DOI:
10.1039/C5RA23941A
(Paper)
RSC Adv., 2016,
6, 9254-9260
Biobutanol production using unhydrolyzed waste acorn as a novel substrate
Received
12th November 2015
, Accepted 15th January 2016
First published on 18th January 2016
Abstract
Substrate cost and availability are the major bottlenecks that limit the commercialization of next generation biofuels. The possibility of using waste acorn as an abundant and inexpensive feedstock in Iran for acetone–butanol–ethanol (ABE) production with Clostridium acetobutylicum was investigated. No solvent was produced using untreated acorn due to its high contents of growth-inhibitory tannic acid. To circumvent this problem, a simple extraction in close-to-boiling water was employed to remove tannic acid prior to using acorn powder in fermentation. This pretreatment significantly improved the fermentability of acorn powder by C. acetobutylicum. The maximum yields of solvents (g ABE per g substrate) achieved in serum-bottle experiments were 0.43 ± 0.02, 0.40 ± 0.02, and 0.34 ± 0.02 for glucose, pure starch, and treated acorn starch, respectively. Volumetric ABE productivity with tannin-free acorn powder was 0.21 ± 0.01 g L−1 h−1, slightly higher than that achieved with pure starch (0.18 ± 0.05 g L−1 h−1). When scaled up in 5 L fermentors, ABE production using pretreated acorn powder and pure starch resulted in solvent profiles and yields similar to those observed in the serum bottle experiments. This study demonstrates that acorn powder can be a viable feedstock for biobutanol production in Iran. Future work warrants optimization of a two-step process including extraction of tannic acid as a valuable by-product followed by fermentation of tannin-free acorn powder to improve the economic feasibility of acorn to biobutanol conversion process.
1. Introduction
The depletion of fossil fuel resources along with growing concerns over imminent global climate change, greenhouse gas emission, and increasing oil prices have led to increased research on renewable energy resources such as biofuels in the last few decades.1,2 Butanol, a four-carbon alcohol that is widely used as an industrial chemical, is considered to be a potential fuel substitute for combusting engines. Although ethanol is currently the main liquid biofuel produced at an industrial scale, butanol offers several advantages over ethanol. These advantages include lower volatility, more energy content (nearly 30 percent more than ethanol), higher octane number, less corrosive characteristics, and the possibility to blend with gasoline or diesel at any ratio.1,3–5 Besides being an excellent alternative fuel, butanol is an important bulk chemical with numerous industrial applications in food, plastic, and chemical industries.5
Microbial production of butanol using the solvent-producing Clostridium species in the so-called acetone–butanol–ethanol (ABE) fermentation process was one of the first large-scale fermentation processes developed. The ABE process was the second largest industrial fermentation process after ethanol production in the first half of the 20th century. However, the ABE process almost ceased since the early 1960s because of increased substrate price and development of inexpensive petrochemical based butanol.7–10 Recently, butanol production by ABE process has attracted a renewed interest as a result of increasing oil price and environmental problems related to mass consumption of fossil fuels. Therefore, many attempts have been made to improve the economy of ABE process through genetic manipulations of more efficient fermentation strains and development of cost effective product recovery technologies.11 Substrate cost which comprises more than 60% of the production cost8 is one of the major bottlenecks in the fermentative production of butanol.12–14 A variety of low cost substrates such as starchy and lignocellulosic materials have been examined for butanol production.11,15–19
One of the interesting characteristics of Clostridium species is high amylolytic activities which enable them to utilize unhydrolyzed starchy substrates as sole carbon sources.11,20–22 The integration of saccharification and fermentation steps eliminates the need for starch hydrolysis prior to fermentation and reduces equipment and hydrolytic enzyme costs.4,6 Moreover, this alleviates inhibitions associated with high glucose concentration in the culture medium.23
Several starchy materials including corn, cassava, sago, tapioca, potato, and peanut have been investigated for biobutanol production.6,20–23 Most of the examined starchy materials are essential components of the food chain, and therefore they are not reliable resources for sustainable mass production of biofuels. Acorn, the fruit of oak trees, is a non-grain starchy material that is produced in different regions of the world. Quercus brantii, the main species of Iranian oak, grows in more than 4 million hectares in Zagrossian region forests.24 Acorn is usually considered as a waste and useless material. It is mainly comprised of starch24,25 with considerable amounts of tannic acid, a polyphenolic substance with a bitter taste.24,26 Due to its bitter taste and antinutritive effect associated with high tannin content, acorn cannot be directly used as animal feed.24,27 As a result, considerable amounts of acorn fruits are being wasted annually in Iran and many other oak forests around the world (e.g. Mediterrainian forests). However, because of its high starch composition, acorn can be used as a valuable alternative feedstock for fermentation industries, particularly for the production of next generation biofuels.
