DOI:
10.1039/C5RA24358K
(Review Article)
RSC Adv., 2016,
6, 14778-14791
The removal of heavy metal ions from wastewater/aqueous solution using polypyrrole-based adsorbents: a review
Received
18th November 2015
, Accepted 19th January 2016
First published on 21st January 2016
Abstract
Water pollution caused by heavy metal ions is becoming a serious threat to human and aquatic lives day by day. Therefore, the treatment of heavy metal ions is of special concern for environmental scientists and engineers. Historically, various methods, such as physical and chemical precipitation, ion-exchange, reverse osmosis, membrane filtration, electrochemical treatment, solvent extraction, and adsorption, have been widely studied for the removal of these metal ions from aqueous/wastewater. However, over the past few decades, conducting polymer-based adsorbents have received considerable attention owing to their potential applications for different heavy metal ions especially Cr(VI), Zn(II), and Pb(II). Among the various conducting polymers, polypyrrole (PPy) based adsorbents play a major role for the removal of various heavy metal ions due to their ease of synthesis, biocompatibility and redox properties. The current review has mainly focused on the physico-chemical properties, adsorption characteristics and mechanism of different polypyrrole-based adsorbents, including PPy/biosorbents, PPy/Fe3O4 nanocomposites, PPy–polyaniline nanofibers, PPy–graphene nanocomposites, exfoliated PPy-organically modified clay nanocomposites, and hierarchical porous PPy-nanoclusters, as well as their applications towards the removal of heavy metal ions.
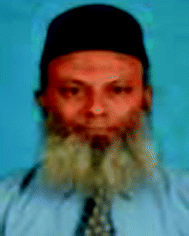 Habibun Nabi Muhammad Ekramul Mahmud | H. N. M. Ekramul Mahmud, Ph.D. has received his BSc and MSc in Applied Chemistry and Chemical Technology from the University of Dhaka, Bangladesh in 1986 and 1987, respectively. He received his M.Eng in Chemical Engineering in 1998 from the Universiti Teknologi Malaysia (UTM) and his Ph.D. from Universiti Putra Malaysia (UPM) in 2006. His research interests include synthesis and characterization of conducting polymers and their various applications, as well as catalytic conversion of biomass to energy. He is now working as an associate professor in the Department of Chemistry, University of Malaya (UM), Malaysia. |
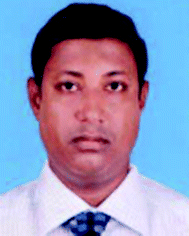 A. K. Obidul Huq | A. K. Obidul Huq obtained his BSc (Hons.) and MS in Nutrition and Food Science from the University of Dhaka, Bangladesh in 2001 and 2002, respectively. He is working at the Department of Food Technology and Nutritional Science, Mawlana Bhashani Science and Technology University, Bangladesh and currently pursuing his Ph.D. in conducting polymer at the Department of Chemistry, University of Malaya, Malaysia. His research interests include the development of eco-friendly bio-adsorbents for the treatment of wastewater effluent and the management of food industries' by-products. |
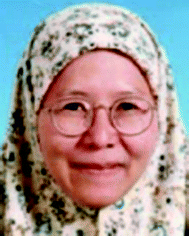 Rosiyah binti Yahya | Rosiyah binti Yahya, Ph.D. has received her BSc in Chemistry from Leicester University, United Kingdom and Ph.D. from Brunel University, United Kingdom in 1990. Her research interests include materials chemistry in particular polymers, composite materials and nanomaterials, as well as their various applications. She is now working as a professor in the Department of Chemistry, University of Malaya (UM), Malaysia. |
1. Introduction
Nowadays, hazardous heavy metal pollution of wastewater is the utmost significant environmental problem and endangers human beings throughout the world. Due to rapid urbanization and industrialization, such as metal plating, mining, tanneries, painting, batteries, paper industries, printing and photographic industries, pesticides and fertilizer industries, and car radiator manufacturing, heavy metal ions, such as As(III)/AS(V), Pb(II), Cd(II), Ni(I), Cr(III)/Cr(VI), Zn(II), Cu(II), Hg(I)/Hg(II), and Co(II) contained in wastewater are increasingly directly or indirectly discharged into streams, lakes, rivers or oceans, especially in developing countries.1–9 Moreover, the soils surrounding military bases offer a potential risk of certain heavy metals, which can be a threat to groundwater and surface water.9,10 Characteristically, these heavy metal ions are not biodegradable and tend to easily accumulate in living organisms.10–13 Although taking in a trace amounts of different heavy metals is extremely necessary for human beings due to their presence of some vitamins or co-factors, excessive exposure or intake can have dangerous consequences. In addition, a lot of physical and mental retardation, such as nausea, vomiting, diarrhea, asthma, pneumonia, skin degeneration, kidney and liver malfunction, congenital abnormalities, weight loss and various cancers, can be the result of heavy metals found in industrial wastewater.8–16 The toxicity and the consequences of these heavy metals are highlighted here using the findings of the World Health Organization (WHO) and the United States Environmental Protection Agency (US EPA).
1.1. Arsenic (As)
Although arsenic (As) is a naturally occurring metalloid and common constituent of the earth's crust, its ground and surface water contamination is very critical.13–16 It has led to a massive epidemic of arsenic poisoning such as skin or lung cancer and even bladder cancer in Asia and America, and especially in India, Bangladesh, Vietnam, Cambodia, Thailand, Chile, Southwest USA and Canada.13,14,17 Therefore, it is categorized as the first priority toxic element by the WHO and US EPA.18,19 The inorganic species, arsenate [As(V)] and arsenite [As(III)] are the predominant forms of As in groundwater and surface water.20 The main problem of As is that even at low concentrations, chronic exposure may cause As poisoning.13–17,20–22 Thus, the WHO and various environmental protection agencies set the permissible limits of As at 0.01 mg L−1 in drinking water.18,19
1.2. Lead (Pb)
Inorganic lead (Pb) arises from a number of industrial fuel, leaded gasoline and mining sources, much of which eventually enters natural water systems.23,24 Acute Pb poisoning in humans causes severe dysfunction in the kidneys, liver and reproductive system.23–28 Pb poisoning from environmental exposure is also known to cause mental retardation, especially in children.27,28 Its toxic symptoms are anaemia, insomnia, headache, dizziness, irritability, weakness of muscles, hallucination and renal damage.23–28 Therefore, Pb has been classified as a priority pollutant by the US EPA. The maximum contaminant level (MCL) of Pb ions in drinking water has been set at a very low level of 0.015 mg L−1, whereas the WHO limits it at 0.05 mg L−1.18,19
1.3. Chromium (Cr)
Chromium (Cr) is extensively used in electroplating, leather tanning, metal finishing, nuclear power plant, dying, photography industries and textile industries.29–33 In an aqueous solution, it exists in both the Cr(III) and Cr(VI) forms. Hexavalent chromium, which is more toxic, alters human physiology, accumulates in the food chain and causes severe health problems ranging from simple skin irritation to lung cancer.31–38 The permissible limit of Cr(VI) for industrial effluents to be discharged into surface water is 0.1 mg L−1 and for drinking water is 0.05 mg L−1.18,37
1.4. Mercury (Hg)
Mercury (Hg) is released into the environment through the discharge from agricultural fungicide, chemicals, waste incineration, electronic materials, scientific instruments (thermometers, barometers), batteries, dental amalgams, textile, photographic and pharmaceutical industries, and fossil fuel combustion.39–42 It is a neurotoxin that can cause damage to the central nervous system.43 High concentrations of Hg causes the impairment of pulmonary and kidney function, chest pain and dyspnea.39–49 The classic example of mercury poisoning is Minamata Bay.44,45 In consideration of its risk, the US EPA has listed mercury as a priority pollutant and has mandated an upper limit of 2 ppb for Hg(II) in drinking water.19
1.5. Cadmium (Cd)
Cadmium (Cd) is spread in some surface and subsurface waters via welding, electroplating, Cd and Ni batteries, nuclear fission plants, paints and plastics, and fertilizers.50–52 It is well known that chronic cadmium toxicity is the cause of Japan Itai–Itai disease.51 Acute or chronic exposure of Cd also causes high blood pressure, kidney damage, the destruction of testicular tissue, osteoporosis and the destruction of red blood cells.50–58 Cd may replace zinc in some enzymes, thereby altering the stereostructure of the enzyme and impairing its catalytic activity.57 It has been classified by US EPA as a probable human carcinogen and the safe drinking water limit set up to 0.005 mg L−1.18,19
1.6. Zinc (Zn)
Trace amounts of some metal ions, such as zinc (Zn), copper (Cu) and cobalt (Co), are required by organisms as cofactors for enzymatic processes.59,60 However, an excess of these metal ions will cause serious problems in living organisms due to their higher toxicity, carcinogenic and bioaccumulation.59–62
Zn is one of the most common pollutants for surface and groundwater as it has versatile uses.62 Again, due to its non-biodegradability and acute toxicity, Zn-containing liquid and solid wastes are considered as hazardous wastes. An excessive amount of Zn exposure can cause well-known health problems such as stomach cramps, skin irritations, vomiting, nausea and anemia.59–62 The WHO recommends the maximum acceptable concentration of Zn ions in drinking water at 5.0 mg L−1.18
1.7. Copper (Cu)
Like Zn, copper (Cu) is an essential element for living organisms, including humans and is necessary in small amounts in our diet to ensure good health. However, the excessive ingestion of Cu brings about serious toxicological concerns, such as vomiting, diarrhea, stomach cramps and nausea, or even death.56,63–66 The WHO recommends the maximum acceptable concentration of Cu ions in drinking water at 1.5 mg L−1, whereas the US EPA defines it at 1.3 mg L−1.18,19
1.8. Cobalt (Co)
Cobalt (Co) is one of the most important transition metals, which plays double-dealing in both harmful and beneficial impact on human beings. The increased use of Co(II) in nuclear power plants and in many industries, such as petrochemical, metallurgical, electroplating, battery, dye, mining and electronic industries, generates large quantities of effluent and thus contaminates surface and groundwater.9,61,67–68 Although, a minute amount of Co is needed for the formation of vitamin B12, excessive exposure can be hazardous.9 A lot of physical and mental problems, such as vomiting, nausea, diarrhea, asthma, pneumonia, kidney congestion, skin degeneration and weight loss, can occur due to excess Co in wastewater.67–70 The permissible limits of cobalt allowed to be in irrigation water, inland surface water and drinking water are 1, 0.05 and 0.01 mg L−1, respectively.18
1.9. Nickel (Ni)
The major sources of nickel contamination in water come from industrial process such as electroplating, batteries manufacturing, mining, metal finishing and forging.71–73 Ni ions are non-biodegradable toxic heavy metals and may cause dermatitis and allergic sensitization, lung and kidney problems and are a known human carcinogen.71–74 According to the WHO guidelines, the maximum permissible concentration of Ni in industrial discharge wastewater is 2 mg L−1, while that in drinking water it should be less than 0.1 mg L−1.18
The maximum contaminant level or permissible limits of some heavy metal ions in drinking water have been set at slightly different values by the two most authentic international organizations, the WHO and US EPA, as can be observe in Table 1.
