DOI:
10.1039/C6RA10404E
(Paper)
RSC Adv., 2016,
6, 62529-62539
Surface selective binding of 2,5-dimercapto-1,3,4-thiadiazole (DMTD) on silver and gold nanoparticles: a Raman and DFT study†
Received
22nd April 2016
, Accepted 22nd June 2016
First published on 23rd June 2016
Abstract
The binding affinity of 2,5-dimercapto-1,3,4-thiadiazole (DMTD) on noble metal silver (Ag) and gold (Au) nanoparticles (NPs) was investigated using the surface-enhanced Raman scattering (SERS) technique in combination with density functional theoretical (DFT) calculations. DMTD contains different anchoring groups; firstly the thiadiazole ring nitrogen (N) atoms, secondly the ring sulphur (S) atom, thirdly the thiocarbonyl S atoms and fourthly the ring π electrons. SERS combined with DFT calculations provided useful insight into the interaction between the molecule and metal, thus, illustrating the active sites of DMTD and the metal substrates being directly involved in binding. Raman, SERS and DFT studies were further exploited to study the tautomeric conformations of DMTD present in solid, aqueous solution and on the Ag and Au NP surfaces. The Raman spectrum indicated the existence of mainly the dithiol tautomer in the solid state with little contribution from the dithione and the thione–thiol forms. The pH-dependent Raman study of DMTD in aqueous solution clearly demonstrated the existence of different tautomers of DMTD with the changes in acidity or basicity. The SERS study of DMTD on the Ag NP surface with support from DFT calculations clearly suggested the abundance of mainly the thiol–thiolate tautomer at neutral pH while the dithiol form was predominant at acidic pH. The concentration-dependent SERS study on the Ag NP surface at acidic pH, indicated the preferential existence of the dithione form at low concentrations while the dithiol form is predominant at higher concentrations. On the Au NP surface at acidic pH, the abundance of the dithiol tautomer with slight contributions from the thione–thiol and the dithione forms are indicated. The binding is mainly through the thiadiazole ring N atom in case of Ag NPs whereas it is from the thiocarbonyl or the thiadiazole ring S atom in case of Au NPs. This selective binding observed in case of DMTD may be further extended in designing novel plasmonic nanostructures.
1. Introduction
Surface-enhanced Raman scattering (SERS) is a very sensitive spectroscopic technique that provides greatly enhanced Raman signals from Raman-active analytes that are adsorbed onto specially prepared nanometer-sized metal particles.1,2 The enhanced Raman signals in SERS are usually explained based on two separate mechanisms, namely, the electromagnetic (EM)3 effect and the chemical (CM)4–6 or charge-transfer effect. Both the effects (EM and CM) occur simultaneously and contribute towards the enhancement of the Raman signals. In the recent past, it has been shown that using spacers or by increasing the chemical bonding between the adsorbed molecule and metal nanoparticle (NP) surface it is possible to separate out the chemically enhanced modes from the electromagnetically enhanced modes in the SERS spectrum.7 The SERS technique has been widely exploited in identifying biomolecules, drugs and proteins as it provides the information about the interaction of the analytes with the metal surface i.e. the binding sites and the orientation of the adsorbates on the metal surfaces.8–22 SERS has also been exploited recently for studying surface-catalyzed oxidation23,24 and charge rearrangement reactions.25 Noble-metal NPs are ideal candidates for studying such interactions. Among the noble metal NPs, silver (Ag) and gold (Au) have received considerable attention due to their attractive physicochemical properties, namely; (i) their surface plasmon resonance (SPR) band lies in the visible region26 and (ii) their large effective scattering cross section make them ideal candidates for molecular labeling,27 where phenomena such as SERS can be exploited.
In this paper, we have carried out a detailed structural and conformational analysis to explore the different tautomeric forms of 2,5-dimercapto-1,3,4-thiadiazole (DMTD) in solid state, aqueous solution and its binding affinity towards Ag and Au NPs by Raman spectroscopy in combination with density functional theoretical (DFT) calculations. DMTD also known as Bismuth I and its derivatives have been extensively studied as chelating agents28–32 due to their unique structure that contains both nitrogen (N) and sulfur (S) atoms. DMTD has wide industrial applications and has been used as additives in the fields of antioxidation,33 anticorrosion,34,35 and antifriction,36–38 as metal deactivators39,40 in motor oils, and also as cross-linkers in polymers.41,42 They have also been widely used as surface-active agents although their surface chemistry is not very well understood43–45 and relatively few studies quantitatively characterize DMTD.46–58 DMTD has four potential electron donating sites that may be available for the adsorption of the molecule on the metal NP surface. It can coordinate as a bidentate ligand with (a) both the thiadiazole ring N atoms, (b) the ring S atom, (c) both the thiocarbonyl S atoms, or (d) one N atom and one S atom on either the same side or different sides of the molecule. Additionally, the π-electrons in the heterocyclic ring can also bind to the Ag and Au NP surfaces. The available literature data on the structure of DMTD is controvertial with the study of IR, UV and pKa measurements suggesting the thione–thiol tautomer to be the most prevalent59 whereas, the 15NMR study51 revealing equilibrium between the thione–thiol and the dithiol tautomers with the equilibrium strongly favouring the latter. The FT-IR and FT-Raman studies on DMTD indicated the dominance of the dithiol form in the solid state.60
Our main objective in this work was twofold (i) to identify the most prevalent conformations of DMTD in solid and in aqueous solution at different pH and (ii) to recognize the adsorbed species along with the binding site(s) that are involved using SERS in combination with DFT calculations (B3LYP functional with 6-311+G*, aug-cc-pvdz and LANL2DZ basis sets). From the SERS spectra, and DFT calculations of the different tautomeric forms of DMTD as well as their neutral Ag, Au, Ag4 and Au4 complexes, it has been inferred that in this study selective binding is observed i.e. different tautomeric forms of DMTD bind to Ag via the ring N atom whereas it binds to Au exclusively through the thiocarbonyl S atoms. At acidic and neutral pH, the dithiol and the thiol–thiolate tautomer of DMTD is exclusively chemisorbed over the Ag NP surface through the ring N atom, while on the Au NP surface at acidic medium, the dithiol and the thione–thiol tautomer of DMTD is adsorbed with slight contribution also from the dithione form. To the best of our knowledge, this is the first detailed report on the pH mediated vibrational analysis of DMTD in solution as well as DMTD functionalized Ag and Au NPs.