The main objective of this study was to evaluate the potential of using acorn starch as a cheap and abundant local feedstock for biobutanol production in Iran. Carbon concentration plays an important role in ABE fermentation, especially for the starchy substrates as amylolytic enzymes secretion capacity and activities vary among Clostridium species.20,22,28 We therefore evaluated the effect of different acorn starch concentrations on ABE fermentation profile by Clostridium acetobutylicum in comparison with pure starch and glucose as model substrates. The results showed that C. acetobutylicum was unable to grow and produce ABE using untreated acorn powder as substrate. This was found to be mainly due to the inhibitory effect of tannin content of acorn kernel on the cell growth and ABE production. This study demonstrated that a simple tannin extraction in close-boiling water could produce low tannin content starch fractions from acorn that could be converted to biobutanol with fermentation yields similar to those obtained with pure starch. Besides, tannic acid can be obtained as a valuable by-product. Therefore, it is concluded that acorn fruit, considering its low price and high availability, can be a viable feedstock for biobutanol production in Iran. However, further optimization of tannin extraction and ABE fermentation process parameters are needed to improve the economic feasibility of acorn to butanol conversion operations.
2. Experimental
2.1. Microorganism, medium and inoculum preparation
C. acetobutylicum NRRL B-591 was obtained from Persian Type Culture Collection (PTCC). Standard anaerobic techniques were used in all cultivation and fermentation experiments described here. Sporulated cells were activated by heat shock in cooked meat medium (CMM) at 80 °C for 10 min. The activated spore culture (25 mL) was then transferred to a 60 mL serum bottle and grown anaerobically at 37 °C for 18 h as described previously.23 The activated spore culture was then inoculated (5% v/v) into the peptone–glucose–yeast extract plus P2 (PGY + P2) medium in 118 mL serum bottles with final working volume of 50 mL, and cultivated at 37 °C for 18 h. PGY medium had the following composition (g L−1): glucose, 20; peptone, 3; yeast extract, 1. P2 stock solutions contained the following vitamins (g L−1): para-amino-benzoic acid, 0.1; thiamin, 0.1; biotin, 0.001; buffer (g L−1): KH2PO4, 50; K2HPO4, 50; ammonium acetate, 220; and minerals (gL−1): MgSO4·7H2O, 20; MnSO4·H2O, 1; FeSO4·7H2O, 1; NaCl, 1.23 P2 stock solution was transferred into autoclaved serum bottles via 0.2 μm syringe filter and then purged with filter-sterilized (0.2 μm) O2-free N2 gas for 30 min. A cysteine stock solution (3% w/v) was prepared under completely anaerobic conditions and autoclaved at 120 °C for 15 min.
The carbon and nitrogen sources of the media used for seed culture, serum bottle, and fermentor experiments were prepared anaerobically, autoclaved separately at 120 °C for 15 min, cooled at room temperature, then added together with 1% (v/v) of each of the filter-sterilized P2 stock solutions and 0.5% (v/v) of cysteine solution. The medium pH was adjusted to 6.8 using 4 M NaOH solution. The pH was not controlled during the fermentation in serum bottle and fermentor experiments. All cultures were incubated at 37 °C in a shaking incubator with horizontal agitation at 160 rpm.
2.2. Acorn starch preparation
Mature acorns (Q. brantii) were collected from the oak forests located in the Zagros mountain region, Iran and were shelled out. The acorn kernels were milled using an electric grinder to achieve a size of less than 0.85 mm (20 mesh) and dried at 50 °C for 48 h. This powder was used as carbon source in fermentation experiments.
