 1 Direct Csp3–H amination for the construction of imidazo[1,5-a]pyridines. | |
On the basis of our previous studies on nitrogen heterocycle synthesis,11 we initially envisioned that the sp3-carbon of acetophenone could be iodinated to give the corresponding α-iodoketone in the presence of I2. The latter would then be converted into the corresponding phenylglyoxal by Kornblum oxidation. Subsequently, it would further react with pyridin-2-ylmethylamine, followed by sequential amination and iodination, to give 1-iodoimidazo[1,5-a]pyridine (Scheme 2). Remarkably, this strategy represents the first example of iodine-mediated sequential dual amination of Csp3–H of aryl methyl ketones and Csp3–H iodination of pyridin-2-ylmethylamines to build 1-iodoimidazo[1,5-a]pyridines, in which pyridin-2-ylmethylamines were used as dual nitrogen donors.
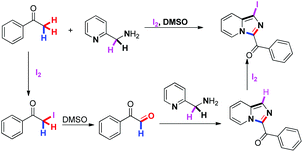 |
| Scheme 2 Design strategy: sequential dual amination of Csp3–H of methyl ketone with pyridin-2-ylmethanamine. | |
To test the above hypothesis, our initial efforts focused on the reaction of acetophenone 1a (1.0 mmol) with pyridin-2-ylmethylamine 2a (1.0 mmol) in the presence of molecular iodine as a model reaction. This reaction afforded the corresponding annulation product 3a in 63% yield in DMSO at 100 °C for 5 h (Table 1, entry 1). It should be noted that aryl iodides are broadly utilized to construct complex structures in organic synthesis via transition metal-catalyzed coupling reactions (such as Suzuki coupling/Negishi coupling, and Buchwald/Hartwig amination/amidation).12 Encouraged by the results, we further optimized the reaction conditions in order to improve the yield of the target product. Initially, studies indicated that an increase or decrease in the equivalents of 2a had a significant influence on the yield (Table 1, entries 2–4). The influence of temperature on the reaction was also investigated, and 110 °C was determined as the optimal temperature for the reaction (Table 1, entries 5–8). Decreasing the I2 dosage greatly decreased the yield (Table 1, entries 9–11). Subsequently, a series of Brønsted and Lewis acids, such as HCl, CF3SO3H, TsOH·H2O, FeCl3 and Zn(OTf)2, were screened for the reaction. To our satisfaction, protic acids could effectively promote the reaction and hydrochloric acid was the best promoter for this reaction (Table 1, entries 12–16). Based on the above experimental results, various equivalents of hydrochloric acid were screened and 1.0 equivalent of hydrochloric acid was the best choice. After screening different parameters, the optimal reaction conditions were determined as 1a (1.0 mmol) with 2a (1.0 mmol) in the presence of I2 (1.6 mmol) and HCl (1.0 mmol) in DMSO at 110 °C for 5 h.
Table 1 Optimization of reaction conditionsa
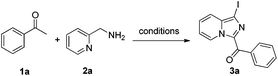
|
Entry |
2a (equiv.) |
Iodine (equiv.) |
Temp. (°C) |
Acid (mmol) |
Yieldb (%) |
Reaction conditions: 1a (1.0 mmol), 2a, I2, and acid (1.0 mmol) heated in 3 mL of DMSO within 5 h.
Isolated yield.