Table 1 The permissible limits of some heavy metal ions in drinking water
Heavy metal ions |
Permissible limits (ppm) |
WHO18 |
US EPA19 |
As(III)/As(V) |
0.05 |
0.01 |
Pb(II) |
0.05 |
0.015 |
Cd(II) |
0.005 |
0.005 |
Cr(VI)/Cr(III) |
0.05 |
0.05 |
Hg(II) |
0.001 |
0.002 |
Zn(II) |
5.0 |
5.0 |
Cu(II) |
1.5 |
1.3 |
Co(II) |
0.01 |
— |
Ni(I) |
0.1 |
— |
1.10. Suitable techniques for the removal of heavy metal ions
Heavy metal ions existing in various aqueous streams/wastewater from multiple sources are currently one of the most important environmental concerns. Therefore, it is necessary to remove heavy metal ions from contaminated wastewater prior to its discharge to the environment to protect the aquatic lives and human beings.
Although several methods have been used for many years to remove heavy metal ions from wastewater, achieving the most effective treatment is still challenging for environmental scientists/engineers. Physical and chemical precipitation, ion-exchange, reverse osmosis, membrane filtration, electrochemical treatment, solvent extraction and adsorption processes are the widely used methods for removing heavy metal ions from aqueous streams/wastewater.4,9,15,65,75–82 To date, various adsorption methods have been considered as effective and widely used methods due to their simplicity and easy operational conditions.80–86 In addition, adsorption is mostly reversible, thus the adsorbents can be easily regenerated using a suitable desorption process.83–85
Several inorganic and organic adsorbents have been utilized for the adsorption method, including zeolites, montmorillonite, clay minerals, trivalent and tetravalent metal phosphates, biosorbents, activated carbon, polymer-based adsorbent, and polymer-inorganic hybrid adsorbents.15,33,47,83–95 Among the polymer-based adsorbents, conducting polymer-based adsorbents, such as polyaniline (PANI), polyethylenamine (PEI), polypyrrole (PPy) and their composites, have received considerable attention due to their potential applications in adsorbing various heavy metal ions, ease of synthesis, regeneration and operation, environmental and mechanical stability, and low cost. In addition, their highly porous structures with specific surface electro-chemical properties as well as ion exchange capacities have also drawn much attraction from researchers.89–96 Moreover, the existence of positively charged nitrogen atoms in polypyrrole provides a good prospect for their applications in adsorption.97
This article presents an overview of the various polypyrrole-based polymeric adsorbents used for the removal of heavy metal ions from aqueous streams/wastewater sources. These polymeric adsorbents can be produced from different chemical oxidation polymerization processes from pyrrole to obtain simple polypyrroles and multi-dimensional PPy formation such as PPy-bio-adsorbents, PPy-magnetic composites, PPy-nanofibers, PPy–graphene nanocomposites and hierarchical porous PPy-nanomaterials. The effects of different parameters, such as the pH of the solution, adsorbent dosage, initial concentration of heavy metal ions, and contact time, on the removal efficiency and adsorption capacity of the adsorbents are also discussed in this section.
2. Polypyrrole-based adsorbents
2.1. Polypyrrole conducting polymer
Polypyrrole (PPy) conducting polymer has been extensively researched due to its varied potential applications, environmental stability, high conductivity, redox properties and ease of synthesis.89,90,93–97 PPy synthesized in solutions with small dopants, such as Cl−, ClO4−, and NO3−, mainly exhibits anion exchanging behavior due to the high mobility of these ions in the polymer matrix. However, under certain conditions, cationic exchange was also found to occur with large dopants, such as polyvinylsulfonate and polystyrenesulfonate, due to immobility of these ions in the polymer matrix.98 PPy has also exhibited good prospects in adsorption applications because of the nitrogen atoms present in the polymer chains. Therefore, the removal of heavy metal ions is one of the applications of conducting polymers. A lot of effort has been made to remove heavy metal ions using polypyrrole conducting polymers prepared via the chemical oxidative polymerization of pyrrole in the presence of different dopants under different conditions, as the adsorption efficiency largely depends on the conditions used to prepare PPy.89,99–105
One of the studies used FeCl3·6H2O as an oxidant in an aqueous solution to form PPy using a molar ratio of monomer to oxidant of 1
:
1.97 The effects of various parameters, such as the pH of the solution, dosage of the adsorbent and contact time, have been investigated and this study exhibited a 100% adsorption efficiency for the removal of Ni ions from aqueous solution at pH 7.0, 8 h of contact time and 0.08 g PPy adsorbent dose with a 1 ppm initial Ni ion concentration. The FT-IR spectrum (Fig. 1) showed that the coordination of Ni ions to the nitrogen atoms in the PPy polymer chain were involved in the adsorption process and a possible mechanism of metal ion adsorption is shown in Scheme 1.97
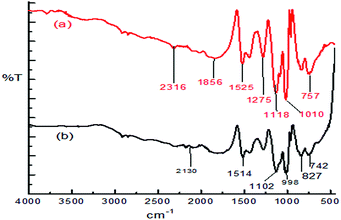 |
| Fig. 1 The FTIR spectra of the PPy before (a) and after (b) the adsorption of nickel.97 | |
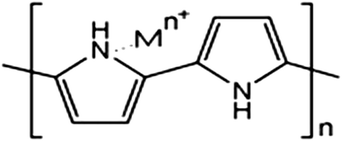 |
| Scheme 1 A plausible mechanism for metal ion adsorption on the surface of nitrogen functional groups in PPy conducting polymer.97 | |
Another study revealed that PPy has been prepared via the chemical oxidative polymerization of pyrrole using anhydrous FeCl3 as an oxidant100 and various parameters such as the pH of the solution, dosage of the adsorbent and contact time were investigated. This study has shown the prepared PPy markedly removes (84%) Ni ions under alkaline pH conditions and the results compared with other adsorbents (Table 2).