2.3. Tannic acid extraction
Preliminary experiments showed that the C. acetobutylicum strain could not be cultivated on the acorn powder. That was attributed to the high tannic acid contents of acorn powder and the anti-microbial activity associated with that. Extraction and removal of tannic acid from acorn powder was based on the method described by Pourrat et al.29 and Barthomeuf et al.30 with some modifications. To this end, the acorn powder was placed in a Whatman filter paper (no. 41). The filter paper was soaked in a beaker containing distilled water, then brought to a close-boiling condition (slightly more than 80 °C), and kept for about 5–6 min until dark and bitter water was appeared. The dark wash water was poured off and replaced with fresh water. This process was repeated 5 to 6 times until the wash water was clear. The treated acorn powder was analyzed for tannic acid to ensure that the extraction of tannic acid was performed efficiently. The treated acorn powder was dried at 50 °C for 48 h and used in subsequent fermentations. It should be noted that pH of distilled water was adjusted to 7 during the extraction using 0.5 M NaOH to avoid hydrolysis of acorn starch. The extracted water was analyzed with glucose kit (Man Lab Co., Tehran, Iran) and iodine–potassium iodide reagents31 to examine the hydrolysis or wasting of starch during tannic acid extraction.
2.4. ABE fermentation testing in serum-bottles
ABE fermentation using treated acorn starch as the source of substrate was first tested in 118 mL serum-bottles containing 50 mL of PY (peptone–yeast extract) + P2 medium. The medium contained (g L−1): peptone, 3; yeast extract, 1, and P2 stock solutions as described above. Glucose or pure potato starch (20, 40, and 60 g L−1) were also used as the model carbon sources in parallel experiments. The bottles were inoculated with 2.5 mL of an early stationary phase culture grown in PGY + P2 medium and incubated at 37 °C.
2.5. ABE fermentation scale up in bioreactors
The ABE fermentation using treated acorn starch was scaled up and evaluated in 5 L fermentors (Infors, Switzerland). Bioreactors had a total working volume of 2 litters comprised of PY + P2 medium as described and 20 g L−1 of treated acorn starch or pure starch. Fermentors containing the carbon source were first autoclaved, and autoclaved nitrogen source, and filter-sterilized P2 stock and cysteine solutions were then transferred separately to the fermentors. The bioreactors were inoculated (10% v/v) with an early stationary phase culture grown in PGY + P2 medium. The anaerobiosis in bioreactors before and after inoculation was maintained by purging the medium with filtered O2-free N2 gas until ABE fermentation gases (CO2 and H2) were detected in the fermentors off gas as analyzed by gas chromatography.
2.6. Analytical procedures
Total starch and tannic acid content of acorn kernel powder were determined using a method described by Sluiter and Sluiter32 and Rakić et al.,33 respectively.
For the measurement of acetone, butanol, and ethanol during the fermentation, liquid samples were withdrawn every 12 h and centrifuged at 18
000g, at 4 °C for 20 min. The concentration of ABE in the supernatant was determined by gas chromatography (GC). One μL of the sample was injected into a GC (Agilent 6890, USA) equipped with a FID detector. Analytes were separated on HP-Innowax capillary column (60 m × 0.32 mm I.D., 0.5 μm film thickness) using helium as the carrier gas with flow rate of 1.5 mL min−1. A split ratio of 1
:
50 was used. The injector and detector temperatures were set at 220 and 280 °C, respectively. Initial oven temperature was 80 °C. The oven temperature was increased to 130 °C at a rate of 10 °C min−1 followed by a ramp of 25 °C min−1 to 220 °C. The oven temperature was held at this temperature for 1 min.
ABE yield and ABE productivity were calculated using the following equations:
All experiments were performed at least in duplicate, and the results are presented as mean ± standard deviation.
3. Results and discussion
3.1. Acorn analysis and tannic acid extraction
Total starch and tannic acid contents of the acorn kernel powder were determined as 58.9 ± 2.7% and 12.25 ± 0.3% dry weight, respectively, which are comparable with the previous reports on acorn composition.24,33 No tannic acid was detected in the treated acorn powder, demonstrating that the tannic acid extraction procedure was efficient. Furthermore, the analysis of final tannic acid extraction wash water with glucose kit and iodine–potassium iodide reagent did not show any color change indicating that no hydrolysis or waste of starch has occurred during the extraction. Correia and Beirão-da-Costa34 reported that acorn starch was very stable and exhibited stability similar to cross-linked starch. They also found that granular shape and size of acorn starch did not change under treatments such as low shear at alkaline pH and high shear in water. This high stability of acorn starch could explain why hydrolysis and wasting of starch did not happen during tannic acid extraction at neutral conditions (pH = 7).