|
1 |
1.0 |
1.6 |
100 |
|
63 |
2 |
0.5 |
1.6 |
100 |
|
45 |
3 |
0.8 |
1.6 |
100 |
|
53 |
4 |
1.2 |
1.6 |
100 |
|
Trace |
5 |
1.0 |
1.6 |
80 |
|
0 |
6 |
1.0 |
1.6 |
90 |
|
Trace |
7 |
1.0 |
1.6 |
110 |
|
66 |
8 |
1.0 |
1.6 |
130 |
|
63 |
9 |
1.0 |
0.8 |
110 |
|
0 |
10 |
1.0 |
1.2 |
110 |
|
30 |
11 |
1.0 |
2.0 |
110 |
|
62 |
12
|
1.0
|
1.6
|
110
|
HCl (1.0)
|
74
|
13 |
1.0 |
1.6 |
110 |
CF3SO3H (1.0) |
72 |
14 |
1.0 |
1.6 |
110 |
TsOH·H2O (1.0) |
73 |
15 |
1.0 |
1.6 |
110 |
FeCl3 (1.0) |
0 |
16 |
1.0 |
1.6 |
110 |
Zn(OTf)2 (1.0) |
69 |
17 |
1.0 |
1.6 |
110 |
HCl (0.5) |
66 |
18 |
1.0 |
1.6 |
110 |
HCl (1.5) |
74 |
19 |
1.0 |
1.6 |
110 |
HCl (2.0) |
74 |
20 |
1.0 |
1.6 |
110 |
HCl (3.0) |
56 |
With the optimized conditions in hand, the generality and scope of this I2-promoted Csp3–H oxidation amination/iodination reaction was explored. As summarized in Scheme 3, various acetophenone derivatives smoothly reacted with 2a, to afford the desired products in good yields (3aa–3qa). Substrates with electron-neutral (4-H, 4-Me), electron-donating (4-OMe, 2,4-(OMe)2, 3,4-OCH2O, 3,4-OCH2CH2O), and electron-deficient (4-NO2, 3-NO2) groups, that were attached to the phenyl rings of the aryl methyl ketones, were compatible with this reaction (63–77%, 3aa–3ha). The electronic and steric nature of aromatic methyl ketones was seen to have little influence on the reaction efficiency, and all of the corresponding products were obtained in moderate to good yields. Much to our satisfaction, the optimized conditions were mild enough to allow a broad range of halogenated (4-Br, 4-Cl, 3,4-Cl2) substrates being converted into the corresponding products (70–82%, 3ia–3ka), which could be used as intermediates to synthesize more complex compounds. Meanwhile, sterically hindered 1-acetylnaphthalene 1l and 2-acetylnaphthalene 1m also furnished the desired products 3la and 3ma smoothly in 79% and 72% yields, respectively. Furthermore, the structure of the target compound 3ma was determined by X-ray crystallographic analysis (Fig. S1, ESI†). Heteroaryl methyl ketones were also investigated, and the corresponding products 3na–3qa were obtained in moderate to good yields (57–62%). Unfortunately, alkyl methyl ketones could not undergo the reaction under the standard conditions. Moreover, the scope of substituted pyridin-2-ylmethylamines was also extended. The substrate (3-methylpyridin-2-yl)methylamine could react smoothly with acetophenone and 4-methylacetophenone to give the desired products 3ab and 3bb in 61% and 67% yields, respectively. (5-Bromopyridin-2-yl)methylamine was used to react with acetophenone to afford the product 3ac in moderate yield (50%). Moreover, 1-(pyridin-2-yl)ethylamine was compatible in the reaction and the corresponding product 3ad was obtained in 75% yield. The result indicated that the second iodination should be selective and concurrently implied that it might have occurred in the last step.
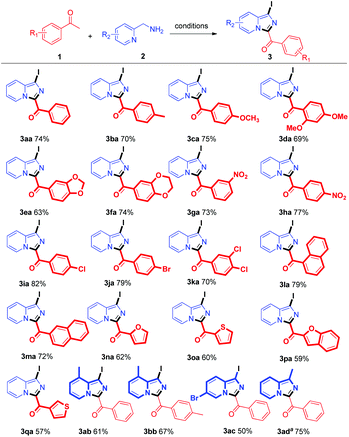 |
| Scheme 3 Scope of aryl methyl ketones and pyridin-2-ylmethylamines. Reaction conditions: 1 (1.0 mmol), 2 (1.0 mmol), I2 (1.6 mmol) and HCl (1.0 mmol) in DMSO (3 mL) at 110 °C for 5 h. Isolated yield. a 1-(Pyridin-2-yl)ethylamine was used instead of pyridin-2-ylmethylamine. | |
To gain some insights into the mechanism of the reaction process, the following experiments were performed. After heating with I2 and HCl in DMSO at 110 °C, acetophenone 1a was transformed into phenylglyoxal (1ab) and the corresponding hydrated species (1ac) in quantitative conversion (Scheme 4a). Subsequently, the reaction of α-iodo ketone 1aa with pyridin-2-ylmethylamine 2a occurred very well to give the product 3aa in good yield (Scheme 4b). When the acetophenone substrate was replaced with hydrated species (1ac), the desired product was obtained in 84% yield (Scheme 4c). These results clearly confirmed that α-iodo ketone 1aa is a possible precursor of α-ketoaldehyde (1ab). Moreover, the results also indicated that phenacyl iodine 1aa and phenylglyoxal 1ac are the key intermediates in the transformation. Finally, the hydrated species (1ac) was treated with pyridin-2-ylmethylamine 2a under the standard conditions without iodine. However, the expected product 3aa′ was not yielded (Scheme 4d). This result emphasized the crucial role of iodine in the subsequent domino process of building the imidazo[1,5-a]pyridin-3-yl(phenyl)methanone skeleton.