2. Materials and methods
2.1 Chemicals
2,5-Dimercapto-1,3,4-thiadiazole (DMTD), hydrogen tetrachloroaurate, silver nitrate (AgNO3), trisodium citrate and sodium borohydride, used for the preparation of colloidal Au and Ag NPs were from Aldrich chemicals, and S. D. fine chemicals, India. All the experiments were performed with Millipore water having resistivity of 18.2 MΩ cm at 25 °C.
2.2 Synthesis of silver and gold NPs
Colloidal Ag NPs were prepared by the chemical reduction of AgNO3 with NaBH4 using Creighton method.61 In brief, 10 mL AgNO3 (1 mM) solution was added drop wise to 30 mL of 2 mM ice-cold NaBH4 with slow stirring. The Ag NP formed was yellow in colour and was stable at room temperature (RT) for several weeks. The pH of the Ag NP was ∼9. Colloidal Au NPs were prepared by modified Turkevich method.62 In brief; 100 mL solution containing 0.01 g of HAuCl4·3H2O was brought to boil. 3 mL of 1% sodium citrate was then added to the boiling solution. Boiling was continued for another 15 min till the colour of the solution turned bright red. The solution was then allowed to cool down to RT and was further used for UV-vis absorption and Raman measurements. The pH of the Au NP was ∼6.5. These Ag and Au NPs were then used for further characterization and to carry out the SERS studies of DMTD.
2.3 Instrumental details
Bare and DMTD functionalized Ag as well as Au NPs were characterized using UV-vis absorption, transmission electron microscopic (TEM) and Raman techniques. The UV-vis absorption spectra were recorded using a Jasco V-650 spectrophotometer. For TEM measurements, the sample was prepared by adding one drop of sample solution onto a carbon-coated copper grid. The evaporation of the solvent leaves the NP on the surface and TEM measurements were carried out with JEM Model CM (200 kV), Philips. The Raman spectra of DMTD in solid state, aqueous solution and adsorbed over Ag NPs were recorded at RT using the 532 nm line, from a diode-pumped solid state (DPSS) Nd3+
:
YAG laser (Cobolt Samba 0532-01-0500-500) M/s Cobolt AB, Sweden. The SERS measurements of DMTD functionalized Au NPs were recorded using the 660 nm line from a DPSS laser (Ignis 660-500) M/s Laser Quantum Ltd. England. The laser power used to record the Raman spectrum was 25 mW and the spot size on the sample was ∼50 μm. For all the Raman and SERS measurements, the sample solution was taken in a standard 1 × 1 cm2 cuvette and the Raman scattered light was collected at 90° scattering geometry and detected using a charge-coupled device (CCD, Synapse, Horiba Jobin Yvon) based monochromator (Triax550, Horiba Jobin Yvon, France) together with edge filters for 532 and 664 nm, covering a spectral range of 200–1600 cm−1. The Raman spectrum of indene was used to calibrate the spectrometer, and the accuracy of the spectral measurement was estimated to be better than 1 cm−1.
3. Computational methods
In order to get an insight into the experimental results, the geometry optimization was performed for various tautomeric forms of DMTD, its mono-anionic forms, its di-anionic form and their neutral Ag, Au, Ag4 and Au4 complexes using DFT [Gaussian 03 program63] with B3LYP functional64 and 6-311+G* or aug-cc-pVDZ basis set for DMTD. For Ag and Au, LANL2DZ basis set was used. DMTD exists in three possible tautomeric forms, dithiol, thione–thiol and dithione as shown in Scheme 1. Geometry optimization was carried out for all the three tautomeric forms of DMTD. At the optimized geometry, the molecular vibrations were calculated and compared with the experimentally observed normal Raman spectra of solid and acidic solution. Geometry optimization was also performed for the thiol–thiolate, thione–thiolate and the dithiolate forms of DMTD (Scheme 1).
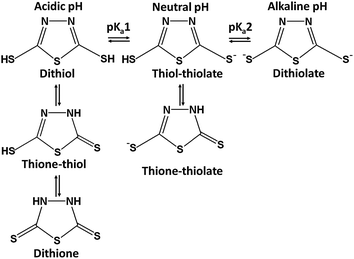 |
| Scheme 1 Scheme showing the proton dissociation equilibria of DMTD. | |
No symmetry restriction was applied during geometry optimization. The vibrational frequencies for the thiol–thiolate, thione–thiolate and dithiolate forms of DMTD were computed at the optimized geometry to ensure that they correspond to a local minimum on the potential energy surface and not to saddle points. Geometry optimization was also carried out for the various possible neutral Ag, Au, Ag4 and Au4 complexes of DMTD. The computed vibrations at the optimized geometry were compared with the experimentally observed SERS spectrum.
4. Result and discussions
4.1 UV-visible absorption study
The UV-visible electronic absorption spectra of metal NPs are related to their surface plasmon resonance (SPR) band,65 whose frequencies depend on the aggregation pattern of the NPs. The position and shape of the SPR band strongly depends on the size and shape of the NPs, the dielectric constant of the medium and the surface-adsorbed species. The UV-visible absorption spectra of bare Ag NPs and with varying concentrations of DMTD were monitored. As shown in Fig. 1(A), the electronic absorption spectrum of the bare Ag NPs (yellow) showed a single extinction maximum at 389 nm due to the SPR band. The absorption spectra of Ag NPs functionalized with DMTD at various concentrations (1, 5, 10, 50 and 100 μM) are shown in Fig. 1(A). It is observed that with the addition of 1 μM DMTD to the Ag NPs, the absorption spectrum shows a decrease in the absorbance at 389 nm. With an increase in the DMTD concentration (5, 10, 50 and 100 μM), the colour of the Ag NPs changed from yellow to dark brown due to adsorption of DMTD on the Ag NP surface and the red-shifted bands appeared at 432, 465, 481 and 501 nm, respectively. The appearance of the red-shifted bands are attributed to the aggregation of the Ag NPs in presence of the adsorbed molecule.66,67 The UV-visible electronic absorption spectra were also recorded for the bare Au NPs and DMTD (100 μM) functionalized Au NPs. In Fig. 1(B), is shown the electronic absorption spectrum of the stable Au NPs (bright red) with a single sharp peak at 519 nm due to the SPR band. Addition of 100 μM DMTD to the stable Au NPs resulted in the change in colour from bright red to purple along with a reduction in the absorbance at 519 nm and a broad peak appearing with maximum at 710 nm that is attributed to the aggregated Au NPs. The absorption spectrum of 100 μM aqueous solution of DMTD is also included in Fig. 1(B). A strong band with maximum at 328 nm (π–π* transition) is observed along with a weak broad peak appearing at 473 nm (n–π* transition).
