DOI:
10.1039/C6RA04538C
(Paper)
RSC Adv., 2016,
6, 35771-35777
Efficient L-lactic acid production from sweet sorghum bagasse by open simultaneous saccharification and fermentation
Received
20th February 2016
, Accepted 2nd April 2016
First published on 5th April 2016
Abstract
As an important industrial feedstock with various applications, most lactic acid is currently fermented from refined food carbohydrates. To research the feasibility of using non-food feedstock for L-lactic acid production, sweet sorghum bagasse (SSB) as an important lignocellulosic biomass was used by Bacillus coagulans LA1507 in open simultaneous saccharification and fermentation (SSF). In the fed-batch SSF process with a stepwise controlled pH, a cellulase dose of 25 FPU g−1 pretreated SSB and 15 g L−1 corn steep liquor powder (CSLP) were used to produce L-lactic acid from 17.5% (w/v) stover, and B. coagulans LA1507 provided a balanced result which contained a product titer of 111 g L−1, a yield of 0.437 g g−1 crude SSB and an average productivity of 1.59 g L−1 h−1. The process introduces an effective way to produce L-lactic acid from abundant SSB.
1 Introduction
As one versatile block-building chemical used in food, pharmaceuticals, leather, textiles and chemical industries, lactic acid can be produced in two ways: chemical synthesis and fermentation. The fermentation process is attractive because of its advantages including the production of optically pure lactic acid, with a low production temperature, and decreased energy consumption.1 Approximately 90% of the commercially available lactic acid is produced by submerged fermentation of corn, and the corn feedstock accounts for nearly 70% of the overall production cost in the fermentation process.2 The purification cost of lactic acid production based on refined materials should be significantly reduced. However, this is still economically unfavourable because the refined carbohydrates are so expensive and they need much more nutrient supplementation than the complex substrate for the fastidious LA bacteria.3 Therefore, it is highly desired to develop an efficient and cost-effective process for lactic acid fermentation from inexpensive and non-food substrates.4 Among the cheap raw materials, cellulosic materials, which are abundant and renewable, are receiving great attention of researchers.
Sweet sorghum (Sorghum bicolor (L.) Moench) has been considered an attractive raw material for the production of biofuel, owing to its greater biomass yield per hectare, high photosynthetic efficiency, and wide adaptability to harsh environmental conditions.5 And sweet sorghum stalk contains 43.6–58.2% soluble sugars,6 22.6–47.8% insoluble cellulose and hemicelluloses7 and 14.1–20.8% lignin8 as previous literature reported. Recently, the common approach of bioethanol production from sweet sorghum is based on sorghum juice fermentation.9–11 And a large amount of sweet sorghum bagasse (SSB) is produced as biomass waste during the process of crushing and juice pressing. Lignocellulosic biomass, especially agro stovers, provides a promising source of carbohydrate for fermentation that does not compete with other food sources.12 Numerous studies revealed that lactic acid can be produced from various lignocellulosic biomasses, such as wheat straw, corn stover, hemp hurds and bagasse sulfite pulp.12–16 However, according to previous studies, the structure and biological composition of SSB are quite different from those of other related lignocellulosic biomasses such as corn stalk or sugar cane.17,18 And few reports have studied fermentative production of lactic acid from SSB.
In this study, to efficiently produce L-lactic acid from SSB, a new thermophilic B. coagulans LA1507 was isolated and characterized for L-lactic acid fermentation. And an open SSF process was investigated to improve enzymatic efficiency and reduce production cost. The cellulase concentration and stover loading were particularly optimized, and CSLP was used instead of YE & synthetic salts. In addition, high-titer L-lactic acid production using fed-batch SSF based on SSB was further studied, and a stepwise controlled pH was adopted to obtain balanced results maintaining product titer, yield and average productivity all at relative high levels.