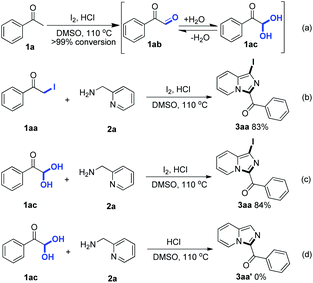 |
| Scheme 4 Control experiments. | |
According to the aforementioned information and based on previous reports,4c,13 a possible mechanism was proposed using acetophenone (1a) and pyridin-2-ylmethylamine (2a) as an example (Scheme 5). Initially, the substrate acetophenone 1a reacted with I2 to afford the intermediate α-iodo acetophenone 1aa, which was converted into phenylglyoxal 1ab with releasing HI after subsequent Kornblum oxidation. The aldehyde 1ab was then attacked by pyridin-2-ylmethylamine (2a) to afford the intermediate C-acyl iminium ion A in the presence of molecular iodine, which would be further attacked by the nitrogen of pyridine followed by oxidation to give the intermediate B. It would then undergo tautomerization to afford intermediate C, which was followed by iodination with I2 to provide the desired product 3aa.
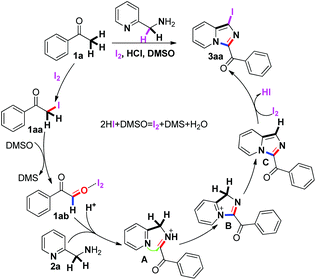 |
| Scheme 5 Proposed mechanism. | |
Conclusions
In summary, a molecular iodine-promoted efficient synthesis of 1-iodoimidazo[1,5-a]pyridines via sequential dual oxidative Csp3–H amination of aryl methyl ketones/Csp3–H iodination of pyridin-2-ylmethylamines has been developed. The initial studies of the mechanism suggest that this reaction could occur through a self-sequenced iodination/Kornblum oxidation/dual Csp3–H amination/oxidation/iodination cascade reaction. Moreover, the present protocol, which utilizes pyridin-2-ylmethylamines as the nitrogen source, and cheap iodine, provides not only a green and attractive approach to 1-iodoimidazo[1,5-a]pyridines, but also a useful example of direct incorporation of iodine atoms from molecular iodine into organic frameworks. Further synthetic applications are now under investigation and the results will be reported in due course.
Acknowledgements
This work was supported by the National Natural Science Foundation of China (Grant No. 21272085 and 21472056). We also thank Dr Chuanqi Zhou, Hebei University, for analytical support. We also acknowledge an excellent doctoral dissertation cultivation grant from Central China Normal University (2015YBYB087).
Notes and references
-
(a)
G. Dyker, Handbook of C–H Transformations Applications in Organic Synthesis, Wiley-VCH, Weinheim, 2005 Search PubMed;
(b) J. Xie, C. D. Pan, A. Abdukadera and C. J. Zhu, Chem. Soc. Rev., 2014, 43, 5245 RSC;
(c) L. M. Xu, B. J. Li, Z. Yang and Z. J. Shi, Chem. Soc. Rev., 2010, 39, 712 RSC;
(d) J. Wencel-Delord and F. Glorius, Nat. Chem., 2013, 5, 369 CrossRef CAS PubMed;
(e) W. Shi, C. Liu and A. W. Lei, Chem. Soc. Rev., 2011, 40, 2761 RSC;
(f) Z. Z. Shi, C. Zhang, C. H. Tang and N. Jiao, Chem. Soc. Rev., 2012, 41, 3381 RSC;
(g) M. L. Louillat and F. W. Patureau, Chem. Soc. Rev., 2014, 43, 901 RSC.
- For representative references, see:
(a) J. L. Jeffrey and R. Sarpong, Chem. Sci., 2013, 4, 4092 RSC;
(b) T. A. Ramirez, B. Zhao and Y. Shi, Chem. Soc. Rev., 2012, 41, 931 RSC;
(c) F. Collet, C. Lescot and P. Dauban, Chem. Soc. Rev., 2011, 40, 1926 RSC;
(d) K. Y. Cho, J. Kwak and B. Chang, Chem. Soc. Rev., 2011, 40, 5068 RSC;
(e) K. Y. Cho, J. Kwak and B. Chang, Chem. Soc. Rev., 2011, 40, 5068 RSC;
(f) Z. K. Chen, B. J. Wang, J. T. Zhang, W. L. Yu, Z. X. Liu and Y. H. Zhang, Org. Chem. Front., 2015, 2, 1107 RSC.