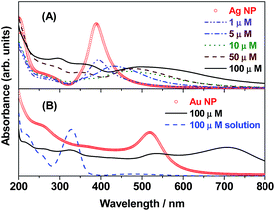 |
| Fig. 1 Surface plasmon resonance (SPR) bands of (A) Ag NPs and DMTD (1–100 μM) functionalized Ag NPs. (B) Au NPs, surface modified Au NPs with DMTD (100 μM) and 100 μM DMTD solution. | |
4.2 TEM measurements
In order to study the size and shape of the bare and DMTD functionalized Ag and Au NPs, TEM analysis was carried out. Ag NPs prepared by NaBH4 reduction method resulted in the formation of well-separated 15–20 nm particles as shown in Fig. 2(A). Addition of 100 μM DMTD to the Ag NPs resulted in aggregation of the particles. A representative TEM image of the aggregated particles is shown in Fig. 2(B). It is evident from the figure that addition of DMTD facilitates the aggregation of Ag NPs. The average size of the particles upon aggregation was 25 nm, although the particles retained their spherical shape. On the sides of Fig. 2(A) and (B) are shown the colour of the Ag NPs changing from yellow to dark brown upon the addition of 100 μM DMTD. Au NPs formed by sodium citrate reduction method resulted in the formation of well-separated 20–25 nm particles as shown in Fig. 2(C). Addition of 100 μM DMTD to the colloidal Au NPs leads to aggregation of the particles. The TEM image of the aggregated particles is shown in Fig. 2(D). The average size of the aggregated Au NPs was 35 nm with the particles retaining their spherical shape. On the sides of Fig. 2(C) and (D) are shown the colour of the Au NPs changing from dark red to purple upon aggregation.
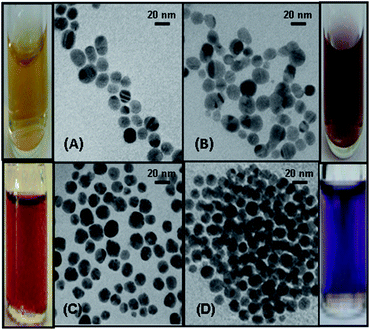 |
| Fig. 2 TEM image of (A) Ag NPs, (B) DMTD functionalized Ag NPs, (C) Au NPs and (D) DMTD functionalized Au NPs. On the sides of the figures are shown the colours of the bare and aggregated NPs. | |
4.3 Computational results
The dithiol, thione–thiol and dithione tautomers of DMTD, its mono-anionic and di-anionic forms were optimized using B3LYP functional with 6-311+G* and aug-cc-pvdz basis sets. The B3LYP/aug-cc-pvdz optimized structures of dithiol, thione–thiol and dithione forms of DMTD are shown in Fig. 3(A)–(C), respectively. In order to gain information regarding the relative stability of the different tautomers of the molecule in the gas phase, we have compared the minimum energies at their respective optimized geometries. The relative computed energies of the neutral tautomers at the B3LYP/aug-cc-pvdz level of theory indicate that the thione–thiol form of DMTD is lowest in energy and hence, the most stable tautomer. The dithione and dithiol forms are less stable in energy when compared to the thione–thiol tautomer by 5.49 and 8.48 kcal mol−1, respectively. The mono-anionic forms of DMTD viz. thiol–thiolate and thione–thiolate were optimized using B3LYP/aug-cc-pvdz and their optimized structures are shown in Fig. 3(D) and (E). The di-anionic tautomer of DMTD is the dithiolate and its optimized structure (B3LYP/aug-cc-pvdz) is shown in Fig. 3(F). The computed energies suggest that the thione–thiolate form is more stable than the thiol–thiolate tautomer by 14.94 kcal mol−1 and the dithiolate form has the highest energy and hence the least stable tautomer. The order of stability of the tautomeric forms of DMTD in the gas phase is as follows: thione–thiol (0 kcal mol−1) > dithione (5.49 kcal mol−1) > dithiol (8.48 kcal mol−1) > thione–thiolate (310.11 kcal mol−1) > thiol–thiolate (325.05 kcal mol−1) > dithiolate (724.22 kcal mol−1). The vibrations for all the tautomers of DMTD were computed and compared with the experimental Raman spectra.
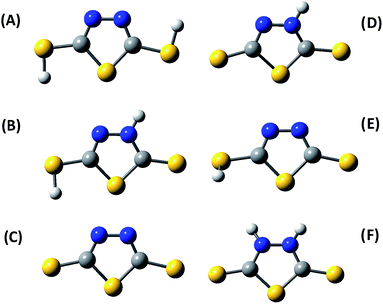 |
| Fig. 3 Optimized structures of (A) dithiol, (B) thione–thiol, (C) dithione, (D) thione–thiolate, (E) thiol–thiolate and (F) dithiolate forms. The colour codes used to identify the atoms are N (blue), S (yellow), C (grey) and H (white). | |
The acid dissociation constants of DMTD are known to be 1.4 and 7.4 (ref. 68) (2.1 and 7.5),69 making its neutral form (dithiol, thione–thiol and dithione) a very strong acid and its monoanionic form (thione–thiolate and thiol–thiolate) a weak acid. Hence, at an acidic pH 1.5, DMTD exists mainly in the neutral form as dithiol, thione–thiol or dithione whereas, at pH ≥ 8 it remains primarily as dithiolate. In the intermediate pH range i.e. 2 ≥ pH ≤ 8, DMTD exists mostly in the mono-anionic form, viz. thione–thiolate or thiol–thiolate. In this work, the pH of the aqueous solution was varied to identity the features arising due to the neutral, monoanionic or dianionic forms of DMTD. Similarly, the adsorption of the neutral (dithiol, thione–thiol, dithione), monoanionic (thione–thiolate and thiol–thiolate) or dianionic (dithiolate) forms of DMTD was monitored at pH 1.5 and pH 7 on the Ag and Au NP surfaces.