2 Methods
2.1 Strain identification and primary characterization
One strain, designated as LA1507, was isolated and identified by analysis of 16S rDNA genes. The 16S rDNA sequence of the isolated strain was deposited in the GenBank database under accession number KU196783. The genomic DNA of the strain was extracted using the Axygen Genomic DNA Miniprep kit (Axygen, Hangzhou, China). And the 16S rDNA was amplified by PCR using the universal primers 27f and 1492r.12 PCR products were then analyzed and sequenced by BGI (Beijing, China). The 16S rDNA sequence obtained was blasted in the NCBI GenBank database against several LA-producing Bacillus strains. And the neighbor-joining method with MEGA5 was used to construct a phylogenetic tree.19
2.2 Materials and culture media
Sweet sorghum was cultivated on an experimental plot by the Chinese Academy of Agricultural Sciences at Shunyi District, Beijing. Yeast extract, beef extract and soya peptone were purchased from Aobox Biotechnology Co., Ltd. (Beijing, China). And CSLP was provided by Beijing Mannafeed International Group (Beijing, China). Cellulase used in this work was provided by Tianfeng Bioengineering Corporation (Hebei, China). And the specific activities of cellulase was 78 FPU ml−1 according to the manufacturers' data. And all other chemicals used were reagent grade.
The medium for agar slant and inoculum preparation was as previously described.20 Aerobic growth medium, which was used for microorganism culture, contained 10 g L−1 glucose and 15 g L−1 CSLP. And fermentation medium consisted of NaOH-pretreated SSB and cellulase on the basis of aerobic growth medium. In batch fermentations, NaOH-pretreated SSB was added into aerobic growth medium with a concentration of 10% (w/v), while the concentration reached 17.5% (w/v) in fed-batch fermentation. Cellulase was added at a dose of 25 FPU g−1 stover.
2.3 Pretreatment of raw materials
After squeezing out the fresh juice of sweet sorghum, the stover was soaked in hot water to remove residual sugars. The chopped stalk was dried at 80 °C to a constant weight and milled to about 850 μm in a laboratory grinder. The dry SSB contained 38.47% glucan, 21.24% xylan, 2.13% arabinian and 21.41% lignin. Then, it was pretreated at 118 °C for 80 min by 2% (w/v) NaOH at a 10% (w/v) loading. The slurry obtained was further treated by filtration to achieve solid–liquid separation. The solid fraction was washed with water until its pH decreased to 7.0. The NaOH-pretreated SSB contained 51.67% glucan, 18.5% xylan, 1.45% arabinian and 4.3% lignin. Totally, 599.4 g pretreated SSB was obtained from 1000 g dry SSB.
2.4 Microorganism cultivation conditions
B. coagulans LA1507 was maintained on modified De Man–Rogosa–Sharpe (mMRS) agar slant. After incubated at 50 °C for 24 h, the full grown slant was stored at 4 °C. And the stock culture was transferred to fresh mMRS agar slants monthly. In seed culture preparation, the cells were transferred to liquid mMRS medium containing 40 g L−1 glucose in 250 ml Erlenmeyer flasks and were incubated for 20 h at 50 °C and 150 rpm on a rotary shaker. The seed culture was then inoculated into aerobic growth medium in 1 L fermentor (BLBIO-mini-1GJ, Bailun biological technology co., LTD. Shanghai, China) or 5 L fermentor (SGB-5L, Changzhou Sungod Bio-technology & Engineering Equipment Co., Ltd. Jiangsu, China) for aerobic cell growth. The inoculation rate was 10% (v/v).
2.5 Aerobic cultivation and fermentation
A two-stage fermentation was performed in this study. The whole process was conducted without sterilization, which temperature was kept at 50 °C. In aerobic cultivation, the pH was maintained at 6.5. The aeration rate and agitation speed were 2 vvm and 400 rpm, respectively. In fermentation, carbon sources (glucose, xylose or pretreated SSB) was added into aerobic growth medium containing active B. coagulans LA1507 cells. An agitation speed of 260 rpm was adopted without aeration, and the pH was automatically controlled by 33% Ca(OH)2. When pretreated SSB was used as carbon source, cellulase was added at a dose of 25 FPU g−1 stover.