Table 2 Heavy metal adsorption capacity on polypyrrole-based materials
Type of adsorbents |
Heavy metal ions |
Optimum conditions/experimental conditions |
Adsorption |
(mg g−1) |
% |
Conducting PPy97 |
Ni |
pH 7.0; contact time 8 h; dose 0.08 g; initial conc. 1 ppm |
— |
100 |
Conducting PPy100 |
Ni |
pH 12.0; contact time 30 min; dose 0.5 g; initial conc. 100 mg L−1 |
— |
84 |
Conducting PPy106 |
As(III) |
Contact time 30 min; dose 0.25 g; initial conc. 5 mg L−1 |
— |
7.4 |
PPy particles with acetonitrile107 |
Cd(II) |
pH 6.6; temp. 45 °C; batch adsorption equilibrium time 12 min, initial conc. varied 10–350 mg L−1 |
71.4 |
— |
PPy particles with acetonitrile107 |
Co(II) |
pH 6.6; temp. 45 °C; batch adsorption equilibrium time 12 min, initial conc. varied 10–350 mg L−1 |
70.04 |
— |
PPy–PVP108 |
Cd(II) |
pH 5; contact time 45 min; room temp.; dose 0.25 g; initial conc. 62.36 mg L−1 |
— |
51.41 |
PPy–PVA108 |
Cd(II) |
pH 5; contact time 45 min; room temp.; dose 0.25 g; initial conc. 62.36 mg L−1 |
— |
49.98 |
PPy–SD62 |
Zn(II) |
pH 3.0; contact time 14 min; temp. 40 °C; dose 0.5 g; initial conc. 100 mg L−1 aqueous solution |
— |
94.4 |
PPy–SD109 |
Cr(VI) |
pH 5.0; equilibrium time 15 min; temp. 25 °C; dose 0.5 g; initial conc. 100 mg L−1 |
3.4 |
— |
PPy/rice husk ash56 |
Cu(II) |
Contact time 25 min; room temp.; dose 0.5 g; 700 rpm; textile wastewater containing initial conc. 0.94 mg L−1 |
— |
96.4 |
PPy/rice husk ash56 |
Cd(II) |
Contact time 25 min; room temp.; dose 0.5 g; 700 rpm; textile wastewater containing initial conc. 0.28 mg L−1 |
— |
92.4 |
Polypyrrole functionalized chitin110 |
Cr(VI) |
pH 4.8; temp. 30–50 °C; batch adsorption contact time 60 min; dose 0.1 g; initial conc. 50 mg L−1; 250 rpm |
28.92–35.22 |
— |
PPy-g-chitin58 |
Pb(II) |
pH 6.0; contact time 60 min; temp. 50 °C; dose 0.1 g; initial conc. 10 mg L−1 aqueous solution |
9.14 |
— |
PPy-g-chitin58 |
Cd(II) |
pH 6.0; contact time 60 min; temp. 50 °C; dose 0.1 g; initial conc. 10 mg L−1 aqueous solution |
6.49 |
— |
PPy-glycine doped composites38 |
Cr(VI) |
pH 2.0–5.0; temp. 25 °C; batch adsorption contact time 0.5–3 h |
217 |
— |
Fe3O4@glycine-doped PPy magnetic nanocomposites111 |
Cr(VI) |
pH 2.0; temp. 25 °C; batch adsorption contact time 0.5–3 h; dose 0.1 g; initial conc. 200 mg L−1 |
238 |
99.91 |
PPy/Fe3O4 nanocomposites112 |
Cr(VI) |
pH 2.0; temp. 25 °C; fixed bed column flow rate 3 mL min−1 with 100 mg L−1 |
230.17 |
— |
PPy/Fe3O4 magnetic nanocomposites113 |
Cr(VI) |
pH 2.0; temp. 25 °C; batch adsorption contact time 12 h |
169.4 |
— |
Bamboo-like PPy nanotubes114 |
Cr(VI) |
pH 2.0; room temp. |
482.6 |
— |
MWCNT–PPy nanotubes115 |
Pb(II) |
pH 6.0; room temp. continuous column; flow rate 1.5 mL min−1 |
25.0 |
— |
PPy–PANI nanofibers33 |
Cr(VI) |
pH 2.0; equilibrium time 30–180 min; temp. 25 °C |
227 |
— |
PANI–PPy copolymer9 |
Co(II) |
pH 7.0; equilibrium time 11 min; dose 0.11 g with initial conc. 100 mg L−1 |
— |
99.68 |
PAN/PPy core/shell nanofiber mat116 |
Cr(VI) |
pH 2.0; equilibrium time 30–90 min; temp. 25 °C |
62 |
— |
Orange-like Fe3O4/PPy composites microspheres117 |
Cr(VI) |
pH 2.0; temp. 25 °C; batch adsorption contact time 30–180 min |
209.2 |
— |
PPy/γ-Fe2O3 (ref. 118) |
Cr(VI) |
pH 2.0; equilibrium time 15 min; temp. 25 °C |
209 |
— |
PPy/γ-Fe2O3 (ref. 118) |
Cu(II) |
pH 5.5; equilibrium time 35 min; temp. 25 °C |
171 |
— |
PPy–GO nanosheets119 |
Cr(VI) |
pH 3.0; temp. 25 °C; batch adsorption contact time 24 h |
497.1 |
— |
PPy–GO NC120 |
Cr(VI) |
pH 2; temp. 25 °C; batch adsorption equilibrium time 50 min, dose 0.025 g, 200 rpm |
625 |
— |
GO–αCD–PPy nanocomposites121 |
Cr(VI) |
pH 2; temp. 25–45 °C; batch adsorption equilibrium time 30–200 min |
606–666 |
— |
PPy–rGO47 |
Hg(II) |
pH 3.0; temp. 20 °C; batch adsorption contact time 3 h |
980 |
— |
PPy–Fe3O4/rGO122 |
Cr(VI) |
pH 3; temp. 30–45 °C; batch adsorption equilibrium time 720 min |
293.3 |
— |
PPy-OMMTNC123 |
Cr(II) |
pH 2.0; temp. 20 °C; batch adsorption dose 0.15 g; contact time 24 h |
119.34 |
— |
Graphene/Fe3O4@PPy nanocomposites124 |
Cr(VI) |
pH 2.0; temp. 25 °C; batch adsorption, magnetic separation |
348.4 |
— |
PPy coated Fe3O4 nanocomposites125 |
Cr(VI) |
pH 2.0; room temp.; continuous flow rate 0.2 L min−1 with 20 mg L−1; residence time 30 min |
— |
80–98 |
PPy–TP nanocomposite126 |
Cr(VI) |
pH 2; temp. 25–45 °C; batch adsorption equilibrium time 19 min, dose 0.2 g; initial conc. 200 mg L−1 aqueous solution |
31.64 |
— |
PPy/silica nanocomposites127 |
Hg(II) |
Stirred room temp 10 h |
0.97 mmol g−1 |
PPy/silica nanocomposites127 |
Pb(II) |
Stirred room temp 10 h |
0.53 mmol g−1 |
PPy/SH-beta/MCM-41 (ref. 49) |
Hg(II) |
pH 8.0; temp. 25–45 °C; batch adsorption contact time 10 min; dose 0.11 g; initial conc. 400 mg L−1 |
157.43 |
— |
PPy/SBA-15 nanocomposite128 |
Hg(II) |
pH 8.0; temp. 25–45 °C; batch adsorption contact time of 60 min dose 0.1 g; initial conc. 60 mg L−1 |
200 |
— |
PANI/PPy/HMS129 |
Cd(II) |
pH 8.0; room temp.; batch adsorption contact time of 8 min dose 0.04 g; initial conc. 50 mg L−1 |
384.61 |
99.2 |
Hierarchical porous PPy-nanoclusters2 |
Cr(II) |
pH 5.0; temp. 20 °C; batch adsorption equilibrium time 20 min |
3.47 mmol g−1 |
A recent study on the removal of Cd and Co ions from aqueous solutions was carried out using the oxidative polymerization of pyrrole in acetonitrile and this study showed the maximum monolayer adsorption capacity of Cd and Co ions to be 71.4 and 70.04 mg g−1, respectively (Table 2).107 Their findings suggest that Cd and Co could be used as suitable agents for doping polypyrrole conducting polymers. Another recent study also showed the successful synthesis of polypyrrole using ferric chloride as an oxidant in the presence of poly(vinyl pyrrolidone) (PPy/PVP) and poly(vinyl alcohol) (PPy/PVA) as surfactants in aqueous media.108 The role of the various surfactants on controlling the particle size and homogeneity of the polymer for its use as adsorbents for various heavy metals from aqueous solution has been reported. The removal percentage of Cd(II) was carried out using a batch method at pH 5 with a contact time of 45 minutes as the optimum conditions of sorption. The prepared PPy/PVP and PPy/PVA showed an adsorption efficiency of 51.41% and 50.64%, respectively.
2.2. Polypyrrole bio-adsorbents
The adsorption of different heavy metal ions using various PPy-based bio adsorbents is a very promising process in terms of low cost, renewable sources and ecofriendly. The major benefits of these types of bio-adsorbents are their wide range of effectiveness. Different forms of inexpensive materials, such as sawdust, rice husks, and chitin, have been studied as potential bio-adsorbents for heavy metals.
2.2.1. Polypyrrole sawdust (PPy/SD) composites. Sawdust (SD) obtained from wood industry is an abundant by-product, low cost and easily available. It contains various organic compounds with polyphenolic groups103 that could be easily coated with PPy and form PPy/SD composites, which can bind heavy metal ions through different conditions and mechanisms.130 Several experiments on the efficiency of PPy/SD for the removal of Zn(II), Ni and Cr(VI) ions have been conducted by researchers.62,109,131–133Omraei et al. showed the PPy/SD composite has considerable potential for the removal of Zn(II) from aqueous solution using a batch method.62 The optimum conditions for sorption were been found to be a PPy/SD dose of 0.5 g in 100 mL, a contact time of 14 min, pH 3.0 and temperature 40 °C, and under these conditions the maximum removal efficiency of PPy/SD was 94.4%. Again, this study also demonstrated its effective adsorption in wastewater containing 328, 32.5 and 15.2 mg L−1 of Cr(VI), Ni and Zn(II), respectively. The removal efficiency was found to be 96.4%, 93.5% and 92.8% for Cr(VI), Ni and Zn(II), respectively.62 Ansari and Fahim also prepared PPy/SD wherein FeCl3 was used as the chemical oxidant for the oxidation of pyrrole into the polymer (PPy/Cl).109 This study demonstrated that PPy/SD can be used in both batch and column adsorption as an efficient sorbent used for the removal of Cr(VI) ion from aqueous solutions. In the batch system, the removal of 98% Cr(VI) was found under the optimum conditions for sorption (PPy/SD dose 1.0 g in 25 mL; 100 ppm initial Cr(VI) concentration, contact time 15 min, pH 5.0 and temperature 25 °C). Again, in the column system, a maximum 98.6% removal efficiency for Cr(VI) was found using a uniformly packed 1.0 g PPy/SD in glass column at 2 mL min−1 with the other factors remaining constant.
2.2.2. Polypyrrole–rice husk ash (PPy/RHA) composites. Rice husk ash (RHA) is a solid obtained after burning rice husk, which is another natural abundant by-product of rice milling, low cost and easily available. A polypyrrole/rice husk ash nanocomposite was prepared by coating the rice husk ash substrate with pyrrole using chemical oxidative polymerization.56 Their ability in the removal of heavy metals from wastewater were investigated by several researchers.56,134 Ghorbani and Eisazadeh studied a continuous mode fixed bed column with a flow rate of 1.0 mL min−1 using different bed depths such as 10, 20 and 30 cm and found that PPy/RHA can be used as an effective adsorbent in wastewater treatment.56 Another study reported the percentage efficiency of Cu and Cd ions removal was 96.4% and 92.4%, respectively, using 0.5 g adsorbent dosage with only 25 minutes of contact time at room temperature.134
2.2.3. Polypyrrole with chitin (PPy/ch) composites. Polypyrrole functionalized chitin (PPy-Ch) was synthesized via in situ polymerization and batch experiments were carried out to examine the adsorption of Cr(VI) ions under certain conditions.110 This study showed a maximum adsorption capacity of 35.22 mg g−1 for an initial concentration of 50 mg L−1 at 50 °C and pH 4.8 with 60 min of contact time and 0.1 g adsorption dosage. The use of chitin alone as an adsorbent for heavy metals appears to be unsatisfactory due to its poor solubility in common solvents, low sorption capacity and poor stability. Therefore, it has been introduced to the polypyrrole structure to enhance the sorption capacity of this conducting polymer-based bio-adsorbent. Another study was carried out to investigate the possibility of using chemically modified chitin with polypyrrole (PPy-g-Ch) as an adsorbent for the removal of Pb(II) and Cd(II) ions from an aqueous solution.58 The maximum removal was obtained at pH 6 for both Pb(II) and Cd(II) ions with an adsorption capacity of 9.14 and 6.49 mg g−1, respectively. Ion-exchange and electrostatic attraction followed by complexation have been found as the plausible mechanism for the removal of Pb(II) and Cd(II) ions from aqueous solution.