For the DMTD–Ag and DMTD–Au complexes, various conformers are possible. The dithiol and thione–thiol tautomers of DMTD can bind to Ag or Au through the ring N atoms, ring S atom, the thiocarbonyl S atom and the π electrons of the thiadiazole ring. Similarly, the dithione tautomer can bind to Ag or Au through the ring S atom, the thiocarbonyl S atom and the π electrons of the thiadiazole ring. B3LYP functional with aug-cc-pvdz (for DMTD) and LANL2DZ (for Ag and Au) basis sets were used for simulating the optimized structure of various Ag, Au, Ag4 and Au4 complexes of dithiol, thione–thiol and dithione. The optimized structures of the neutral dithiol–Ag, thione–thiol–Ag and dithione–Ag complexes are shown in Fig. S1(A–I) as ESI.† The computed Raman spectra of the various possible Ag and Ag4 complexes were compared with the experimental SERS spectrum at acidic pH. The molecular structures were also optimized for the monoanionic and dianionic DMTD–Ag and DMTD–Ag4 complexes i.e. thione–thiolate–Ag, thiol–thiolate–Ag, dithiolate–Ag, thione–thiolate–Ag4, thiol–thiolate–Ag4 and dithiolate–Ag4 complexes. The optimized structures of the thione–thiolate–Ag, thiol–thiolate–Ag and dithiolate–Ag complexes are shown in Fig. S2(A–I) as ESI.† The computed Raman spectrum of the monoanionic and dianionic DMTD–Ag as well as DMTD–Ag4 complexes were compared with the SERS spectrum of DMTD functionalized Ag NPs at pH 7. Similar to the Ag complexes of DMTD, various possible conformers for the DMTD–Au and DMTD–Au4 complexes were optimized using B3LYP with aug-cc-pvdz basis set for DMTD and LANL2DZ basis set for Au. The optimized structures for the Au complexes of the neutral tautomers (dithiol, thione–thiol and dithione) of DMTD are shown in Fig. S3(A–I) as ESI.† The vibrational frequencies were also calculated at the respective optimized geometries for the Au and Au4 complexes of DMTD and compared with the experimental SERS spectrum at acidic pH.
4.4 Raman spectra of DMTD and its vibrational assignments
The neutral tautomeric forms of DMTD, viz. dithiol, thione–thiol and dithione with 9 atoms have 21 fundamental modes of vibrations. Of these, the dithiol and dithione forms belong to the Cs point group and have 15 in-plane (A′) and 6 out-of-plane (A′′) fundamental vibrations. All these modes of vibrations are thus expected to appear both in Raman and infrared spectra. On the other hand, the thione–thiol form belongs to the C1 point group and all the fundamental vibrations are thus expected to appear both in Raman and infrared spectra. The Raman spectrum of DMTD in the solid state for the region 200–1600 cm−1 is shown in Fig. 4. All the observed Raman vibrations are tabulated in Table 1. The assignments of the Raman bands to the stretching and bending vibrations of the thiadiazole ring and the mercapto groups are based on comparison of the Raman spectrum of the solid with the calculated Raman vibrations of the dithiol, thione–thiol and dithione forms of DMTD as shown in Fig. 5(A). The Raman spectrum of solid DMTD exhibits two strong bands in the frequency region 200–1600 cm−1 [Fig. 4] at 1458 and 663 cm−1 and are assigned to C
N stretch (str) in combination with CSH bend and CSC endocyclic symmetric (sym) str or ring breathing mode. Medium intensity Raman bands are observed at 1518, 1483, 1398, 1288, 1107, 1039, 1021, 544, 500, 382, 329 and 215 cm−1. The 1518, 1483 and 1288 cm−1 vibrations are assigned to C
N str, C–N asymmetric (asym) str and C–N sym str, respectively. The bands at 1398 and 1107 cm−1 are attributed to ring C
N str and N–N str in combination with NH bend. The 1039 and 1021 cm−1 bands are assigned to N–N str and C–S str. The Raman bands at 544 (500), 382, 329 and 215 cm−1 are attributed to ring distortion in combination with NH out of plane (oop) bend, NH wagging, CSC sym bend and HNNH torsion, respectively. Weak bands are observed at 1080, 725, 405 and 259 cm−1. Of these vibrations, the modes observed at 1080 and 725 cm−1 correspond to N–N str and CNN bend while the bands at 405 and 259 cm−1 are attributed to NH twisting and ring rotation, respectively. A close examination of the solid Raman spectrum [Fig. 5(A)(a)] and comparing the same with the theoretically calculated (B3LYP/aug-cc-pVDZ) Raman spectra of all the tautomeric forms of DMTD viz. dithiol [Fig. 5(A)(b)], thione–thiol [Fig. 5(A)(c)] and dithione [Fig. 5(A)(d)] suggests that the solid spectrum is a combination of the theoretically calculated spectra of all three tautomeric forms of DMTD, viz. dithiol, thione–thiol and dithione. In addition, the presence of the medium intensity peak around 2499 cm−1 (shown in Fig. S4(a) as ESI†) attributed to S–H str in the Raman spectrum of solid indicates, the preferential existence of the dithiol or the thione–thiol form of DMTD in the solid state. Weak Raman band is observed around 3062 cm−1 indicating H-bonded N–H stretch [Fig. S4(a)†]. The simulated Raman spectrum [Fig. 5(A)(e)] with contributions from dithiol (44%), dithione (36%) and thione–thiol (20%) matches well with the solid Raman spectrum, thus, indicating the existence of all the three tautomers in the solid state.
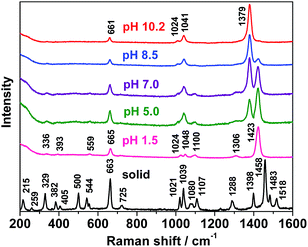 |
| Fig. 4 Raman spectra of solid DMTD and its 10−2 M solution at a range of pH. | |
Table 1 Assignments of Raman (solid) and B3LYP/aug-cc-pvdz calculated vibrations (in cm−1) for the dithiol, thione–thiol and dithione tautomeric forms of DMTDa
Raman |
Calculated vibrations |
Assignments |
Solid |
Dithiol |
Thione–thiol |
Dithione |
Abbreviations used: s: strong, m: medium, w: weak; asym: asymmetric, sym: symmetric, str: stretch, oop: out-of-plane. |
3062w |
|
|
|
N–H str |
2499m |
|
|
|
S–H str |
1518m |
|
1533 |
|
C N str, NH bend |
1483m |
|
1430 |
1458 |
C–N asym str, NH bend |
1458s |
1438 |
1483s |
|
C N str, CSH bend |
1398m |
1416 |
|
1408 |
C N str, N–N str, NH bend |
1288m |
|
|
1304 |
C–N sym str, NH bend |
1107m |
|
1123 |
|
N–N str, NH bend |
1080w |
1053 |
1056 |
|
N–N str, CSH bend |
1039m |
1090 |
|
1089 |
N–N str |
1021m |
|
|
1019 |
C–S str, NH bend |
725w |
699 |
685 |
|
CNN bend, CSH bend |
663s |
610 |
623 |
641 |
CSC endocyclic sym str/ring breathing |
544m |
487 |
521 |
532 |
Ring distortion, NH oop bend |
500m |
405w |
|
|
462 |
NH twisting |
382m |
|
|
439 |
NH wagging |
329m |
|
|
362 |
CSC sym bend |
259w |
|
|
314 |
Ring rotation |
215m |
|
|
251 |
HNNH torsion |