In order to examine the effect of temperature on strain growth and L-lactic acid fermentation, different temperatures ranging from 45–60 °C were used in 1 L fermentor containing 15 g L−1 YE, 1 g L−1 (NH4)2SO4, 0.4 g L−1 KH2PO4, 0.3 g L−1 MgSO4·7H2O and 2.5 g L−1 NaCl. The carbon source in fermentation was 50 g L−1 glucose or xylose. In the experiments studying CSLP as an alternative for YE and synthetic salts, 1 L fermentor containing 15 g L−1 CSLP or YE & synthetic salts was used, and L-lactic acid fermentation from 50 g L−1 glucose or xylose was studied. In addition, experiments in 5 L fermentor containing YE medium were conducted to confirm the efficient L-lactic acid production based on glucose and xylose by strain LA1507.
In the process of SSF, two main factors containing cellulase dose and stover loading were studied. In order to investigate the effect of cellulase on SSF, different cellulase concentrations ranging from 5 to 30 FPU g−1 stover were adopted with a stover loading of 10% (w/v). In stover loading optimization, the stover concentrations ranging from 2.5–15% (w/v) were used with a cellulase dose of 25 FPU g−1 stover. The pH in batch fermentations was maintained at 5.6. However, high solid loading in SSF is always required to increase lactic acid titer for industrial organic acid production (generally above 100 g L−1).21 As a high product titer is benefit for reducing costs of lactic acid separation and concentration, a fed-batch SSF was conducted. It is worth to mention that, in previous study, because of the indigenous thermophilic bacteria from CSLP, an efficient open fermentation of L-lactic acid based on white rice bran cannot be achieved solely relying on temperature at 50 °C. The calcium lactate produced during fermentation could be combined with pH for indigenous bacteria suppression.22 Thus, in order to achieve a balanced process in which L-lactic acid concentration, productivity and yield were all at relative high levels, a stepwise controlled pH was adopted in fed-batch SSF based on pretreated SSB.
2.6 Analytical methods
Product (calculated as L-lactic acid) and glucose were measured by SBA-40C biosensor analyzer (Institute of Biology, Shandong Province Academy of Sciences, Shandong, China),23 and the fermentation broth was centrifuged to remove insoluble substances before properly diluted. The SBA-40C biosensor analyzer, which was based on immobilized enzyme sensor, mainly included a reaction cell equipped with two hydrogen peroxide electrodes. The immobilized glucose oxidase membrane and L-lactate oxidase were covered in the surface of each electrode. Glucose or L-lactate in the fermentation broth was in contact with oxidase membrane, and H2O2 was produced on the corresponding membrane. The amount of H2O2 accumulated on each electrode was linearly proportional to glucose and L-lactate concentration. Finally, the hydrogen peroxide detected the H2O2 and converted its amount into an electrical signal. The electrical signal was further calculated as glucose or L-lactic acid concentration which was shown on the screen. The cell density was measured by a spectrophotometer (UV-1100, Beijing Eternal Cause Instrument Co., Ltd, China) at 620 nm.24 Monosaccharides concentration was determined by high-performance liquid chromatography (HPLC) equipped with a HPX-87P carbohydrate analysis column (Bio-Rad Labs, USA) operated at 80 °C using deionized water as mobile-phase (0.6 ml min−1).25 And acetic acid was determined using HPLC as previously reported.22 The composition of crude and pretreated SSB were determined according to the National Renewable Energy Laboratory (NREL) protocol.26
3 Results and discussion
3.1 Identification of strain B. coagulans LA1507
The 16S rDNA sequence showed 99% similarity to B. coagulans 36D1 (DQ297926) by BLAST analysis in NCBI, and the isolated strain was designated as B. coagulans LA1507. Based on the 16S rDNA sequences of several LA-producing Bacillus strains in the NCBI database and the one studied, a phylogenetic tree was constructed. As shown in Fig. 1, Bacillus sp. XZL9, which is an efficient producer of L-lactic acid from corncob molasses,27 clusters together with strain LA1507 in phylogenetic relationship, indicating the closeness of the two strains. And the relative low bootstrap values indicates the independent status of B. coagulans LA1507 in phylogenetic relationship compared with these referential strains (Fig. 1).