2.3. Glycine-doped polypyrrole and its magnetic nanocomposites
Chromium is the top most heavy metal ion contaminant found in highly industrial areas in developing countries due to its versatile uses.33–38,111 Therefore, environmental scientists are more concerned to remove Cr ions efficiently and a lot of polymeric adsorption techniques have been applied.33,38,111–114,116 In this continuation, a highly efficient removal of Cr(VI) using glycine doped polypyrrole from an aqueous solution has been investigated, which was prepared via the in situ polymerization of pyrrole monomer in the presence of glycine.38 Formation of the PPy homopolymer and the inclusion of glycine in the PPy matrix were confirmed using ATR-FTIR spectroscopy and XRD analysis, respectively (Fig. 2 and 3).38
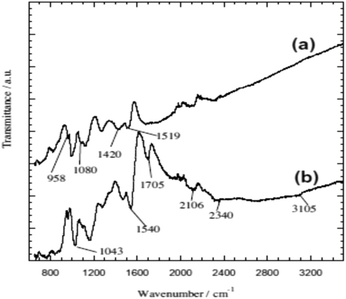 |
| Fig. 2 The ATR-FTIR spectra of (a) PPy homopolymer and (b) PPy-gly.38 | |
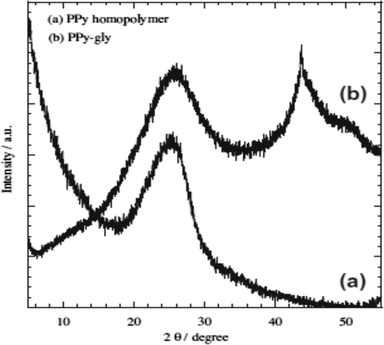 |
| Fig. 3 XRD curves of (a) PPy homopolymer and (b) PPy-gly.38 | |
The adsorption of Cr(VI) onto the PPy-gly adsorbent was highly pH dependent and the removal efficiency of PPy-gly was much higher compared to other PPy homopolymers (Table 2). The maximum adsorption capacity of PPy-gly was found to be 217 mg g−1 at pH 2.0 and 25 °C. It is much better than the other reported polymer-based materials. It is much better than the other reported polymer-based materials. The adsorption mechanism was shown to be the ionic interactions between the amine groups of gly and the HCrO4− ions (Scheme 2).38 In another attempt, a very similar study was conducted by Ballav et al. for the removal Cr ions.111 In this study, a Fe3O4 coated glycine doped polypyrrole magnetic nanocomposite (Fe3O4@gly-PPy NC) was prepared. An adsorption capacity of 238 mg g−1 was reported with a maximum removal efficiency of 99.91%. The adsorption is highly pH dependent and the adsorbent can be separated using an external magnetic field.
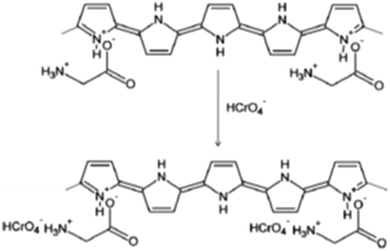 |
| Scheme 2 The plausible adsorption mechanism for the removal of Cr(II) ions using the PPy-gly adsorbent.38 | |
2.4. Polypyrrole/Fe3O4 nanocomposites
Various forms, size and shapes of PPy/Fe3O4 nanocomposites have recently been used for the adsorption of heavy metal ions from wastewater or an aqueous solution.111–113,117,118,135–137 A Fe3O4 coated PPy magnetic nanocomposite has been prepared via the in situ polymerization of pyrrole monomer for the removal of highly toxic Cr(VI).113 The batch experimental results showed upto 100% adsorption with a 200 mg L−1 Cr(VI) aqueous solution at pH 2. The adsorption results showed that the Cr(VI) removal efficiency using the nanocomposite decreased with an increase in pH. XPS studies also suggested that ion exchange and reduction on the surface of the nanocomposite may be the possible mechanism for Cr(VI) removal by the PPy/Fe3O4 nanocomposite (Fig. 4).113 Two energy bands at about 577.5 eV and 587.2 eV corresponding to the binding energies of the Cr (2p3/2) and Cr (2p1/2) orbitals were observed.113 This observation suggests the existence of both Cr(III) and Cr(VI) on the adsorbent surface. The existing of Cr(VI) species on the surface of the adsorbent are consistent with the sorption of Cr(VI) ions due to the anion exchange properties of PPy by replacing the doped Cl− ions, as shown in Scheme 3. The presence of Cr(III) on the nanocomposite surface suggests that some fraction of the adsorbed Cr(VI) was reduced to Cr(III) via a reduction process. The reduction process may be due to the presence of electron rich polypyrrole moieties in the nanocomposite.
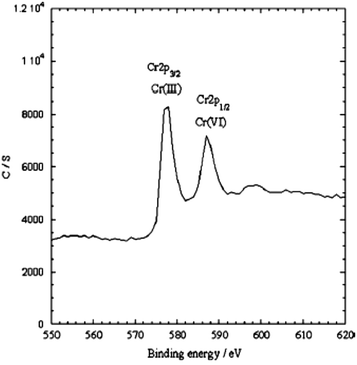 |
| Fig. 4 The XPS spectra of the PPy/Fe3O4 nanocomposites after Cr(VI) adsorption.113 | |
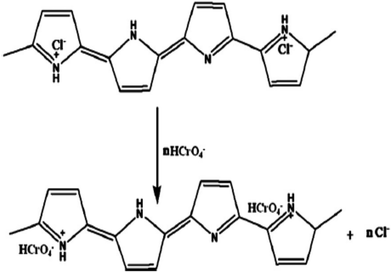 |
| Scheme 3 A plausible mechanism for the removal of Cr(II) ions from aqueous solution.113 | |
Another study showed that a similar PPy/Fe3O4 nanocomposite has been synthesized via an in situ chemical oxidative polymerization technique with 9 h of preparation time.112 A fixed-bed column with continuous flow was also selected at a flow rate of 3 mL min−1 with 100 mg L−1 of Cr(VI). From this experiment, it has been confirmed that the breakthrough curve was dependent on the bed mass, initial Cr(VI) concentration, pH and flow rate. The efficiency of the PPy/Fe3O4 nanocomposite in environmental water to remove Cr(VI) ions was effective to give below acceptable levels of Cr(VI) upon processing 5.04 L of water with an initial 76.59 mg L−1 Cr(VI) concentration using only 2 g of adsorbent. An alternative method using a magnetic adsorption separation (MAS) process for extracting Cr(VI) ions from aqueous solution with polypyrrole coated Fe3O4 nanocomposites has been reported very recently.125 The sorption capacity is influenced by the Fe3O4 nanoparticles loading. The separation process was aided by a mechanical and magnetic filtration mechanism by introducing steel wool into the separation chamber. This special arrangement yielded a 100% absorption capacity for Cr(VI) ions from an aqueous solution.
2.5. Polypyrrole-nanofibers
The inherent features of PPy, which include ease of chemical and electrochemical polymerization, a capacity to form an adhesive coating with different substrates, ease of chemical substitution to modify its properties and porous structure enables the exchange of ions with the surrounding medium, allow its application to heavy metal ions removal from an aqueous solution/wastewater.33,118,135–138 The use of other conducting polymers, such as polyaniline (PANI) and polyacrylonitrile (PAN), have been used with PPy for heavy metal ions removal from an aqueous solution due to the presence of imine and amine groups, which can chelate metal ions and also adsorb anionic metal species through electrostatic interactions or hydrogen bonding.
Nowadays, nanotubes, nanowires, nanobelts or nanofibers structure based materials have been extensively used in medical, filtration, barrier, drug delivery, energy storage and many other sophisticated purposes due to their high aspect ratio and uniqueness.9,11,33,118 The existence of nitrogen atoms in polyaniline and polypyrrole provides a good prospect for their applications in adsorption separation.138 Therefore, a few studies have been adapted using both PPy and PANI as a nanofiber in adsorption technology9,33 and PAN with PPy.137 One of the pioneering studies showed that PPy–PANI nanofibers as an adsorbent of Cr(VI) were prepared without a template via the coupling of propagating PPy˙+ and PANI˙+ free radicals via the simultaneous polymerization of pyrrole and aniline monomers in the presence of FeCl3 as an oxidant. The inclusion of both polymeric moieties, PPy and PANI, in the fibers was confirmed by ATR-FTIR spectroscopy (Fig. 5) and the polymeric process is shown in Scheme 4.