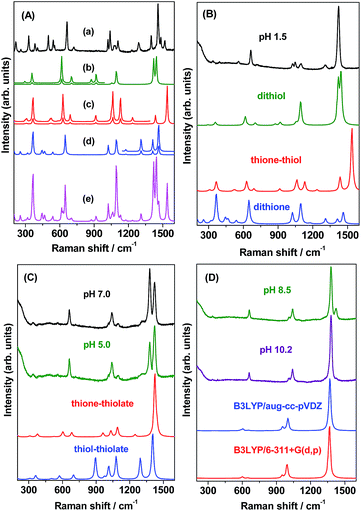 |
| Fig. 5 (A) (a) Raman spectrum of solid DMTD and calculated (B3LYP/aug-cc-pvdz) Raman spectra of (b) dithiol, (c) thione–thiol, (d) dithione and (e) sum of dithiol, thione–thiol and dithione. Raman spectrum of 10−2 M DMTD solution (B) at pH 1.5 and the calculated Raman spectra of dithiol, thione–thiol, dithione, (C) at pH 7.0 and pH 5.0 and the calculated Raman spectra of thione–thiolate and thiol–thiolate and (D) at pH 8.5 and pH 10.2 and the calculated Raman spectra [B3LYP/aug-cc-pvdz and B3LYP/6-311+G*] of dithiolate. | |
4.5 pH-dependent normal Raman spectra of DMTD in aqueous solution
The Raman spectra of 10−2 M aqueous solution of DMTD at different pH is shown in Fig. 4 for the 200–1600 cm−1 region. The Raman vibrations are tabulated in Table 2. As shown in Fig. 4, at pH 1.5 intense peak is observed at 1423 cm−1 followed by a medium intense band at 665 cm−1. Few weak Raman bands are also observed at 1306, 1100, 1048, 1024, 559, 393 and 336 cm−1. Of these modes, the 1423 cm−1 band is assigned to C
N stretch in combination with CSH bend. The vibration observed at 665 cm−1 is attributed to CSC endocyclic sym str. The weak Raman bands at 1306, 1100 (1048), 1024, 559, 393 and 336 cm−1 are assigned to C–N sym str, N–N str, C–S str, ring distortion, NH wagging and CSC sym bend, respectively. A close examination of the Raman spectrum at pH 1.5 [Fig. 5(B)] and comparing it with the calculated Raman spectra of the three tautomeric forms of DMTD viz. dithiol, thione–thiol and dithione [Fig. 5(B)] suggests that the solution spectrum has contributions from dithiol (48%), dithione (32%) and thione–thiol (20%). At pH 1.5, a weak band is observed at 2330 cm−1 assigned to the S–H str and is shown in Fig. S4(b) as ESI.† The N–H str vibration around 3000 cm−1 is not observed in the solution Raman spectrum at pH 1.5 as it is completely masked by the broad O–H str vibration (3600 cm−1) of water. The normal Raman spectrum of the 10−2 M DMTD solution at pH 5.0, shows the growth of a new band at 1379 cm−1 in addition to the increase in intensity of the 1048 cm−1 band. All the other vibrational features remain similar to the Raman spectrum of DMTD solution at pH 1.5. The 1379 cm−1 band is attributed to the C
N str in combination with N–N str and NH bend. The 1048 cm−1 band is assigned to the N–N str. The Raman spectrum of DMTD at pH 7.0 is almost similar to the spectrum at pH 5.0 with the only exception that the 1379 cm−1 band shows further growth and has enhanced intensity as compared to the 1423 cm−1 band. The Raman spectra at pH 5.0 and pH 7.0 were found to be comparable to the calculated Raman spectra of thione–thiolate and thiol–thiolate as shown in Fig. 5(C). The Raman spectrum of DMTD recorded at pH 8.5 shows sharp reduction in the intensities of the 1423 and 1100 cm−1 bands along with further growth of the 1379 cm−1 band with the latter having enhanced intensity as compared to the 1423 cm−1 band. Other features remain almost similar to the spectra at pH 5.0 and pH 7.0. From the figure it is clearly seen that around pH 5–8 DMTD solution exists as a combination of the tautomers, viz. thione–thiolate and thiol–thiolate. At pH 10.2, the Raman band at 1379 cm−1 is found to be very intense. In addition, two medium intense peaks are observed at 1041 and 661 cm−1 along with a weak band at 1024 cm−1. In Fig. 5(D), the Raman spectra recorded at pH 8.5 and 10.2 are shown along with the calculated Raman spectra [B3LYP/aug-cc-pvdz and B3LYP/6-311+G*] of the dithiolate tautomer of DMTD. The figure shows that the Raman spectrum of DMTD solution at pH 10.2 closely resembles the calculated Raman spectra of the dithiolate tautomer. Comparison of the Raman spectra in the entire pH range shows that the spectra is strikingly similar around pH 5–9 with slight variations in the intensities of the 1379 and the 1423 cm−1 peaks. At acidic pH 1.5, the 1423 cm−1 band is strong with complete disappearance of the 1379 cm−1 peak whereas, the opposite trend is observed at alkaline pH 10.2 (a strong band is observed at 1379 cm−1 with complete disappearance of the 1423 cm−1 band).
Table 2 Assignments of Raman (10−2 M solution) and SERS spectra of DMTD at varying pHa
Raman spectra (solution) |
SERS spectrum |
Assignments |
Ag NPs |
Au NPs |
pH 1.5 |
pH 5.0 |
pH 10.2 |
pH 1.5 |
pH 7.0 |
pH 1.5 |
Abbreviations used: s: strong, m: medium, w: weak; sym: symmetric, str: stretch, oop: out-of-plane. |
2330w |
|
|
|
|
|
S–H str |
1423s |
1423s |
|
1413vs |
|
1412s |
C N str, CSH bend |
|
1379s |
1379vs |
1358vs |
1362vs |
|
C N str, NN str, NH bend |
1306w |
|
|
|
|
1299w |
C–N sym str, NH bend |
1100w |
|
|
|
|
|
N–N str, NH bend |
1048w |
1042m |
1041m |
1062s |
1064s |
1058m |
N–N str |
1024w |
|
1024w |
|
|
1027m |
C–S str, NH bend |
|
|
|
|
|
720w |
CNN bend, CSH bend |
665m |
662m |
661m |
664m |
668s |
657s |
CSC endocyclic sym str/ring breathing |
559w |
|
|
546m |
542m |
542m |
Ring distortion, NH oop bend |
|
|
|
|
|
485m |
NH twisting |
393w |
|
|
382m |
384m |
385s |
NH wagging |
336w |
|
|
346w |
352w |
|
CSC sym bend |
4.6 Surfaced-enhanced Raman scattering (SERS) spectra of DMTD
The SERS spectra of DMTD at different concentrations (0.1 to 100 μM) on the Ag NP surface were monitored at pH 7.0 and are shown in Fig. 6(A). The SERS spectra show slight enhancement at 100, 50, 10, 5 and 0.1 μM concentrations of DMTD. Excellent SERS enhancement is observed at the concentrations of 1 and 0.5 μM DMTD, with maximum enhancement for the former, probably due to monolayer coverage. Intense SERS intensities were observed for the 1362 (C
N str), 1064 (N–N str), 668 (CSC endocyclic sym str) and 384 (NH wagging) cm−1 bands with medium and weak intensities for the 542 (ring distortion) and the 352 (CSC sym bend) cm−1 bands, respectively. From Fig. 6(A) it is observed that the change in concentration of DMTD, results only in the change in intensities of the Raman bands with absolutely no change in their peak positions. This indicates that the adsorbed DMTD molecule undergoes no change in the binding mechanism or orientation with change in concentration. In the pH range 5–8, DMTD mainly exists on the Ag NP surface as monothiolate ion i.e. as thione–thiolate or the thiol–thiolate tautomer. With the change in concentrations from 100 to 0.1 μM, the monothiolate ions of DMTD undergo no change in binding or orientation over the Ag NP surface.