 |
| Fig. 1 Phylogenetic relationship of B. coagulans LA1507 and other known LA-producing Bacillus strains based on their 16S rRNA sequences. Bootstrap values are marked on the branches and the scale represents 10% divergence between sequences. | |
In addition, most of these referential strains have shown potential in L-lactic acid production from lignocellulosic biomass with the advantages of relative high fermentation temperature and efficient xylose metabolic capability. B. coagulans LA204 was used for L-lactic acid SSF from corn stover, and the yield and titer reached 0.68 g g−1 and 97.59 g L−1, respectively.12 B. coagulans JI12 was reported to produce L-lactic acid from acid hydrolysate of oil palm empty fruit bunch, and 59.2 g L−1 lactic acid was obtained with a yield of 97%.28 Other lignocellulosic materials including wheat straw and hemp hurds were also used for LA production by B. coagulans strains.13,14 Furthermore, open fermentative production of lactic acid could be achieved by virtue of the thermophilic feature of Bacillus coagulans,24 and the relative high fermentation temperature is benefit to establish conditions optimal for both biocatalysis: enzymes and microorganism in SSF.16 Thus, B. coagulans LA1507 was further characterized to efficiently produce L-lactic acid from SSB by open SSF.
3.2 Characterization of B. coagulans LA1507 for L-lactic acid fermentation
Usually, Bacillus coagulans was well known in terms of growing and fermenting at temperature which ranged from 50 °C to 60 °C.14,28,29 In order to investigate the effect of temperature on B. coagulans LA1507, different temperatures ranging from 45–60 °C were adopted, and 50 g L−1 glucose or xylose were used as carbon source in fermentation. The experiments were conducted in 1 L fermentor, and the pH was maintained at 6.50 by 33% Ca(OH)2. As shown in Fig. 2a, the maximum L-lactic acid was produced at 50 °C which providing 48 g L−1 and 46 g L−1 L-lactic acid from 50 g L−1 glucose and xylose, respectively. And the highest cell density (14.1) after aerobic cultivation was also obtained at 50 °C indicating this temperature is optimal for the growth of strain LA1507.
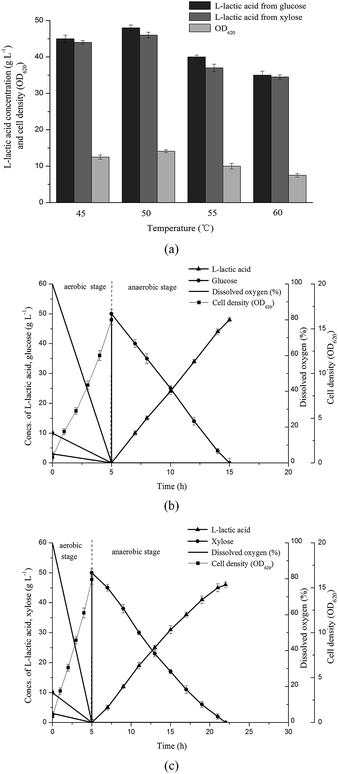 |
| Fig. 2 Characterization of B. coagulans LA1507 based on (a) temperature, and L-lactic acid fermentation using (b) glucose and (c) xylose. The error bars in the figure indicate the standard deviations of three parallel replicates. | |
To further confirm the efficient L-lactic acid production based on glucose and xylose by strain LA1507, experiments in 5 L fermentor containing 50 g L−1 glucose or xylose were conducted as shown in Fig. 2b and c. The yield and productivity of L-lactic acid from glucose were 97.3% and 4.8 g L−1 h−1, respectively. However, the yield and productivity reduced to 93.2% and 2.71 g L−1 h−1 when xylose was used as carbon source, indicating the strain LA1507 was a carbon catabolite repression positive (CCR-positive) strain. Although the cell growth of LA1507 in aerobic cultivation was similar to B. coagulans (NBRC 12583),24 the results indicated B. coagulans LA1507 had strong ability to ferment lignocellulosic hydrolysates into L-lactic acid. The effect of pH values on bacteria growth and L-lactic acid fermentation was examined, and the optimal pH for strain growth and fermentation was found at around 6.50. As one cheap nitrogen source containing rich inorganic salts, CSLP was studied to replace YE and synthetic salts. Experiments were conducted in 1 L fermentor containing 15 g L−1 CSLP or YE & synthetic salts. When glucose and xylose were used as carbon source, the medium containing 15 g L−1 CSLP provided a L-lactic acid titer of 47 g L−1 and 44 g L−1, and the medium containing YE & synthetic salts provided a L-lactic acid titer of 48.5 g L−1 and 44.5 g L−1, respectively. These results indicated CSLP could replace YE & synthetic salts without a significant drop in product titer, and it has been confirmed in L-lactic acid fermentation based on white rice bran.22 Thus, in order to reduce raw material cost in L-lactic acid fermentation, CSLP was used in the following experiments.