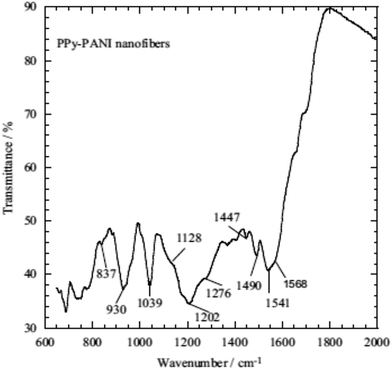 |
| Fig. 5 The ATR-FTIR spectrum of the PPy–PANI nanofibers.33 | |
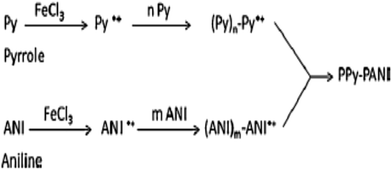 |
| Scheme 4 The polymerization of pyrrole and aniline monomers, and the formation of PPy–PANI nanofibers.33 | |
The experiments were carried out in a batch system to investigate the effect of pH, adsorbent dose, contact time, temperature and concentration of Cr(VI) ions. The adsorption of Cr(VI) ions on the nanofibers surface has been shown highly pH dependent and the maximum adsorption capacity of the PPy–PANI nanofibers for Cr(VI) was found to be 227 mg g−1 (Table 2).33 Again, selective adsorption of Cr(VI) from an aqueous solution was achieved in the presence of other co-existing ions. The main inspiration for synthesizing the nanofibers lies in fact that the incorporation of PANI into the growing polymer chain of PPy provides an increase in surface area that may enhance Cr(VI) ion adsorption when compared to its polypyrrole homopolymer counterpart and also overcome the limitations of the rareness of the new conjugated bond-containing monomers.139 Another recent study showed that PANI/PPy copolymer nanofibers can be synthesized via an in situ chemical polymerization method and demonstrated its capacity for the removal of Co(II) from aqueous solutions.9 Under the optimum conditions of the batch method, the sorption of Co(II) ions showed 99.68% removal efficiency for a 100 mg L−1 Co(II) solution (Table 2).
Another study reported on bamboo-like PPy nanotubes that were successfully prepared via reactive-template vapor phase polymerization for Cr(VI) ion removal.114 The adsorption capacity of the bamboo-like PPy nanotubes (482.6 mg g−1) was much higher than that of traditional PPy nanoparticles (Table 2).
A recent study showed a multi-walled carbon nanotubes–polypyrrole conducting polymer nanocomposite (MWCNT–PPy nanotube composite) could be successfully synthesized and applied to the removal of Pb(II) ions.115 The adsorption capacity of the nanocomposite was reported to be 25.0 mg g−1 at pH 6.0 and room temperature. Because of the uniform structure of the MWCNT and its higher affinity for heavy metals, the material has been incorporated into PPy to realize the enhanced adsorption capacity of the prepared nanotube composites.
2.6. Polypyrrole–graphene nanocomposites
Due to optimal transformation of life, the demand for higher performance applications of nanoadsorbent materials has dramatically increased in the last few years. Different nanoparticles, such as zeolites, Fe2O3, TiO2, carbon nanotubes (CNT), graphene oxide nanosheets or nanoplatelets, have been employed to characterize the nanocomposites at different stages of polymerization.140–143 Graphene is a two-dimensional monoatomic thin sheet with a large lateral dimensional sp2-hybridized carbon nanostructure and has unique properties of high tensile strength and Young's modulus, good electrical and thermal conductivities, and high aspect ratio.142–144
Theoretical and experimental results show that single-layered graphene sheets are the strongest materials developed to date,142 and as a result, it has made possible novel applications, for example, the removal of heavy metal ions by nanoadsorbents. Recently, some study has been carried out on the preparation of PPy/graphene oxide (PPy/GO) and PPy/graphene nanocomposites for their applications in supercapacitor, transparent electrodes and environmental fields.142–149 There are some reports on hierarchical PPy/GO nanosheets that combine the 2D nanosheets of GO and 3D nanoflowers of PPy.144–147 Very recently, a study has been attempted to fabricate PPy/GO nanocomposite sheets using a simple and reliable sacrificial template polymerization method to remove Cr(VI) from an aqueous solution.119 The adsorption capacity of the PPy/GO composite nanosheets is about two times as large as that of conventional PPy nanoparticles. Again, in another study, a polypyrrole graphene oxide nanocomposite (PPy–GO NC) was synthesized via an in situ polymerization of Py monomer in GO dispersion and used for Cr(VI) ion adsorption from aqueous solutions using both batch and packed-bed column methods.120 The results showed that in the batch mode at 25 °C and pH 2, the maximum sorption capacity was 625 mg g−1.
A novel graphene oxide–alpha cyclodextrin–polypyrrole nanocomposite (GO–αCD–PPy NC) has been successfully synthesized and applied for toxic hexavalent chromium removal from an aqueous solution.121 Having both hydrophilic and hydrophobic characteristics, alpha cyclodextrin appeared to be useful in adsorbing organic and inorganic pollutants from wastewater forming inclusion complexes. To take advantage of this material as an adsorbent, it has been used to form a nanocomposite with graphene oxide and polypyrrole for a synergetic adsorption effect for hexavalent chromium ions removal. The maximum adsorption capacity was found to be 666.67 mg g−1 under optimum conditions (Table 2). Another novel hybrid nanocomposite prepared via anchoring the Fe3O4@polypyrrole nanospheres with hierarchical porous structure on graphene nanosheets (graphene/Fe3O4@PPy, GFP) has been reported for the removal of Cr(VI) ions.124 The GFP exhibits an excellent adsorption capability (348.4 mg g−1) for Cr(VI) removal due to the combined effect of graphene and Fe3O4@polypyrrole.
Again, a short communication reported a facile chemical route to synthesize a polypyrrole–reduced graphene oxide composite that showed a highly selective Hg2+ ion removal capacity.47 Usually, materials functionalized with sulphur, nitrogen or oxygen have shown a high binding affinity to mercury. Conducting polymers incorporating these functional groups, interfaced with carbon and carbon-based derivatives have shown the enhanced removal of mercury and other toxic materials from water, but their surface area is not large,150 which has been shown to be their major drawback. Thus, the higher surface area of reduced graphene oxide (rGO) and higher stability as well as non-toxicity of PPy motivated the synthesis of a PPy–rGO composite used for the removal of mercury(II) ions. The synthesis was achieved via the chemical exfoliation of graphite to graphene oxide and its subsequent reduction to rGO in the presence of PPy. The high BET surface area of the rGO (280.7 m2 g−1) influences the morphology of the PPy and increases the surface area from 6.18 m2 g−1 (PPy) to 166 m2 g−1 (PPy–rGO). The large surface area of the PPy–rGO composite increases the binding sites for mercury ions in solution. On the other hand, this prepared adsorbent is more stable and environmentally friendly. The adsorption capacity of PPy–rGO was much higher (980 mg g−1) when compared to other traditional adsorbents (Table 2) with a characteristic feature of higher desorption capacity and good practical applications in the treatment of wastewater.151
Fig. 6 shows another facile synthesis of polypyrrole decorated rGO–Fe3O4 magnetic composites (PPy–Fe3O4/rGO) in two steps, which is used for the removal of Cr(VI) ions.122 The results showed that the ternary PPy–Fe3O4/rGO nano-hybrid exhibited excellent performance for chromium(VI) removal from an aqueous solution. The maximum adsorption capacity for Cr(VI) on PPy–Fe3O4/rGO was 293.3 mg g−1. The removal process was found to be pH dependent, exothermic and spontaneous. Both the electrostatic attraction and ion-exchange properties of the prepared nanocomposites were involved in the adsorption process of Cr(VI) ions.
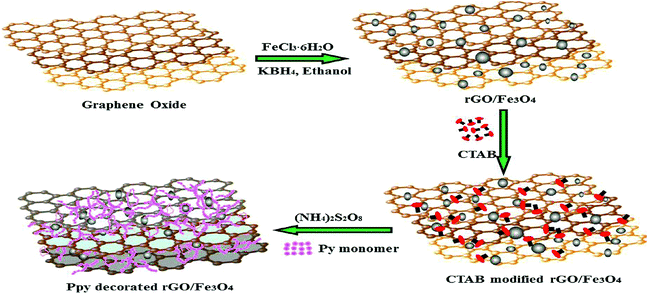 |
| Fig. 6 Schematic of the preparation of the ternary composites.122 | |
2.7. Polypyrrole and other nanocomposites
Not only graphene, many other synthetic nano-adsorbents or organically modified clay nanocomposites or hierarchical porous polypyrrole nanoclusters act as a potential adsorbents for heavy metal ions especially for the more abundant Cr(VI) ions.2,49,126–128 As a synthetic adsorbent, a polypyrrole–titanium(IV) phosphate nanocomposite (PPy–TiP) was prepared via an in situ oxidative polymerization process.126 The adsorption process was based on thermodynamic parameters and was spontaneous and endothermic. The maximum adsorption capacity for Cr(VI) ion removal was found to be 31.64 mg g−1 under the optimum conditions (Table 2).