3.2. Effect of type and concentration of carbon source on ABE fermentation in serum bottles
Acorn starch was examined as an alternative substrate for ABE fermentation at three different concentrations of 20, 40, and 60 g L−1. Glucose and pure starch were also used separately at the same concentrations as controls. At the end of fermentations, all carbon sources (glucose, pure starch, and treated acorn starch) were completely consumed. Fermentation of glucose was rapid, and maximum ABE yield was achieved after 36 h (Fig. 1). Maximum concentrations of ABE produced from 20, 40, and 60 g L−1 glucose were 8.6, 15.7, and 21.3 g L−1, respectively. These results are consistent with concentrations achieved in previous studies. For example, Jesse et al.23 and Qureshi et al.35 reported 24.7 and 21.1 g L−1 total ABE from 60 g L−1 initial glucose concentration by C. beijerinckii in 125 mL screw capped bottles. In their studies, the authors also observed a reduction in the yield after a maximum due to evaporative loss of the solvents.23
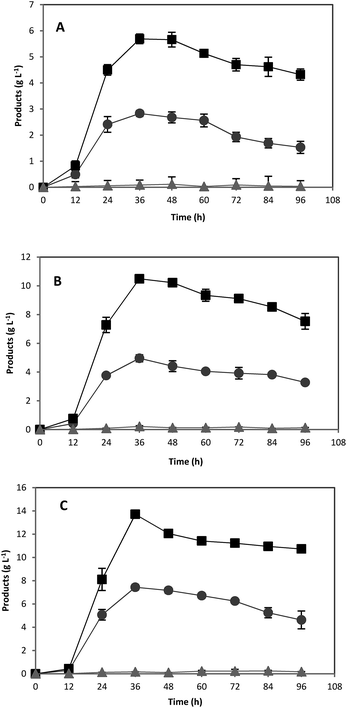 |
| Fig. 1 Acetone (●), butanol (■), and ethanol (▲) fermentation profiles by C. acetobutylicum in 118 mL serum bottles using various glucose concentrations: (A) 20 g L−1; (B) 40 g L−1; (C) 60 g L−1. | |
As expected, using pure starch as a substrate, ABE fermentation was not as rapid as glucose and the maximum ABE concentrations obtained were 8.0, 13.2, and 7.9 g L−1 from 20, 40, and 60 g L−1 initial pure starch, respectively (Fig. 2). While total ABE production was increased by increasing the carbon source concentration from 20 to 40 g L−1 of pure starch, a decrease in ABE concentration was observed when pure starch concentration was further increased from 40 to 60 g L−1. Reduced fermentation performance at high starch concentration may be due to an increase in viscosity, which limits the amylolytic activities and mass transfer rate.20 A similar reduction in ABE productivity due to high viscosity has been observed in other studies upon using starchy materials for ABE production.20,22
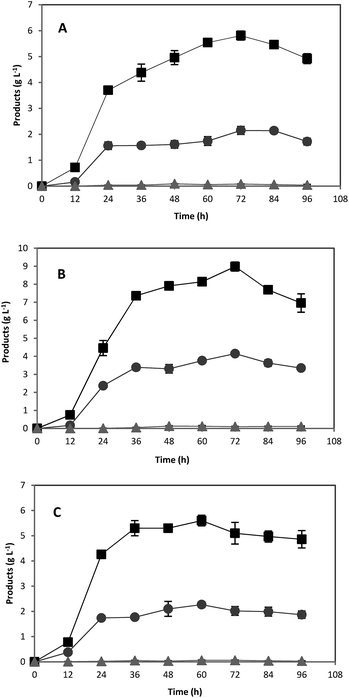 |
| Fig. 2 Acetone (●), butanol (■), and ethanol (▲) fermentation profiles by C. acetobutylicum in 118 mL serum bottles using pure starch at various concentrations: (A) 20 g L−1; (B) 40 g L−1; (C) 60 g L−1. | |
No ABE was detected when untreated acorn powder was used as substrate. Acorn kernel contains considerable amounts of tannins, which exhibit inhibitory effects on the growth of some anaerobic and aerobic bacteria.36 Tannic acid could actively form a complex with metal ions especially iron.37 It has been demonstrated that iron is an essential factor for ABE production by C. acetobutylicum.38 Addition of mineral stock solution to the cultures containing untreated acorn starch or pure starch supplemented with tannic acid caused a dark and purple color, respectively. This could be due to the tannic acid–iron complex formation. Therefore, tannic acid inhibition effect may be due to removing iron or other metal ions from culture access. Toxic effects of tannins have also been reported for animals. Tannins are capable of binding to proteins and form insoluble tannin–protein complexes. They can also inhibit digestive enzymes, and therefore tannin rich plants are not recommended as animal feedstock.39,40 Thus, it is inferred that ABE production is inhibited by tannin content of acorn kernel powder. When treated acorn starch was used as substrate, solvent production was observed. Fermentations were run using 33.9, 67.9, and 101.8 g L−1 of treated acorn powder equivalent to 20, 40, and 60 g L−1 of acorn starch, respectively.