3.3 Effect of cellulase concentration on L-lactic acid fermentation
In order to investigate the effect of cellulase concentration on L-lactic acid production, different cellulase concentrations were adopted in unsterilized SSF with a stover loading of 10% (w/v). After aerobic cultivation, a cell density (OD620) as high as 16.0 could be obtained in this study (Fig. 2), and it was 1.43 times higher than that in the aerobic cultivation of B. coagulans (NBRC 12583).24 In the initial stage, the amount of carbon source could be a limiting factor for productivity. As shown in Fig. 3a, a high L-lactic acid productivity in the first 20 h could be obtained with a high cellulase concentration, which is caused by the fast increasement of glucose and xylose concentrations. Similar L-lactic acid titers of 63 g L−1 and 64 g L−1 were obtained when cellulase concentrations of 25 FPU g−1 and 30 FPU g−1 pretreated SSB were adopted. Further increasing cellulase had no significant influence on final product titer, which was also observed in bio-ethanol fermentation based on reed,30 indicating the cellulase dose of 25 FPU g−1 stover was just suitable to satisfy the enzymatic hydrolysis of pretreated SSB in this study.
 |
| Fig. 3 Effects of cellulase concentration on production of (a) L-lactic acid, (b) acetic acid and consumption of (c) glucose, (d) xylose by strain B. coagulans LA1507 in unsterilized SSF. Symbols represent different cellulase concentrations (FPU g−1 pretreated SSB) were (■) 5, (●) 10, (▲) 15, (▼) 20, (♦) 25 and (◀) 30, respectively. The temperature and pH was maintained at 50 °C and 5.6. A 1 L fermentor was used with a stover loading of 10% (w/v), and the error bars in the figure indicate the standard deviations of three parallel replicates. | |
As the growth of cellulase concentration, the top values of glucose and xylose concentration in SSF increased, which is shown in Fig. 3c and d. As expected, glucose and xylose were sequencely consumed and the results confirmed that B. coagulans LA1507 was a carbon catabolite repression positive (CCR-positive) strain, which was also reported by Zhang et al.14 when B. coagulans IPE22 was used in lactic acid production from mixed sugars containing glucose and xylose. In addition, the tendency of acetic acid was similar to that of L-lactic acid, and acetic acid concentrations ranging from 2.81 g L−1 to 3.86 g L−1 were obtained under different cellulase concentrations (Fig. 3b). Totally, the L-lactic acid yield under different cellulase concentrations of 5 FPU g−1, 10 FPU g−1, 15 FPU g−1, 20 FPU g−1, 25 FPU g−1 and 30 FPU g−1 pretreated SSB were 0.306 g g−1, 0.421 g g−1, 0.541 g g−1, 0.625 g g−1, 0.764 g g−1 and 0.772 g g−1, respectively. Thus, the results indicated that the cellulase concentration of 25 FPU g−1 pretreated SSB should be adopted with a high L-lactic acid titer and yield while controlling the cellulase concentration at a relative low level.