For the last few years, various fabrications of nanocomposite materials using conducting polymers and inorganic materials have been introduced in the nanotechnology area. For example, Maeda and Armes reported colloidal nanocomposites as adsorbents that were formed when pyrrole or aniline was oxidatively polymerized in the presence of silica (SiO2) or tin(IV) oxide.152 Recently, a short communication described a simple fabrication method for PPy/silica nanocomposites with cratered surfaces using a modified silica-templated oxidation/polymerization of pyrrole in the presence of FeCl3 as the oxidant.127 The PPy/silica nanocomposites were examined with regard to removing heavy metals from water. The results showed a relatively high adsorption capacity for Hg2+ and Pb2+ compared to Cd2+ and Cr3+, which was most likely due to a complexation reaction between the secondary amine of pyrrole and the heavy metal ions (Table 2). However, another study showed the enhanced adsorption of Cd2+ ions using a nanostructured composite of modified hexagonal type mesoporous silica with polyaniline/polypyrrole nanoparticles (PANI/PPy/HMS).129 The adsorption efficiency was found to be 99.91% after only 8 min (Table 2). Mesoporous M41S silicate groups, including MCM-41 and SBA-15, are considered as suitable adsorbents due to their high surface area, high pore volume and ordered structure to functionalize its surface.153–156 A composite of polypyrrole/thiol-functionalized beta/MCM-41 (PPy/SH-beta/MCM-41) was prepared via in situ polymerization of pyrrole in the presence of SH-beta/MCM-41.49 This study revealed that the adsorption of Hg2+ onto PPy/SH-beta/MCM-41 is an endothermic and spontaneous process, and is potentially able to remove Hg2+ ions from aqueous solutions at even high concentrations (400 mg L−1) with an adsorption capacity of 157.43 mg g−1. Another composite of PPy/SBA-15 was synthesized via chemical oxidation with FeCl3 for 5 h.128 A maximum adsorption capacity of Hg(II) removal (200 mg g−1) was observed under the optimal conditions of pH 8, contact time of 60 min and absorbent dosage of 1 g L−1 at room temperature (Table 2).
An exfoliated PPy-organically modified montmorillonite clay nanocomposite (PPy-OMMTNC) was prepared as a potential adsorbent via the in situ polymerization of pyrrole monomer (Fig. 7) and used for the adsorption of toxic Cr(VI) from an aqueous solution.123 In batch adsorption studies, it has been found that the removal efficiency was dependent on the pH, contact time, temperature and initial concentration, and the optimum removal of Cr(VI) ions was achieved at pH 2 wherein the maximum adsorption capacity was found to be 119.34 mg g−1 at 25 °C (Table 2).
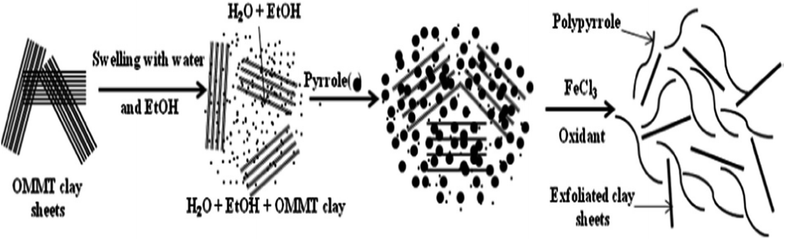 |
| Fig. 7 The formation of exfoliated PPy-OMMTC nanocomposites.123 | |
It has been already reported that for the adsorption of Cr(VI) onto PPy based materials, an ion-exchange mechanism was involved via replacement of the doped Cl− by the HCrO4− ions.113 However, some of Cr(VI) ions were reduced to Cr(III) by the electron-rich PPy polymer after adsorption. The whole adsorption and reduction of Cr(VI) onto the exfoliated PPy-OMMTNC has been shown to occur via another mechanism (Scheme 5).
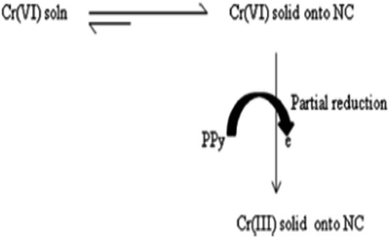 |
| Scheme 5 The mechanism for adsorption and partial reduction of Cr(VI) onto the PPy-OMMTC nanocomposite.123 | |
Hierarchical porous nanomaterials have drawn more attention due to their higher surface area compared to traditional nanoparticles, nanocapsules or nanofibers.2,157 A recent study was based on hierarchical porous polypyrrole nanoclusters, which were synthesized via a reactive-template method wherein Fe3O4 nanoclusters acted as both the template for shaping the PPy nanostructures and the oxidant.2
The prepared PPy-nanoclusters exhibited a wide surface area as high as 104 m2 g−1 and due to their special hierarchical porous structures it was 3.5 times larger than that of traditional PPy. The maximum removal amount of Cr(VI) ions for the PPy-nanoclusters was 3.47 mmol g−1 in an aqueous solution at pH 5.0 (Table 2).
3. Conclusions and prospects
This review article offers the extensive information on the removal of different heavy metal ions from aqueous streams/wastewater effluents using a wide range of polypyrrole-based adsorbents. The effects of various parameters associated with the adsorption of heavy metal ions from aqueous solutions were compiled and discussed. They have some potential advantages and limitations. Polypyrrole homopolymer and PPy-bio-adsorbents have been readily synthesized and used for the removal of different heavy metal ions due to their low cost and simplicity. PPy-bio-adsorbents are recognized as effective and economic adsorbents for low concentration heavy metal ions treatment. However, the adsorption capacity is low and largely depends on the type of adsorbent. Among the available polymeric adsorbents, PPy-based nanomaterials have been widely explored as highly efficient adsorbents used for removal of different heavy metal ions from different aqueous/wastewater sources due to the presence of highly active surface sites in the nano-adsorbents. They exhibit various advantages, such as fast kinetics, high capacity and preferable sorption, towards heavy metal ions in aqueous streams/wastewater. Nevertheless, to further promote the practical applications of PPy-based polymers in the abatement of heavy metal pollution, there still exist some technical constraints to be solved. To date, from a commercial point of view, no greater success has been achieved using conducting polymer-based adsorbents, particularly, polypyrrole and its composites. Extensive research is imperative to materialise the commercial success of conducting polymer-based adsorbents, which will provide a new dimension in adsorption technology towards mitigating the environmental pollution problem. Various polypyrrole-based composites/nanocomposites/bio-composites should be sourced out and focus on regeneration to minimize the cost involved for their commercial applications.
4. Conflict of interest
The authors declare no conflict of financial interest or any others.
Acknowledgements
The authors would like to acknowledge the University Malaya Research Grant (UMRG Project no. RG-262/13AFR and RP014A-15SUS) for financial support.
Notes and references
- J. O. Nriagu and J. M. Pacyna, Nature, 1988, 333, 134 CrossRef CAS PubMed
. - T. Yao, T. Cui, J. Wu, Q. Chen, S. Lu and K. Sun, Polym. Chem., 2011, 2, 2893 RSC
. - K. Kadirvelu, K. Thamaraiselvi and C. Namasivayam, Bioresour. Technol., 2001, 76, 63 CrossRef CAS PubMed
. - T. Aman, A. A. Kazi, M. U. Sabri and Q. Bano, Colloids Surf., B, 2008, 63, 116 CrossRef CAS PubMed
. - Y. Jiang, H. Pang and B. Liao, J. Hazard. Mater., 2009, 164, 1 CrossRef CAS PubMed
. - S. Babel and T. A. Kurniawan, Chemosphere, 2004, 54, 951 CrossRef CAS PubMed
. - M. R. Awual, S. Urata, A. Jyo, M. Tamada and A. Katakai, Water Res., 2008, 42, 689 CrossRef CAS PubMed
. - B. Samiey, C. Cheng and J. Wu, Materials, 2014, 7, 673 CrossRef CAS
. - H. Javadian, J. Ind. Eng. Chem., 2014, 20, 4233 CrossRef CAS
. - R. W. Peters, J. Hazard. Mater., 1999, 66, 151 CrossRef CAS PubMed