The fermentation time was very similar to that of pure starch, and the maximum ABE concentration achieved were 6.8, 11.6, and 15.5 g L−1 from 20, 40 and 60 g L−1 of treated acorn starch, respectively (Fig. 3).
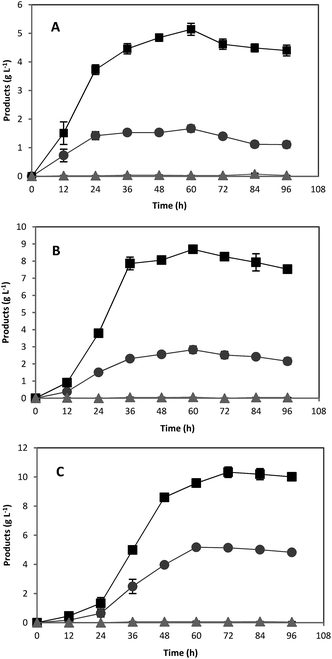 |
| Fig. 3 Acetone (●), butanol (■), and ethanol (▲) fermentation profiles by C. acetobutylicum in 118 mL serum bottles using treated acorn starch at various concentrations of: (A) 20 g L−1; (B) 40 g L−1; (C) 60 g L−1. | |
Unlike pure starch, reduction in solvent production was not observed at 101.8 g L−1 of acorn powder (equivalent to 60 g L−1 of acorn starch). The viscosity of starch solution, which affects the enzymatic and biological reactions, depends on the size of granules and amylose or amylopectin content of starch.20,34,41 Smaller granules size and higher amylose content could result in lower viscosity and better mass transfer rate in gelatinized starch solution. The scanning electron micrographs of acorn and potato starch indicated that acorn starch granules are smaller than potato starch granules.26 The maximum viscosity of gelatinized starch also depends on the amylose content20 and higher contents of amylose results in lower viscosity.34 It has been shown that acorn starch contains more amylose than potato starch (28.4% vs. 21.4%), and potato starch solution is significantly more viscous than the acorn starch solution.26 Therefore, higher amylose content, smaller granule size, and lower viscosity of acorn starch could justify this observation.
Increasing treated acorn starch concentration from 20 to 60 g L−1 was accompanied by increased lag time in ABE production, which was not observed for glucose and pure starch (Fig. 1–3). Although the extraction of tannin compounds using water was repeated several times until the color of extracting water did not change, it is possible that some unknown growth inhibitory chemicals were not extracted with water. Thus, any increase in substrate concentration would increase the concentration of unextractable toxic compounds and enhances their inhibitory effects. This might explain the extended lag phase for the fermentations in which high concentrations of treated acorn starch were used.
A summary of the results for the various fermentation experiments is presented in Table 1. The maximum yields of solvents for the three examined substrates were observed at the substrate concentration of 20 g L−1 (Table 1).