3.4 Effect of stover loading on L-lactic acid production
As shown in Fig. 4a and b, the final L-lactic acid titer and acetic acid concentration increased as the growth of stover concentrations. The maximum L-lactic acid titer and acetic acid concentration under 15% (w/v) stover loading were 74 g L−1 and 6.22 g L−1, respectively. It is worth mentioning that the higher stover loading was used, the lower product yield was observed. The L-lactic acid yield of 2.5%, 5%, 7.5%, 10%, 12.5% and 15% stover loadings were 0.780 g g−1, 0.774 g g−1, 0.763 g g−1, 0.762 g g−1, 0.624 g g−1 and 0.592 g g−1, respectively. The product titer didn't increase proportionally with the stover loading, which could be resulted from the feedback inhibition in low pH (5.6). A significant decline of product yield could be observed as the accumulation of L-lactic acid at a certain pH level. And the yield of L-lactic acid dropped from 93.9% to 74.7% in previous study when the total sugar concentration increased from 44.38 g L−1 to 100.1 g L−1 at pH 6.25.31 In comparison to other lactic acid producer (Lactobacillus), strain of B. coagulans was more sensitive to lactic acid.32,33 In addition, the accumulation of glucose and xylose significantly occurred when stover loading exceeded 10% (w/v) (Fig. 4c and d) reflecting the decline in metabolic ability of B. coagulans LA1507 under feedback inhibition. Considering the L-lactic acid titer and yield, 10% (w/v), which provided relative high levels of both product titer (66 g L−1) and yield (0.762 g g−1), was adopted as the initial stover loading in the following experiments, and 4.54 g L−1 acetic acid was obtained at the same time.
 |
| Fig. 4 Effects of stover loading on production of (a) L-lactic acid, (b) acetic acid and consumption of (c) glucose, (d) xylose by strain B. coagulans LA1507 in unsterilized SSF. Symbols represent different stover concentrations (w/v) were (■) 2.5%, (●) 5%, (▲) 7.5%, (▼) 10%, (♦) 12.5% and (◀) 15%, respectively. The temperature and pH was maintained at 50 °C and 5.6. A 1 L fermentor was used, and the cellulase concentration was 25 FPU g−1 pretreated SSB. The error bars in the figure indicate the standard deviations of three parallel replicates. | |
3.5 High-titer L-lactic acid production using fed-batch SSF based on pretreated SSB
On the basis of above results, CSLP and NaOH-pretreated SSB were used in fed-batch SSF under unsterilized conditions, and the fermentation was performed in a 5 L fermentor. As the interference of lignocellulosic biomass in fermentation, the cell density (OD620) was only determined in aerobic stage. As shown in Fig. 5, a cell density (OD620) of 15.8 was obtained at the end of aerobic stage, and dissolved oxygen dropped from 100% to 0% continuously, indicating the fast growth of B. coagulans LA1507. Three feedings of NaOH-pretreated SSB with a loading of 2.5% (w/v) were conducted. And cellulase was added with a dose of 25 FPU g−1 stover at the same time. In the batch fermentations (Fig. 3 and 4), a low pH (5.60) was adopted for contamination suppression. However, although Ca(OH)2 has been considered as an effective neutralizing agent other than NH3·H2O or NaOH,34 the accumulation of lactate usually caused a feedback inhibition on lactic acid bacteria,35 which would be more serious at low pH. Thus, a stepwise controlled pH which was gradually increased was adopted for indigenous bacteria suppression.
 |
| Fig. 5 L-Lactic acid fermentation by unsterilized SSF in 5 L fermentor using 10% NaOH-pretreated SSB at the initial stage, and the same stover was fed with a final loading of 17.5% (w/v). The temperature was maintained at 50 °C, and a stepwise controlled pH was adopted. Cellulase concentration was 25 FPU g−1. (●) L-lactic acid, (▲) acetic acid, (■) glucose, (×) xylose, (……) pH, (—) dissolved oxygen, and (▼) cell density. The error bars in the figure indicate the standard deviations of three parallel replicates. | |
From 5 to 15 h, in which stage pH was maintained at 5.60, a productivity of 5.10 g L−1 h−1 was obtained, and the L-lactic acid concentration reached 54 g L−1. In order to ease the product inhibition caused by low pH, the pH was maintained at 5.80 from 15 to 26.5 h, and L-lactic acid concentration increased to 74 g L−1 with a productivity of 1.74 g L−1 h−1. From 26.5 to 39.5 h, the pH was further increased to 6.00, and the L-lactic acid titer and productivity reached 94 g L−1 and 1.54 g L−1 h−1, respectively. Then, pH was maintained at 6.20 from 39.5 to 73 h, the productivity was 0.51 g L−1 h−1, and a final product titer of 111 g L−1 was obtained. Totally, the average productivity was 1.59 g L−1 h−1. Fig. 6 briefly showed a mass balance of the experimental process, and a L-lactic acid yield of 0.729 g g−1 NaOH-pretreated SSB or 0.437 g g−1 crude SSB was obtained by fed-batch SSF under unsterilized conditions. The yield of L-lactic acid in this study was near the results reported by Gandolfi et al.13 (0.42 g g−1 raw hemp hurds) and Zhang et al.14 (0.46 g g−1 wheat straw), which indicated the feasibility of this process for efficient L-lactic acid production from abundant SSB.