- For recent selected examples, see:
(a) C. M. Che, V. K. Y. Lo, C. Y. Zhou and J. S. Huang, Chem. Soc. Rev., 2011, 40, 1950 RSC;
(b) J. W. Yuan, C. Liu and A. W. Lei, Chem. Commun., 2015, 51, 1394 RSC;
(c) R. W. Evans, J. R. Zbieg, S. L. Zhu, W. Li and D. W. C. MacMillan, J. Am. Chem. Soc., 2013, 135, 16074 CrossRef CAS PubMed;
(d) X. R. Mao, Y. Z. Wu, X. X. Jiang, X. H. Liu, Y. X. Cheng and C. J. Zhu, RSC Adv., 2012, 2, 6733 RSC;
(e) Y. J. Bao, Y. Z. Yan, K. Xu, J. H. Su, Z. G. Zha and Z. Y. Wang, J. Org. Chem., 2015, 80, 4736 CrossRef CAS PubMed;
(f) H. Chen, S. Sanjaya, Y. F. Wang and S. Chiba, Org. Lett., 2013, 15, 212 CrossRef CAS PubMed;
(g) G. T. Rice and M. C. White, J. Am. Chem. Soc., 2009, 131, 11707 CrossRef CAS PubMed;
(h) J. J. Neumann, S. Rakshit, T. Dröge and F. Glorius, Angew. Chem., Int. Ed., 2009, 48, 6892 CrossRef CAS PubMed;
(i) E. T. Nadre and O. Daugulis, J. Am. Chem. Soc., 2012, 134, 7 CrossRef PubMed;
(j) M. Yang, B. Su, Y. Wang, K. Chen, X. Jiang, Y. F. Zhang, X. S. Zhang, G. Chen, Y. Cheng, Z. Cao, Q. Guo, L. Wang and Z. J. Shi, Nat. Commun., 2014, 5, 4707 CrossRef CAS PubMed;
(k) P. H. Dobbelaar and C. H. Marzabadi, Tetrahedron Lett., 2010, 51, 201 CrossRef CAS.
-
(a) Y. Z. Yan, Y. H. Zhang, C. T. Feng, Z. G. Zha and Z. Y. Wang, Angew. Chem., Int. Ed., 2012, 51, 8077 CrossRef CAS PubMed;
(b) Y. Z. Yan, Y. Xu, B. Niu, H. F. Xie and Y. Q. Liu, J. Org. Chem., 2015, 80, 5581 CrossRef CAS PubMed;
(c) Y. P. Zhu, Z. Fei, M. C. Liu, F. C. Jia and A. X. Wu, Org. Lett., 2013, 15, 378 CrossRef CAS PubMed.
- For representative references, see:
(a) P. Finkbeiner and B. J. Nachtsheim, Synthesis, 2013, 979 CAS;
(b) D. Liu and A. W. Lei, Chem. – Asian J., 2015, 10, 806 CrossRef CAS PubMed;
(c) J. S. Tian, K. W. J. Ng, J. R. Wong and T. P. Loh, Angew. Chem., Int. Ed., 2012, 51, 9105 CrossRef CAS PubMed;
(d) X. Wu, Q. H. Gao, S. Liu and A. X. Wu, Org. Lett., 2014, 16, 2888 CrossRef CAS PubMed;
(e) C. D. Zhu, Y. Liang, X. Hong, H. Q. Sun, W. Y. Sun, K. N. Houk and Z. Z. Shi, J. Am. Chem. Soc., 2015, 137, 7564 CrossRef CAS PubMed;
(f) S. Guha, V. Rajeshkumar, S. S. Kotha and G. Sekar, Org. Lett., 2015, 17, 406 CrossRef CAS PubMed;
(g) Q. Jiang, B. Xu, A. Zhao, J. Jia, T. Liu and C. C. Guo, J. Org. Chem., 2014, 79, 8750 CrossRef CAS PubMed;
(h) W. Wei, Y. Shao, H. Y. Hu, F. Zhang, C. Zhang, Y. Xu and X. B. Wan, J. Org. Chem., 2012, 77, 7157 CrossRef CAS PubMed;
(i) B. Q. Zhang, C. F. Wan, Q. Wan, S. Zhang, Z. G. Zha and Z. Y. Wang, Acta Chim. Sin., 2012, 70, 2408 CrossRef CAS.