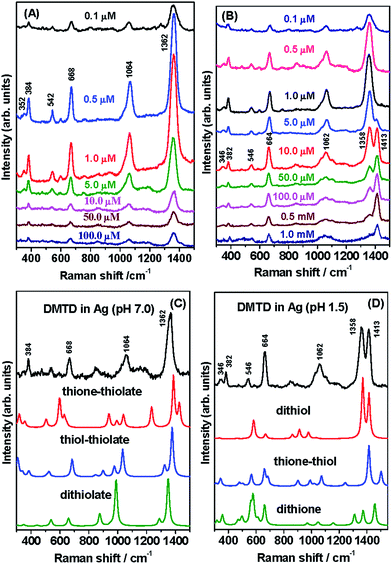 |
| Fig. 6 Concentration-dependent SERS spectra of DMTD functionalized Ag NPs at (A) pH 7.0 and (B) pH 1.5. Comparison of the SERS spectrum (C) at pH 7.0 with the calculated Raman spectra of thione–thiolate–Ag4, thiol–thiolate–Ag4 and dithiolate–Ag4 complexes and (D) at pH 1.5 with calculated Raman spectra of dithiol–Ag4, thione–thiol–Ag4 and dithione–Ag4 complexes. | |
The SERS spectra of DMTD functionalized Ag NPs were also recorded under acidic conditions for various concentrations (1 mM to 0.1 μM). The SERS spectra at pH 1.5 are shown in Fig. 6(B). The SERS spectra show slight enhancement at 1 mM, 0.5 mM, 100 μM, 50 μM and 0.1 μM concentrations of DMTD. Excellent enhancement is observed at the concentrations of 10, 5, 1, 0.5 μM DMTD, with maximum enhancement for 1 μM. DMTD probably forms a monolayer on the Ag NP surface at the concentration of 1 μM. At concentrations lower than 1 μM, there is sub-monolayer coverage over the Ag NP surface and for concentrations greater than 1 μM, multi-layers of the adsorbate are stacked over the NP surface and its behavior is similar to that in bulk solution. It is interesting to see that at minimum concentration (0.1 μM) of DMTD, the Raman bands are mainly observed at 1358, 1062, 664 as well as 382 cm−1 and are assigned to C
N stretch in combination with N–N str, N–N str, CSC endocyclic sym str and NH wagging, respectively. With increase in concentration of DMTD (0.5, 1 and 5 μM), the Raman bands at 1358, 1062, 664 and 382 cm−1 grow in intensities and new features also appear at 1413, 546 and 346 cm−1 and are assigned to C
N str, ring distortion and CSC sym bend. With further increase in concentration (10 μM, 50 μM, 100 μM, 0.5 mM and 1 mM) the 1413 cm−1 bands show enhancement with simultaneous decrease in intensity for the 1358 cm−1 band. The latter Raman band appears as a shoulder at highest (1 mM) concentration of DMTD. Thus, the concentration-dependent SERS spectra of DMTD show interesting structural changes at pH 1.5. This could possibly be due to the preferential existence of dithiol, thione–thiol and dithione forms of DMTD on the Ag NP surface at acidic pH. At low concentrations of DMTD, the enhancement of the 1358 cm−1 band (C
N stretch coupled to N–N str) indicates the favored existence of dithione form on the Ag NP surface at pH 1.5. At higher concentrations (5, 10, 50, 100 and 500 μM), there is a gradual increase in the intensity of the 1413 cm−1 band (C
N str) with simultaneous decrease in the 1358 cm−1 peak. Thus, increase in the concentration of DMTD, leads to the favourable existence of the dithiol form on the Ag NP surface although slight contribution from the thione–thiol form cannot be completely neglected. At the highest concentrations of DMTD (1 mM), the presence of the SERS bands at 1413 and 664 cm−1 confirm the abundance of the dithiol form on the Ag NP surface.
The SERS spectra of DMTD at neutral and acidic pH at the adsorbate concentration of 0.1 μM on the Ag NP surface are almost similar in the range 300–1300 cm−1. In the region 1300–1500 cm−1, the SERS spectra at pH 7.0 and pH 1.5 are strikingly different. At pH 7.0, a strong single band is observed at 1362 cm−1 with band width of 40 cm−1 whereas at pH 1.5, two Raman bands are observed with almost equal intensities at 1358 and 1413 cm−1. This difference is mainly due to the structural changes that exist in DMTD at pH 7.0 and pH 1.5. At pH 7.0, DMTD mostly exists as thione–thiolate and thiol–thiolate tautomers with little contribution from the dithiolate form. The presence of the latter (dithiolate form) may be due to the fact that the pKa values of the compounds containing mercapto groups are usually lowered on the NP surface. It is to be noted that increasing the pH to 10.2 does not facilitate the adsorption of the dithiolate form of DMTD on the Ag NP surface.