Table 1 Maximum ABE yields and productivities at different concentrations of glucose, pure starch, and treated acorn starch in 118 mL serum bottles
Substrate |
Initial concentration (g L−1) |
ABE yield (g g−1) |
ABE productivity (g L−1 h−1) |
Glucose |
20 |
0.43 ± 0.02 |
0.239 ± 0.009 |
40 |
0.39 ± 0.01 |
0.435 ± 0.011 |
60 |
0.36 ± 0.01 |
0.592 ± 0.013 |
Pure starch |
20 |
0.40 ± 0.02 |
0.112 ± 0.005 |
40 |
0.33 ± 0.01 |
0.184 ± 0.053 |
60 |
0.13 ± 0.01 |
0.132 ± 0.005 |
Treated acorn starch |
20 |
0.34 ± 0.02 |
0.114 ± 0.005 |
40 |
0.29 ± 0.02 |
0.193 ± 0.007 |
60 |
0.26 ± 0.01 |
0.216 ± 0.006 |
In previous studies, the ABE yields of 30% and 34% were reported for hydrolyzed cassava and unhydrolyzed sago starch, respectively,7,42 which are comparable with the ABE yield for treated acorn starch, 34%, obtained in this study. Productivity is also an important factor in ABE fermentation. It has been suggested that a high substrate concentration enhances productivity.28 Although improved productivities were obtained for higher concentrations of glucose, a reduced productivity was observed for the pure starch at 60 g L−1. In contrast to pure starch, high acorn starch concentrations did not result in the reduced ABE productivities.
3.3. Effect of tannic acid on ABE production
The fact that no ABE was detected in the culture media containing untreated acorn powder whereas treatment of acorn powder enabled solvogenesis was attributed to the presence of tannin compounds and their growth inhibitory effects. To make sure that toxicity was due to tannic acid and not to other compounds extracted with water, ABE fermentations were run using pure starch supplemented with various concentrations of pure tannic acid. Concentrations of 0.05 and 0.1 g L−1 of tannic acid led to reduced ABE production but concentrations of above 0.1 g L−1 completely inhibited ABE production (Fig. 4). The lowest acorn powder concentration used in this study (33.9 g L−1 untreated acorn powder) contained a concentration of 4.16 g L−1 tannic acid in the culture medium, and therefore complete ABE inhibition was observed for all fermentations employing untreated acorn powder. Furthermore, the results indicated that tannic acid did not cause any lag time suggesting that lag time was due to the presence of other inhibitory chemicals (Fig. 3 and 4). Inhibitory effect of tannic acid was investigated in another study, and it was found that tannic acid exhibits inhibitory effects to the growth of various bacteria including aerobic and anaerobic such as C. clostridiiforme ATCC 25537, C. perfringens ATCC 13124, Escherichia coli ATCC 25922, Enterobacter cloacae ATCC 13047, and Salmonella typhimurium TA98 within 0.1 to 1 g L−1 concentration range, which could be due to the strong iron binding capacity of tannic acid.35
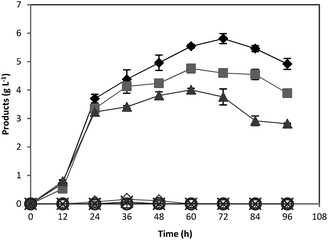 |
| Fig. 4 Effect of various tannic acid concentrations on butanol production. 0 (♦), 0.05 (■), 0.1 (▲), 0.5 (◊), 1 (×), 2 (○), and 3 (□) g L−1 tannic acid. | |
3.4. Fermentation in bioreactor
In order to investigate the scale up effects on ABE fermentation, further studies were performed in 5 L well-controlled fermentors by using optimum concentrations of pure starch and treated acorn powder as substrates. All other conditions were the same as fermentation in serum bottles. Over the course of 72 h, the culture produced 6.45 and 5.81 g L−1 ABE from 20 g L−1 pure starch and treated acorn starch, respectively (Fig. 5). For both pure starch and treated acorn starch, similar ABE production profiles were achieved. However, the produced ABE concentrations were slightly lower than those obtained in 118 mL serum bottles (Fig. 5). The outlet N2 gas was passed through a water-containing vessel in order to separate the solvent carried by the gas. Some acetone was detected in this water suggesting that the lower ABE titer in the bioreactor experiments could be due to evaporation of solvent through the gas stream.