- For selected examples, see:
(a)
J. A. Joule and K. Mills, Heterocyclic Chemistry, Blackwell Science, Oxford, U.K., 4th edn, 2000, ch. 25 Search PubMed;
(b)
A. R. Katritzky, Comprehensive Heterocyclic Chemistry III, Elsevier, Oxford, U.K., 2008, vol. 11 Search PubMed;
(c)
H. Nakamura and H. Yamamoto, PCT Int. Appl, WO2005043630 Search PubMed;
(d) A. Cappelli, G. Giuliani, M. Anzini, D. Riitano, G. Giorgi and S. Vomero, Bioorg. Med. Chem., 2008, 16, 6850 CrossRef CAS PubMed;
(e) D. Kim, Bioorg. Med. Chem. Lett., 2005, 15, 2129 CrossRef CAS PubMed;
(f) S. H. Kakehi, Y. Okumura, K. Itoh, K. Kobayashi, Y. Aikawa and K. Misawa, Chem. Pharm. Bull., 2010, 58, 1502 CrossRef PubMed.
- For selected examples, see:
(a) J. D. Bower and G. R. J. Ramage, Chem. Soc., 1955, 2823 Search PubMed;
(b) G. Pelletier and A. B. Charette, Org. Lett., 2013, 15, 2290 CrossRef CAS PubMed and references cited therein;
(c) F. Shibahara, A. Kitagawa, E. Yamaguchi and T. Murai, Org. Lett., 2006, 8, 5621 CrossRef CAS PubMed and references cited therein;
(d) F. Shibahara, R. Sugiura, E. Yamaguchi, A. Kitagawa and T. Murai, J. Org. Chem., 2009, 74, 3566 CrossRef CAS PubMed;
(e) Y. Shi, A. V. Gulevich and V. Gevorgyan, Angew. Chem., Int. Ed., 2014, 53, 14191 CrossRef CAS PubMed.
-
(a) Y. Yan, Y. Zhang, Z. Zha and Z. Wang, Org. Lett., 2013, 15, 2274 CrossRef CAS PubMed;
(b) D. C. Mohan, S. N. Rao, C. Ravi and S. Adimurthy, Org. Biomol. Chem., 2015, 13, 5602 RSC;
(c) Z. Q. Xie, J. L. Peng and Q. Zhu, Org. Chem. Front., 2016, 3, 82 RSC.
-
(a) M. Y. Li, Y. Xie, Y. Ye, Y. Zou, H. F. Jiang and W. Zeng, Org. Lett., 2014, 16, 6232 CrossRef CAS PubMed;
(b) H. Q. Wang, W. T. Xu, Z. Q. Wang, L. T. Yu and K. Xu, J. Org. Chem., 2015, 80, 2431 CrossRef CAS PubMed;
(c) Q. Wang, S. Zhang, F. F. Guo, B. Q. Zhang, P. Hu and Z. Y. Wang, J. Org. Chem., 2012, 77, 11161 CrossRef CAS PubMed.
- A. Joshi, D. C. Mohan and S. Adimurthy, Org. Lett., 2016, 18, 464 CrossRef CAS PubMed.
-
(a) F. C. Jia, C. Xu, Q. Cai and A. X. Wu, Chem. Commun., 2014, 50, 9914 RSC;
(b) X. Wu, J. J. Zhang, S. Liu, Q. H. Gao and A. X. Wu, Adv. Synth. Catal., 2016, 358, 218 CrossRef CAS;
(c) J. C. Xiang, M. Wang, Y. Cheng and A. X. Wu, Org. Lett., 2016, 18, 24 CrossRef CAS PubMed.
- For representative reviews on this topic, see:
(a) K. C. Nicolaou, P. G. Bulger and D. Sarlah, Angew. Chem., Int. Ed., 2005, 44, 4442 CrossRef CAS PubMed;
(b) J. Hassan, M. Sevignon, C. Gozzi, E. Schulz and M. Lemaire, Chem. Rev., 2002, 102, 1359 CrossRef CAS PubMed and references therein;
(c) A. F. Littke and G. C. Fu, Angew. Chem., Int. Ed., 2002, 41, 4176 CrossRef CAS;
(d) R. Stürmer, Angew. Chem., Int. Ed., 1999, 38, 3307 CrossRef and references cited therein.
-
(a) G. D. Yin, B. H. Zhou, X. G. Meng, A. X. Wu and Y. J. Pan, Org. Lett., 2006, 8, 2245 CrossRef CAS PubMed;
(b) Q. H. Gao, J. J. Zhang, X. Wu, S. Liu and A. X. Wu, Org. Lett., 2015, 17, 134 CrossRef CAS PubMed;
(c) X. Wu, Q. H. Gao, X. Geng, J. J. Zhang and A. X. Wu, Org. Lett., 2016, 18, 2507 CrossRef CAS PubMed.
Footnote |
† Electronic supplementary information (ESI) available. CCDC 1485477. For ESI and crystallographic data in CIF or other electronic format see DOI: 10.1039/c6qo00313c |
|
This journal is © the Partner Organisations 2016 |