 |
| Fig. 6 The mass balance of the experimental process based on 1 kg of dry SSB. | |
4 Conclusions
This study introduces an efficient way to produce L-lactic acid from abundant SSB. A thermophilic L-lactic acid producer B. coagulans LA1507 was isolated and used in unsterilized SSF of NaOH-pretreated SSB. Identification and characterization of strain LA1507 were conducted, which indicated it has strong ability to ferment xylose into L-lactic acid. Cellulase concentration and stover loading of this process were optimized, and in the following fed-batch SSF with a stepwise controlled pH, the product titer, yield and average productivity reached 111 g L−1, 0.437 g g−1 crude SSB and 1.59 g L−1 h−1, respectively.
Acknowledgements
This work was supported in part by the National Basic Research Program of China (Grant No. 2013CB733600, 2012CB725200), the National Nature Science Foundation of China (Grant No. 21390202, 21476015), National High-Tech R&D Program of China (Grant No. 2014AA021903).
Notes and references
- K. Okano, T. Tanaka, C. Ogino, H. Fukuda and A. Kondo, Appl. Microbiol. Biotechnol., 2010, 85, 413 CrossRef CAS PubMed.
- M. A. Abdel-Rahman, Y. Tashiro and K. Sonomoto, Biotechnol. Adv., 2013, 31, 877 CrossRef CAS PubMed.
- R. P. John, G. S. Anisha, K. M. Nampoothiri and A. Pandey, Biotechnol. Adv., 2009, 27, 145 CrossRef CAS PubMed.
- Y. Li, L. M. Wang, J. S. Ju, B. Yu and Y. H. Ma, Bioresour. Technol., 2013, 142, 186 CrossRef CAS PubMed.
- E. M. Rubin, Nature, 2008, 454, 841 CrossRef CAS PubMed.
- S. Amaducci, A. Monti and G. Venturi, Ind. Crops Prod., 2004, 20, 111 CrossRef CAS.
- G. Antonopoulou, H. N. Gavala, I. V. Skiadas, K. Angelopoulos and G. Lyberatos, Bioresour. Technol., 2008, 99, 110 CrossRef CAS PubMed.
- B. Z. Li, V. Balan, Y. J. Yuan and B. E. Dale, Bioresour. Technol., 2010, 101, 1285 CrossRef CAS PubMed.
- O. Deesuth, P. Laopaiboon, P. Klanrit and L. Laopaiboon, Ind. Crops Prod., 2015, 74, 95 CrossRef CAS.
- Z. Luo, L. Wang and A. Shahbazi, Biomass Bioenergy, 2014, 67, 53 CrossRef CAS.
- M. Takaki, L. Tan, T. Murakami, Y. Q. Tang, Z. Y. Sun, S. Morimura and K. Kida, Ind. Crops Prod., 2015, 63, 329 CrossRef CAS.
- J. Hu, Z. Zhang, Y. Lin, S. Zhao, Y. Mei, Y. Liang and N. Peng, Bioresour. Technol., 2015, 182, 251 CrossRef CAS PubMed.
- S. Gandolfi, L. Pistone, G. Ottolina, P. Xu and S. Riva, Bioresour. Technol., 2015, 191, 59 CrossRef CAS PubMed.