. - S. E. Bailey, T. J. Olin, R. M. Bricka and D. D. Adrian, Water Res., 1999, 33, 2469 CrossRef CAS
. - A. Netzer and D. Hughes, Water Res., 1984, 18, 927 CrossRef CAS
. - T. Joseph, B. Dubey and E. A. McBean, Sci. Total Environ., 2015, 527–528, 540 CrossRef CAS PubMed
. - R. Singh, S. Singh, P. Parihar and V. P. Singh, Ecotoxicol. Environ. Saf., 2015, 112, 247 CrossRef CAS PubMed
. - D. Mohan and C. U. Pittman, J. Hazard. Mater., 2007, 142, 1 CrossRef CAS PubMed
. - P. Z. Ray and H. J. Shipley, RSC Adv., 2015, 5, 29885 RSC
. - S. Vadahanambi, S. Lee, W. Kim and K. Oh, Environ. Sci. Technol., 2013, 47, 10510 CAS
. - Organization WH, Guidelines for drinking-water quality: recommendations, World Health Organization, 2004 Search PubMed
. - C. Griffiths, H. Klemick, M. Massey, C. Moore, S. Newbold and D. Simpson, et al., US Environmental Protection Agency valuation of surface water quality improvements, Rev. Environ. Econ. Pol., 2012, 6, 1 CrossRef
. - R. S. Oremland and J. F. Stolz, Science, 2003, 300, 939 CrossRef CAS PubMed
. - S. Kapaj, H. Peterson, K. Liber and P. Bhattacharya, J. Environ. Sci. Health, Part A: Environ. Sci. Eng., 2006, 41, 2399 CrossRef CAS PubMed
. - M. R. Awual, M. A. Hossain, M. A. Shenashen, T. Yaita, S. Suzuki and A. Jyo, Environ. Sci. Pollut. Res., 2013, 20, 421 CrossRef CAS PubMed
. - S. Bhowmick, S. Chakraborty, P. Mondal, W. V. Renterghem, S. V. Berghe and G. Roman-Ross, et al., Chem. Eng. J., 2014, 243, 14 CrossRef CAS
. - E. M. Jouad, F. Jourjon, G. Le Guillanton and D. Elothmani, Desalination, 2005, 180, 271 CrossRef CAS
. - M. R. Awual and M. M. Hasan, Microporous Mesoporous Mater., 2014, 196, 261 CrossRef CAS
. - R. Naseem and S. Tahir, Water Res., 2001, 35, 3982 CrossRef CAS PubMed
. - R. Nevin, Environ. Res., 2009, 109, 301 CrossRef CAS PubMed
. - G. Ding and Y. Bao, Sci. Total Environ., 2014, 472, 289 CrossRef CAS PubMed
. - S. A. Katz and H. Salem, J. Appl. Toxicol., 1993, 13, 217 CrossRef CAS PubMed
. - V. K. Gupta, A. Rastogi and A. Nayak, J. Colloid Interface Sci., 2010, 342, 135 CrossRef CAS PubMed
. - R. M. Sedman, J. Beaumont, T. A. McDonald, S. Reynolds, G. Krowech and R. Howd, J. Environ. Sci. Health, Part C: Environ. Carcinog. Ecotoxicol. Rev., 2006, 24, 155 CrossRef CAS PubMed
. - M. Costa, Toxicol. Appl. Pharmacol., 2003, 188, 1 CrossRef CAS PubMed
. - M. Bhaumik, A. Maity, V. Srinivasu and M. S. Onyango, Chem. Eng. J., 2012, 181–182, 323 CrossRef CAS
. - M. K. Aroua, F. M. Zuki and N. M. Sulaiman, J. Hazard. Mater., 2007, 147, 752 CrossRef CAS PubMed
. - T. D. de Castro, A. D. Neto, A. M. Moura, E. B. Neto and E. P. Telemaco, Langmuir, 2001, 17, 4256 CrossRef
. - A. M. Yusof and N. A. N. Malek, J. Hazard. Mater., 2009, 162, 1019 CrossRef CAS PubMed
. - M. Chiha, M. H. Samar and O. Hamdaoui, Desalination, 2006, 194, 69 CrossRef CAS
. - N. Ballav, A. Maity and S. B. Mishra, Chem. Eng. J., 2012, 181, 323 Search PubMed
. - Y. Wu, S. Wang, D. G. Streets, J. Hao, M. Chan and J. Jiang, Environ. Sci. Technol., 2006, 40, 5312 CrossRef CAS PubMed
. - R. Nakagawa and Y. Yumita, Chemosphere, 1998, 37, 1483 CrossRef CAS PubMed
. - A. B. Mukherjee, R. Zevenhoven, J. Brodersen, L. D. Hylander and P. Bhattacharya, Resour., Conserv. Recycl., 2004, 42, 155 CrossRef
. - M. C. Alvim-Ferraz and S. A. Afonso, Environ. Sci. Technol., 2003, 37, 3152 CrossRef CAS PubMed
. - C. Namasivayam and K. Kadirvelu, Carbon, 1999, 37, 79 CrossRef CAS
. - H. H. Harris, I. J. Pickering and G. N. George, Science, 2003, 301, 1203 CrossRef CAS PubMed
. - C. Sanfeliu, J. Sebastià, R. Cristòfol and E. R. Farré, Neurotoxic. Res., 2003, 5, 283 CrossRef
. - E. M. Nolan and S. J. Lippard, Chem. Rev., 2008, 108, 3443 CrossRef CAS PubMed
. - V. Chandra and K. S. Kim, Chem. Commun., 2011, 47, 3942 RSC
. - M. Choi and J. Jang, J. Colloid Interface Sci., 2008, 325, 287 CrossRef CAS PubMed
. - H. Javadian and M. Taghavi, Appl. Surf. Sci., 2014, 289, 487 CrossRef CAS
. - C. M. Castilla, M. A. Merino, M. L. Ramón and J. R. Utrilla, Langmuir, 2004, 20, 8142 CrossRef PubMed
. - H. Horiguchi, H. Teranishi, K. Niiya, K. Aoshima, T. Katoh, N. Sakurangana and M. Kasuya, Arch. Toxicol., 1994, 68, 632 CrossRef CAS PubMed
. - K. Kadirvelu and C. Namasivayam, Adv. Environ. Res., 2003, 7, 471 CrossRef CAS
. - M. Kobya, E. Demirbas, E. Senturk and M. Ince, Bioresour. Technol., 2005, 96, 1518 CrossRef CAS PubMed
. - T. S. Nawrot, J. A. Staessen, H. A. Roels, E. Munters, A. Cuypers and T. Richart, et al., Biometals, 2010, 23, 769 CrossRef CAS PubMed
. - G. Kazantzis, Biometals, 2004, 17, 493 CrossRef CAS PubMed
. - M. Ghorbani and H. Eisazadeh, Composites, Part B, 2013, 45, 1 CrossRef CAS
. - L. Järup, L. Hellström, T. Alfvén, M. D. Carlsson, A. Grubb and B. Persson, et al., Occup. Environ. Med., 2000, 57, 668 CrossRef
. - R. Karthik and S. Meenakshi, Int. J. Biol. Macromol., 2015, 78, 157 CrossRef CAS PubMed
. - Y. Zhang, H. Chi, W. Zhang, Y. Sun, Q. Liang and Y. Gu, et al., Nano-Micro Lett., 2014, 6, 80 CrossRef CAS
. - H. Kozlowski, A. J. Klos, J. Brasun, E. Gaggelli, D. Valensin and G. Valensin, Coord. Chem. Rev., 2009, 253, 2665 CrossRef CAS
. - J. Sebastian and D. Srinivas, Appl. Catal., A, 2015, 506, 163 CrossRef CAS
. - M. Omraei, H. Esfandian, R. Katal and M. Ghorbani, Desalination, 2011, 271, 248 CrossRef CAS
. - J. Ng, W. Cheung and G. McKay, J. Colloid Interface Sci., 2002, 255, 64 CrossRef CAS PubMed
. - G. Qiu, Q. Xie, H. Liu, T. Chen, J. Xie and H. Li, Appl. Clay Sci., 2015, 118, 107 CrossRef CAS
. - F. Fu and Q. Wang, J. Environ. Manage., 2011, 92, 407 CrossRef CAS PubMed
. - M. R. Awual, M. Ismael, M. A. Khaleque and T. Yaita, J. Ind. Eng. Chem., 2014, 20, 2332 CrossRef CAS
. - I. Shibi and T. Anirudhan, Chemosphere, 2005, 58, 1117 CrossRef CAS PubMed
. - A. Naeem, M. Saddique, S. Mustafa, S. Tasleem, K. Shah and M. Waseem, J. Hazard. Mater., 2009, 172, 124 CrossRef CAS PubMed
. - S. Rengaraj and S. H. Moon, Water Res., 2002, 36, 1783 CrossRef CAS PubMed
. - A. Shahat, M. R. Awual and M. Naushad, Chem. Eng. J., 2015, 271, 155 CrossRef CAS
. - S. Peng, W. Wang, X. Li and Y. Yen, Chemosphere, 2004, 57, 839 CrossRef CAS PubMed
. - M. I. Kandah and J. L. Meunier, J. Hazard. Mater., 2007, 146, 283 CrossRef CAS PubMed
. - E. Malkoc, J. Hazard. Mater., 2006, 137, 899 CrossRef CAS PubMed
. - G. Z. Kyzas and M. Kostoglou, Sep. Purif. Technol., 2015, 149, 92 CrossRef CAS
. - T. A. Kurniawan, G. Y. Chan, W. Lo and S. Babel, Chem. Eng. J., 2006, 118, 83 CrossRef CAS
. - A. Y. Men'shikova and T. Evseeva, Russ. J. Appl. Chem., 2003, 76, 822 CrossRef
. - A. D
browski, Z. Hubicki, P. Podkościelny and E. Robens, Chemosphere, 2004, 56, 91 CrossRef PubMed
. - Y. Takahashi, H. Kasai, H. Nakanishi and T. M. Suzuki, Angew. Chem., 2006, 118, 927 CrossRef
. - S. Shin and J. Jang, Chem. Commun., 2007, 41, 4230 RSC
. - V. J. Vilar, C. M. Botelho and R. A. Boaventura, Process Biochem., 2005, 40, 3267 CrossRef CAS
. - U. Wingenfelder, C. Hansen, G. Furrer and R. Schulin, Environ. Sci. Technol., 2005, 39, 4606 CrossRef CAS PubMed
. - A. Szabó, D. Gournis, M. Karakassides and D. Petridis, Chem. Mater., 1998, 10, 639 CrossRef
. - G. B. Samiey, C. Cheng and J. Wu, Materials, 2014, 7, 673 CrossRef