In order to understand the adsorption behavior of DMTD on the Ag NP surface at neutral pH 7.0, its SERS spectrum is compared with the calculated Raman spectrum of the mono-anionic forms of DMTD–Ag4 complex i.e. thione–thiolate–Ag4 and thiol–thiolate–Ag4 as shown in Fig. 6(C). In addition, the Raman spectrum for the dithiolate–Ag4 complex is also included in Fig. 6(C) for comparison. The optimized structures of the thione–thiolate–Ag4, thiol–thiolate–Ag4 and dithiolate–Ag4 complexes are shown in Fig. 7(A)–(C). It is observed from the Fig. 6(C) that the SERS spectrum matches reasonably well with the thiol–thiolate–Ag4 complex of DMTD indicating the abundance of this tautomeric form on the Ag NP surface bound exclusively through the ring N atom. Slight contributions from the thione–thiolate–Ag4 or the dithiolate–Ag4 complexes cannot be completely ignored.
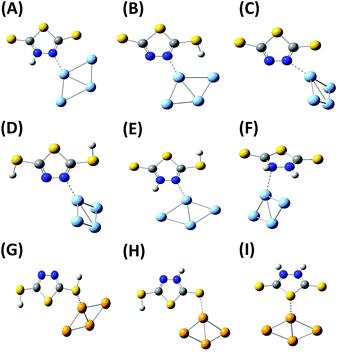 |
| Fig. 7 Optimized structures of (A) thione–thiolate–Ag4, (B) thiol–thiolate–Ag4, (C) dithiolate–Ag4 (D) dithiol–Ag4, (E) thione–thiol–Ag4, (F) dithione–Ag4, (G) dithiol–Au4, (H) thione–thiol–Au4, (I) dithione–Au4 complexes. The colour codes used to identify the atoms are N (blue), S (yellow), C (grey), H (white), Ag (light blue) and Au (orange). | |
Similarly, the binding affinity of DMTD on the Ag NP surface at acidic pH 1.5 was studied by comparing its SERS spectrum with the calculated Raman spectra of the neutral tautomeric forms of DMTD–Ag4 complex i.e. dithiol–Ag4, thione–thiol–Ag4 and dithione–Ag4 as shown in Fig. 6(D). The optimized structures of the dithiol–Ag4, thione–thiol–Ag4 and dithione–Ag4 complexes are shown in Fig. 7(D)–(F). The Fig. 6(D) clearly shows the resemblance of the dithiol–Ag4 Raman spectrum with the SERS spectrum at pH 1.5. This indicates the abundance of the dithiol form of DMTD on the Ag NP surface where the dithiol tautomer is bound to Ag via the thiadiazole ring N atom. The low frequency region in the SERS spectrum from 300–800 cm−1 matches reasonably well with the Raman spectrum of the thione–thiol–Ag4 complex suggesting the presence of the thione–thiol conformer also on the Ag NP surface that remains bonded to Ag via the ring N atom. Slight contribution from the dithione tautomer may also be present on the Ag NP surface.
In colloidal gold nanoparticles (GNPs) under acidic conditions (pH 1.5), the concentration-dependent (100 to 0.1 μM) SERS spectra of DMTD were recorded and are shown in Fig. 8(A). It is to be noted that the adsorption of DMTD on GNPs was observed only for acidic pH and not for neutral or alkaline pH. This is in contrast to the adsorption behavior of DMTD on the Ag NP surface which occurs at both acidic and neutral pH indicating higher binding affinity of DMTD towards Ag as compared to Au NPs. At pH 1.5, the SERS spectra [Fig. 8(A)] show poor signal/noise (S/N) ratio at DMTD concentrations of 0.1 and 0.5 μM. With increase in DMTD concentrations from 1 to 100 μM, good enhancement is observed in the overall SERS spectra. The SERS spectrum of 1 μM DMTD on GNPs show strong Raman bands at 1412, 657 and 385 cm−1 and are assigned to C
N str, CSC endocyclic sym str and NH wagging. In Fig. 8(A) moderate intensity Raman bands are observed at 1058, 1027, 542 and 485 cm−1 and are assigned to N–N str, C–S str, ring distortion and NH twisting. Weak Raman bands are also observed at 1299 and 720 cm−1 and are assigned to C–N sym str and CNN bend. At 1 μM DMTD, the SERS spectrum shows reduced intensity at 1412 cm−1; instead a broad feature is observed around 1355 cm−1 that is attributed to C
N stretch in combination with N–N str. For a comparative picture, the SERS spectrum of DMTD adsorbed on Au NP at acidic pH is plotted in Fig. 8(B) along with the theoretically calculated Raman spectra of the dithiol–Au4, thione–thiol–Au4 and dithione–Au4 complexes. The figure clearly shows that the SERS spectrum matches reasonably well with the calculated Raman spectrum of the dithiol–Au4 complex in the spectral range 1000–1550 cm−1 indicating the abundance of the dithiol tautomer on the Au NP surface. The 657 and 720 cm−1 region of the SERS spectrum is reproduced reasonably well by the calculated Raman spectra of the thione–thiol–Au4 and dithione–Au4 complexes. The low frequency 300–600 cm−1 region matches quite well with the calculated Raman spectrum of dithione–Ag4 complex. This indicates the presence of all the three tautomers, viz. dithiol, thione–thiol and dithione of DMTD on the Au NP surface.
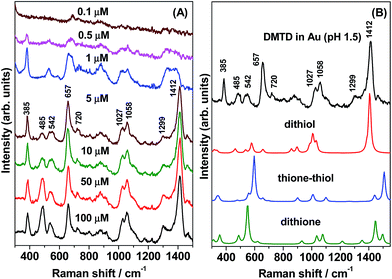 |
| Fig. 8 Concentration-dependent SERS spectra of DMTD functionalized Au NPs (A) at pH 1.5 and (B) comparison of the SERS spectrum at pH 1.5 with the calculated Raman spectra of dithiol–Au4, thione–thiol–Au4 and dithione–Au4 complexes. | |
Comparing the SERS spectrum of DMTD functionalized Au NPs at acidic pH with the SERS spectrum of DMTD functionalized Ag NPs, the main difference observed is primarily due to the presence of a single band at 1412 cm−1 in the case of the former; instead a doublet appears at 1358 and 1413 cm−1 in case of the latter. The main difference in the SERS spectrum arises from their binding behavior i.e. the dithiol, thione–thiol and dithione tautomers bind to Au NPs via the thiocarbonyl or the thiadiazole ring S atom where as in the case of Ag NPs, these conformers are bound via the ring N atom exclusively.
The SERS spectra combined with the DFT results thus, clearly indicate the existence of the thiol–thiolate tautomer of DMTD on the Ag NP surface at neutral pH that is bound to the metal via the thiadiazole ring N atom. At acidic pH, on the Ag NP surface, the existence of the dithiol and the thione–thiol forms are clearly indicated with little contribution from the dithione form. Thus, at acidic pH, the dithiol, thione–thiol and dithione forms of DMTD are bound to the Ag via the ring N atom. In contrast, at acidic pH on the Au NP surface, all the three tautomers of DMTD (dithiol, thione–thiol and dithione) are present on the surface with abundance of the dithiol form and are bound to the metal through the thiocarbonyl or the thiadiazole ring S atom. In this study, SERS in combination with DFT studies clearly indicated the presence of the respective tautomeric forms available on the metal surface at particular pH (neutral or acidic) and their binding affinity.