- Y. Zhang, X. Chen, J. Luo, B. Qi and Y. Wan, Bioresour. Technol., 2014, 158, 396 CrossRef CAS PubMed.
- Z. Zheng, C. Cai, T. Jiang, M. Zhao and J. Ouyang, Appl. Biochem. Biotechnol., 2014, 173, 1896 CrossRef CAS PubMed.
- J. Zhou, J. Ouyang, M. Zhang and H. Yu, BioResources, 2014, 9, 2609 Search PubMed.
- A. Karp and I. Shield, New Phytol., 2008, 179, 15 CrossRef PubMed.
- W. Vermerris, J. Integr. Plant Biol., 2011, 53, 105 CrossRef PubMed.
- K. Tamura, D. Peterson, N. Peterson, G. Stecher, M. Nei and S. Kumar, Mol. Biol. Evol., 2011, 28, 2731 CrossRef CAS PubMed.
- Y. Wang, Z. Yang, P. Qin and T. Tan, RSC Adv., 2014, 4, 8907 RSC.
- K. Zhao, Q. Qiao, D. Chu, H. Gu, T. H. Dao, J. Zhang and J. Bao, Bioresour. Technol., 2013, 135, 481 CrossRef CAS PubMed.
- Y. Wang, D. Cai, M. He, Z. Wang, P. Qin and T. Tan, Bioresour. Technol., 2015, 198, 664 CrossRef CAS PubMed.
- Y. Wang, D. Cai, C. Chen, Z. Wang, P. Qin and T. Tan, Bioresour. Technol., 2015, 198, 658 CrossRef CAS PubMed.
- K. Ma, T. Maeda, H. You and Y. Shirai, Bioresour. Technol., 2014, 151, 28 CrossRef CAS PubMed.
- J. Zhang, X. Ma, J. Yu, X. Zhang and T. Tan, Bioresour. Technol., 2011, 102, 4585 CrossRef CAS PubMed.
- R. Sindhu, M. Kuttiraja, P. Binod, R. K. Sukumaran and A. Pandey, Renewable Energy, 2014, 62, 362 CrossRef CAS.
- L. Wang, B. Zhao, B. Liu, B. Yu, C. Ma, F. Su, D. Hua, Q. Li, Y. Ma and P. Xu, Bioresour. Technol., 2010, 101, 7908 CrossRef CAS PubMed.
- L. Ye, M. S. B. Hudari, X. Zhou, D. Zhang, Z. Li and J. C. Wu, Appl. Microbiol. Biotechnol., 2013, 97, 4831 CrossRef CAS PubMed.
- S. Akao, K. Maeda, S. Nakatani, Y. Hosoi, H. Nagare, M. Maeda and T. Fujiwara, Environ. Technol., 2012, 33, 1523 CrossRef CAS PubMed.
- J. Lu, X. Li, R. Yang, L. Yang, J. Zhao, Y. Liu and Y. Qu, Bioresour. Technol., 2013, 144, 539 CrossRef CAS PubMed.
- Y. Wang, H. Meng, D. Cai, B. Wang, P. Qin, Z. Wang and T. Tan, Bioresour. Technol., 2016, 211, 291 CrossRef CAS PubMed.
- M. S. Ou, L. O. Ingram and K. T. Shanmugam, J. Ind. Microbiol. Biotechnol., 2011, 38, 599 CrossRef CAS PubMed.
- M. A. Patel, M. S. Ou, R. Harbrucker, H. C. Aldrich, M. L. Buszko, L. O. Ingram and K. T. Shanmugam, Appl. Environ. Microbiol., 2006, 72, 3228 CrossRef CAS PubMed.
- S. Nakano, C. U. Ugwu and Y. Tokiwa, Bioresour. Technol., 2012, 104, 791 CrossRef CAS PubMed.
- E. Giraud, B. Lelong and M. Raimbault, Appl. Microbiol. Biotechnol., 1991, 36, 96 CrossRef CAS.
|
This journal is © The Royal Society of Chemistry 2016 |
Click here to see how this site uses Cookies. View our privacy policy here.