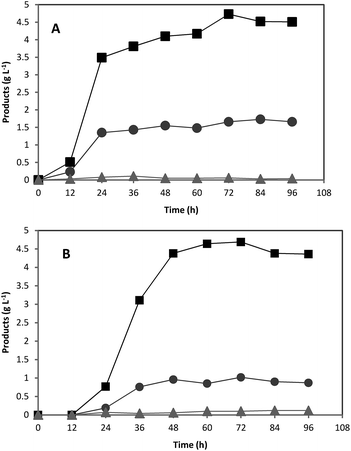 |
| Fig. 5 Acetone (●), butanol (■), and ethanol (▲) fermentation profiles by C. acetobutylicum in a 5 L fermentor containing using (A) 20 g L−1 of pure starch and (B) 20 g L−1 of treated acorn starch as carbon sources. | |
4. Conclusions
Unhydrolyzed acorn starch was utilized as a novel, cheap, and abundant substrate for direct production of ABE using C. acetobutylicum. No solvent was accumulated using untreated acorn powder whereas the extraction of tannin compounds resulted in significant amounts of ABE production. Comparable ABE concentrations achieved using treated acorn starch with those obtained with glucose and pure starch as model substrates at similar concentrations. However, ABE productivities on treated acorn starch were higher than those on pure starch. The results of this study suggest a two-step process for using acorn in the production of high value added products. In the first step, tannic acid is extracted simply by extraction in close-boiling water. Tannin-free acorn powder is then fermented to valuable products such as ABE.
References
- M. Kheyrandish, M. A. Asadollahi, A. Jeihanipour, M. Doostmohammadi, H. Rismani-Yazdi and K. Karimi, Fuel, 2015, 142, 129–133 CrossRef CAS.
- H. Su, Y. Zhao, M. Wang and Y. Xu, RSC Adv., 2015, 5, 12624 RSC.
- H. Amiri, K. Karimi and H. Zilouei, Bioresour. Technol., 2014, 152, 450–456 CrossRef CAS PubMed.
- C. L. Cheng, P. Y. Che, B. Y. Chen, W. J. Lee, C. Y. Lin and J. S. Chang, Appl. Energy, 2012, 100, 3–9 CrossRef CAS.
- N. K. N. Al-Shorgani, M. S. Kalil, W. M. W. Yusoff and A. A. Hamid, Fuel, 2015, 158, 855–863 CrossRef CAS.
- T. C. Ezeji, N. Qureshi and H. P. Blaschek, J. Biotechnol., 2005, 115, 179–187 CrossRef CAS PubMed.
- T. C. Ezeji, N. Qureshi and H. Blaschek, Appl. Microbiol. Biotechnol., 2004, 63, 653–658 CrossRef CAS PubMed.
- M. H. Huesemann, L. J. Kuo, L. Urquhart, G. A. Gill and G. Roesijadi, Bioresour. Technol., 2012, 108, 305–309 CrossRef CAS PubMed.
- G. Jurgens, S. Survase, O. Berezina, E. Sklavounos, J. Linnekoski, A. Kurkijärvi, M. Väkevä, A. van Heiningen and T. Granström, Biotechnol. Lett., 2012, 34, 1415–1434 CrossRef CAS PubMed.
- N. Qureshi, X. L. Li, S. Hughes, B. C. Saha and M. A. Cotta, Biotechnol. Prog., 2006, 22, 673–680 CrossRef CAS PubMed.
- Y. S. Jang, A. Malaviya, C. Cho, J. Lee and S. Y. Lee, Bioresour. Technol., 2012, 123, 653–663 CrossRef CAS PubMed.
- J. R. Gapes, J. Mol. Microbiol. Biotechnol., 2000, 2, 27–32 CAS.
- K. Kraemer, A. Harwardt, R. Bronneberg and W. Marquardt, Comput. Chem. Eng., 2011, 35, 949–963 CrossRef CAS.
- Z. Sun and S. Liu, Biomass Bioenergy, 2012, 39, 39–47 CrossRef CAS.
- F. Moradi, H. Amiri, S. Soleimanian-Zad, M. R. Ehsani and K. Karimi, Fuel, 2013, 112, 8–13 CrossRef CAS.
- C. Sasaki, Y. Kushiki, C. Asada and Y. Nakamura, Ind. Crops Prod., 2014, 62, 286–292 CrossRef CAS.
- A. Ranjan, S. Khanna and V. S. Moholkar, Appl. Energy, 2013, 103, 32–38 CrossRef CAS.
- A. Ranjan, S. Khanna and V. S. Moholkar, Biomass Convers. Biorefin., 2012, 3, 143–155 CrossRef.