. - Z. Geng, Y. Lin, X. Yu, Q. Shen, L. Ma, Z. Li, N. Pan and X. Wang, J. Mater. Chem., 2012, 22, 3527 RSC
. - G. Crini, Prog. Polym. Sci., 2005, 30, 38 CrossRef CAS
. - P. X. Sheng, Y. Ting, J. P. Chen and L. Hong, J. Colloid Interface Sci., 2004, 275, 131 CrossRef CAS PubMed
. - G. Abid, A. Jrad, K. Nahdi and M. T. Ayadi, Desalination, 2009, 246, 595 CrossRef
. - H. Tamai, T. Kakii, Y. Hirota, T. Kumamoto and H. Yasuda, Chem. Mater., 1996, 8, 454 CrossRef CAS
. - A. Weidlich, K. Mangold and K. Jüttner, Electrochim. Acta, 2001, 47, 741 CrossRef
. - M. M. Abdi, A. Kassim, H. N. M. E. Mahmud, W. M. M. Yunus, Z. A. Talib and A. R. Sadrolhosseini, J. Mater. Sci., 2009, 44, 3682 CrossRef CAS
. - H. Zhao, W. Price, P. Teasdale and G. G. Wallace, React. Polym., 1994, 23, 213 CrossRef CAS
. - T. Yao, C. Wang, J. Wu, Q. Lin, H. Lv and K. Zhang, et al., J. Colloid Interface Sci., 2009, 338, 573 CrossRef CAS PubMed
. - H. T. Lee, Y. C. Liu and L. H. Lin, J. Polym. Sci., Part A: Polym. Chem., 2006, 44, 2724 CrossRef CAS
. - V. Truong, P. Lai, B. Moore, R. Muscat and M. Russo, Synth. Met., 2000, 110, 7 CrossRef CAS
. - H. N. M. E. Mahmud, A. Kassim, Z. Zainal and W. M. M. Yunus, J. Appl. Polym. Sci., 2005, 100, 4107 CrossRef
. - M. Redondo and C. Breslin, Corros. Sci., 2007, 49, 1765 CrossRef CAS
. - H. N. M. E. Mahmud, S. Hosseini and R. B. Yahya, Key Eng. Mater., 2014, 594, 793 Search PubMed
. - S. Deng and R. Bai, Water Res., 2004, 38, 2424 CrossRef CAS PubMed
. - A. Wei, S. German, S. Basak and K. Rajeshwar, J. Electrochem. Soc., 1993, 140, 60 CrossRef
. - R. Katal, M. Ghiass and H. Esfandian, J. Vinyl Addit. Technol., 2011, 17, 222 CrossRef CAS
. - F. J. Rodríguez, S. Gutiérrez, J. G. Ibanez, J. L. Bravo and N. Batina, Environ. Sci. Technol., 2000, 34, 2018 CrossRef
. - A. Kassim, H. N. M. E. Mahmud and F. Adzmi, Mater. Sci. Semicond. Process., 2007, 10, 246 CrossRef CAS
. - W. W. Ngah and M. Hanafiah, Bioresour. Technol., 2008, 99, 3935 CrossRef PubMed
. - S. Deng, G. Yu, S. Xie, Q. Yu, J. Huang, Y. Kuwaki and M. Iseki, Langmuir, 2008, 24, 10961 CrossRef CAS PubMed
. - A. K. Ioanis and A. I. Zouboulis, Water Res., 2002, 36, 5141 CrossRef
. - H. Eisazadeh, World Appl. Sci. J., 2008, 3, 10 Search PubMed
. - L. Seid, D. Chouder, N. Maouche, I. Bekas and N. Barka, J. Taiwan Inst. Chem. Eng., 2014, 45, 2969 CrossRef CAS
. - T. Hasani and H. Eisazadeh, Synth. Met., 2013, 175, 15 CrossRef CAS
. - R. Ansari and N. K. Fahim, React. Funct. Polym., 2007, 67, 367 CrossRef CAS
. - R. Karthik and S. Meenakshi, Synth. Met., 2014, 198, 181 CrossRef CAS
. - N. Ballav, H. J. Choi, S. B. Mishra and A. Maity, J. Ind. Eng. Chem., 2014, 20, 4085 CrossRef CAS
. - M. Bhaumik, K. Setshedi, A. Maity and M. S. Onyango, Sep. Purif. Technol., 2013, 110, 11 CrossRef CAS
. - M. Bhaumik, A. Maity, V. Srinivasu and M. S. Onyango, J. Hazard. Mater., 2011, 190, 381 CrossRef CAS PubMed
. - S. K. Li, X. F. Lu, X. Li, Y. P. Xue, C. C. Zhang and J. Y. Lei, et al., J. Colloid Interface Sci., 2012, 378, 30 CrossRef CAS PubMed
. - A. Sahmetlioglu, E. Yilmaz, E. Aktas and M. Soylak, Talanta, 2014, 119, 447 CrossRef PubMed
. - J. Wang, K. Pan, Q. He and B. Cao, J. Hazard. Mater., 2013, 44, 121 CrossRef PubMed
. - Y. Wang, B. Zou, T. Gao, X. Wu, S. Lou and S. Zhou, J. Mater. Chem., 2012, 22, 9034 RSC
. - A. C. Alicia, C. M. Juan, M. Luis, A. S. A. Cesar, G. B. A. Kleber and P. D. Celso, Chem. Eng. J., 2015, 281, 826 CrossRef
. - S. Li, X. Lu, Y. Xue, J. Lei, T. Zheng and C. Wang, PLoS One, 2012, 7, 1 Search PubMed
. - K. Z. Setshedi, M. Bhaumik, M. S. Onyango and A. Maity, Chem. Eng. J., 2015, 262, 921 CrossRef CAS
. - V. P. Chauke, A. Maity and A. Chetty, J. Mol. Liq., 2015, 211, 71 CrossRef CAS
. - H. Wang, X. Yuan, Y. Wu, X. Chen, L. Leig and H. Wang, et al., Chem. Eng. J., 2015, 262, 597 CrossRef CAS
. - K. Z. Setshedi, M. Bhaumik, S. Songwane, M. S. Onyango and A. Maity, Chem. Eng. J., 2013, 222, 186 CrossRef CAS
. - W. Yao, T. Ni, S. Chen, H. Li and Y. Lu, Compos. Sci. Technol., 2014, 99, 15 CrossRef CAS
. - A. M. Muliwa, T. Y. Leswifi, M. S. Onyango and A. Maity, Sep. Purif. Technol., 2016, 158, 250 CrossRef CAS
. - U. Baig, R. A. K. Rao, A. A. Khan, M. M. Sanagi and M. A. Gondal, Chem. Eng. J., 2015, 280, 494 CrossRef CAS
. - C. W. Lim, K. Song and S. H. Kim, J. Ind. Eng. Chem., 2012, 18, 24 CrossRef CAS
. - M. Shafiabadi, A. Dashti and H. A. Tayebi, Synth. Met., 2016, 212, 154 CrossRef CAS
. - H. Javadian, F. Z. Sorkhordi and B. B. Koutenaei, J. Ind. Eng. Chem., 2014, 20, 3678 CrossRef CAS
. - M. Šćiban, M. Klašnja and B. Škrbić, J. Hazard. Mater., 2006, 136, 266 CrossRef PubMed
. - A. Bhattacharya, T. Naiya, S. Mandal and S. Das, Chem. Eng. J., 2008, 137, 529 CAS
. - A. Ahmad, M. Rafatullah, O. Sulaiman, M. H. Ibrahim, Y. Y. Chii and B. M. Siddique, Desalination, 2009, 247, 636 CrossRef CAS
. - M. S. Rahman and M. R. Islam, Chem. Eng. J., 2009, 149, 273 CrossRef CAS
. - M. Ghorbani and H. Eisazadeh, Synth. Met., 2012, 162, 1429 CrossRef CAS
. - Y. Yu, C. Ouyang, Y. Gao, Z. Si, W. Chen and Z. Wang, et al., J. Pol. Sci. A-Polym. Chem., 2005, 43, 6105 CrossRef CAS
. - A. Maity and S. S. Ray, Macromole. Rapid Commun., 2008, 29, 1582 CrossRef CAS
. - J. Wang, K. Pan, Q. He and B. Cao, J. Hazard. Mater., 2013, 244, 121 CrossRef PubMed
. - A. V. Skorb and D. V. Andreeva, Polym. Chem., 2013, 4, 4834 RSC
. - R. Senthurchelvan, Y. Wang, S. Basak and K. Rajeshwar, J. Electrochem. Soc., 1996, 143, 44 CrossRef CAS
. - S. Zhang, M. Zeng, W. Xu, J. Li, J. Li and J. Xu, et al., Dalton Trans., 2013, 42, 7854 RSC
. - A. Fusalba and D. Bélanger, J. Phys. Chem. B, 1999, 103, 9044 CrossRef
. - M. Yoonessi and J. R. Gaier, ACS Nano, 2010, 4, 7211 CrossRef CAS PubMed
. - A. K. Geim and K. S. Novoselov, Nat. Mater., 2007, 6, 183 CrossRef CAS PubMed
. - C. Neto, F. Guinea, N. Peres, K. S. Novoselov and A. K. Geim, Rev. Mod. Phys., 2009, 81, 109 CrossRef
. - C. N. R. Rao, K. Biswas, K. Subrahmanyam and A. Govindaraj, J. Mater. Chem., 2009, 19, 2457 RSC
. - S. Konwer, R. Boruah and S. K. Dolui, J. Electron. Mater., 2011, 40, 2248 CrossRef CAS
. - S. Stankovich, D. A. Dikin, G. H. Dommett, K. M. Kohlhaas, E. J. Zimney and E. A. Stach, et al., Nature, 2006, 442, 282 CrossRef CAS PubMed
. - A. Liu, C. Li, H. Bai and G. Shi, J. Phys. Chem. C, 2010, 114, 22783 CAS
. - A. Qin, T. Gong, Y. Cho, C. Lee and T. kim, Polym. Chem., 2014, 5, 4466 RSC
. - A. Zhu, J. Yang and B. Deng, J. Hazard. Mater., 2009, 166, 866 CrossRef PubMed
. - A. H. Cai and C. Q. Jia, Ind. Eng. Chem. Res., 2010, 49, 2716 CrossRef
. - S. Maeda and S. P. Armes, Chem. Mater., 1995, 7, 171 CrossRef CAS
. - Z. Chen, L. Zhou, F. Zhang, C. Yu and Z. Wei, Appl. Surf. Sci., 2012, 258, 5291 CrossRef CAS
. - S. M. R. Jiménez, S. M. González and A. H. Maldonado, Microporous Mesoporous Mater., 2010, 132, 470 CrossRef
. - A. Shahbazi, H. Younesi and A. Badiei, Chem. Eng. J., 2011, 168, 505 CrossRef CAS
. - A. Mercier and T. J. Pinnavaia, Environ. Sci. Technol., 1998, 32, 2749 CrossRef
. - H. Jiang, T. Zhao, C. Li and J. Ma, J. Mater. Chem., 2011, 21, 3818 RSC
.
|
This journal is © The Royal Society of Chemistry 2016 |
Click here to see how this site uses Cookies. View our privacy policy here.