- A. Ranjan, S. Khanna and V. S. Moholkar, 3 Biotech, 2013, 3, 353–364 CrossRef.
- M. S. Madihah, A. B. Ariff, K. M. Sahaid, A. A. Suraini and M. I. A. Karim, World J. Microbiol. Biotechnol., 2001, 17, 567–576 CrossRef CAS.
- Z. Chang, D. Cai, C. Wang, L. Li, J. Han, P. Qin and Z. Wang, RSC Adv., 2014, 4, 21819 RSC.
- H. T. M. Tran, B. Cheirsilp, B. Hodgson and K. Umsakul, Biochem. Eng. J., 2010, 48, 260–267 CrossRef CAS.
- T. W. Jesse, T. C. Ezeji, N. Qureshi and H. P. Blaschek, J. Ind. Microbiol. Biotechnol., 2002, 29, 117–123 CrossRef CAS PubMed.
- A. Saffarzadeh, L. Vincze and J. Csapo, Acta agraria Kaposváriensis, 1999, 3, 59–69 Search PubMed.
- Z. Lu, F. He, Y. Shi, M. Lu and L. Yu, Bioresour. Technol., 2010, 101, 3642–3648 CrossRef CAS PubMed.
- H. Chung, S. Cho, J. Chung, T. Shin, H. Son and S. T. Lim, Food Sci. Biotechnol., 1998, 7, 269–275 Search PubMed.
- M. G. Cappai, P. Wolf, V. G. Liesner, A. Kastner, G. Nieddu, W. Pinna and J. Kamphues, Livest. Prod. Sci., 2010, 134, 183–186 CrossRef.
- D. T. Jones and D. R. Woods, Microbiol. Rev., 1986, 50, 484–524 CAS.
- H. Pourrat, F. Regerat, P. Morvan and A. Porrat, Biotechnol. Lett., 1987, 9, 731–734 CrossRef CAS.
- C. Barthomeuf, F. Regerat and H. Pourrat, J. Ferment. Bioeng., 1994, 77, 320–323 CrossRef CAS.
- R. M. McCready, J. Guggolz, V. Silviera and H. S. Owens, Anal. Chem., 1950, 22, 1156–1158 CrossRef CAS.
- A. Sluiter and J. Sluiter, Technical ReportNREL/TP-510-42624, 2008 Search PubMed.
- S. Rakić, S. Petrović, J. Kukić, M. Jadranin, V. Tešević, D. Povrenović and S. Šiler-Marinković, Food Chem., 2007, 104, 830–834 CrossRef.
- P. R. Correia and M. L. Beirão-da-Costa, Starch-Stärke, 2010, 62, 421–428 CrossRef CAS.
- N. Qureshi, B. C. Saha, B. Dien, R. E. Hector and M. A. Cotta, Biomass Bioenergy, 2010, 34, 559–565 CrossRef CAS.
- K. T. Chung, Z. Lu and M. W. Chou, Food Chem. Toxicol., 1998, 36, 1053–1060 CrossRef CAS PubMed.
- P. Schofield, D. M. Mbugua and A. N. Pell, Anim. Feed Sci. Technol., 2001, 91, 21–40 CrossRef CAS.
- F. Monot, J. R. Martin, H. Petitdemange and R. Gay, Appl. Environ. Microbiol., 1982, 44, 1318–1324 CAS.
- R. Kumar and M. Singh, J. Agric. Food Chem., 1984, 32, 447–453 CrossRef CAS.
- H. Mehansho, L. G. Butler and D. M. Carson, Annu. Rev. Nutr., 1987, 7, 423–440 CrossRef CAS PubMed.
- C. Brandam, X. M. Meyer, J. Proth, P. Strehaiano and H. Pingaud, Biochem. Eng. J., 2003, 13, 43–52 CrossRef CAS.
- C. Virunanon, C. Ouephanit, V. Burapatana and W. Chulalaksananukul, J. Cleaner Prod., 2013, 39, 273–279 CrossRef CAS.
Footnote |
† Present address: Novozymes North America Inc. 77 Perrys Chapel Church Rd. Franklinton, NC 27617, USA. |
|
This journal is © The Royal Society of Chemistry 2016 |
Click here to see how this site uses Cookies. View our privacy policy here.