5. Conclusions
In this article, detailed structural and conformational investigation of DMTD is reported in solid, aqueous solution at different pH and on Ag and Au NP surfaces. The Raman spectrum of solid DMTD is interpreted with the help of DFT calculations with B3LYP functional and aug-cc-pvdz basis set that shows the presence of the dithiol, thione–thiol and the dithione tautomers in the solid state. The pH-dependent Raman study of DMTD solution suggests major contribution from the dithiol conformer at acidic pH. In the neutral pH range (5–8), the existence of the thione–thiolate and thiol–thiolate forms are indicated. At alkaline pH, the dithiolate form of DMTD exists in solution. At acidic pH, the concentration-dependent SERS spectra of DMTD on Ag NPs indicated the preferential existence of the dithione form at lower concentrations of DMTD while the dithiol tautomer was predominant at higher concentrations. The SERS spectra at acidic and neutral pH clearly showed the abundance of the dithiol and the thiol–thiolate tautomers of the DMTD, respectively on the Ag NP surface selectively bound through the thiadiazole ring N atom. In case of DMTD functionalized Au NPs, the dithiol form is exclusively bound to Au selectively via the thiocarbonyl S atom. This study clearly indicated the selective binding affinity of DMTD on the Ag and Au NP surfaces via the ring N atom and the thiocarbonyl or the thiadiazole ring S atom. This selective binding affinity of DMTD can be further exploited in designing novel supramolecular nanostructures.
Acknowledgements
The authors thank Prof. K. Maiti of TIFR, Mumbai for the Gaussian 03W calculations.
Notes and references
- Surface Enhanced Raman Scattering, R. K. Chang and T. E. Furtak, Plenum Press, New York, 1982 Search PubMed
. - M. Moskovits, Rev. Mod. Phys., 1985, 57, 783 CrossRef CAS
. - J. P. Camden, J. A. Dieringer, J. Zhao and R. P. Van Duyne, Acc. Chem. Res., 2008, 41, 1653 CrossRef CAS PubMed
. - A. Campion and P. Kambhampati, Chem. Soc. Rev., 1998, 27, 241 RSC
. - J. R. Lombardi and R. L. Birke, Acc. Chem. Res., 2009, 42, 734 CrossRef CAS PubMed
. - A. Otto, J. Raman Spectrosc., 2005, 36, 497 CrossRef CAS
. - M. Futamata and Y. Maruyama, Appl. Phys. B: Lasers Opt., 2008, 93, 117 CrossRef CAS
. - M. Fleischmann, P. J. Hendra and A. McQuillan, Chem. Phys. Lett., 1974, 26, 163 CrossRef CAS
. - K. Kneipp, H. Kneipp, I. Itzkan, R. R. Dasari and M. S. Field, Chem. Rev., 1999, 99, 2957 CrossRef CAS PubMed
. - T. M. Cotton, in Spectroscopy of Surfaces, Wiley, New York, 1988, ch. 3 Search PubMed
. - B. Pettinger, Adsorption of Molecules at Metal Electrodes, VCH Publishers, New York, 1992, ch. 6 Search PubMed
. - M. Bolboaca, T. Iliescu, Cs. Paizs, F. D. Irimie and W. Kiefer, J. Phys. Chem. A, 2003, 107, 1811 CrossRef CAS
. - B. Pergolese, M. M. Miranda and A. Bigotto, J. Phys. Chem. B, 2004, 108, 5698 CrossRef CAS
. - N. Maiti, R. Chadha, A. Das and S. Kapoor, Spectrochim. Acta, Part A, 2015, 149, 949 CrossRef CAS PubMed
. - S. SenGupta, N. Maiti, R. Chadha and S. Kapoor, Chem. Phys. Lett., 2015, 639, 1 CrossRef CAS
. - R. Chadha, N. Maiti and S. Kapoor, J. Mol. Struct., 2014, 1076, 35 CrossRef CAS
. - N. Maiti, S. Thomas, J. A. Jacob, R. Chadha, T. Mukherjee and S. Kapoor, J. Colloid Interface Sci., 2012, 380, 141 CrossRef CAS PubMed
. - S. Thomas, N. Maiti, T. Mukherjee and S. Kapoor, Spectrochim. Acta, Part A, 2013, 112, 27 CrossRef CAS PubMed
. - S. Thomas, N. Biswas, V. V. Malkar, T. Mukherjee and S. Kapoor, Chem. Phys. Lett., 2010, 491, 59 CrossRef CAS
. - N. Biswas, S. Thomas, A. Sarkar, T. Mukherjee and S. Kapoor, J. Phys. Chem. C, 2009, 113, 7091 CrossRef CAS
. - N. Biswas, S. Thomas, S. Kapoor, A. Mishra, S. Wategaonkar and T. Mukherjee, J. Chem. Phys., 2008, 129, 184702 CrossRef PubMed
. - N. Biswas, S. Kapoor, H. S. Mahal and T. Mukherjee, Chem. Phys. Lett., 2007, 444, 338 CrossRef CAS
. - Y. F. Huang, M. Zhang, L. B. Zhao, J. M. Feng, D. Y. Win, B. Ren and Z. Q. Tian, Angew. Chem., Int. Ed., 2014, 53, 2353 CrossRef CAS PubMed
. - R. Chadha, N. Maiti and S. Kapoor, J. Phys. Chem. C, 2014, 118, 26227 CrossRef CAS
. - A. C. Dhayagude, N. Maiti, A. K. Debnath, S. S. Joshi and S. Kapoor, RSC Adv., 2016, 17405–17414 RSC
. - R. L. Garrell, Anal. Chem., 1989, 61, 401A CrossRef CAS
. - S. Schultz, D. R. Smith, J. J. Mock and D. A. Schultz, Proceedings of the National Academy of Sciences of the United States of America FIELD Publication FIELD Reference Number: FIELD Journal Code:7505876, 2000, vol. 97, p. 996 Search PubMed
. - G. D. Thorn, Can. J. Chem., 1960, 38, 1439 CrossRef CAS
. - P. Ray and J. Gupta, J. Indian Chem. Soc., 1934, 11, 403 Search PubMed
. - P. Ray and J. Gupta, J. Indian Chem. Soc., 1935, 12, 308 CAS
. - S. A. A. Zaidi and D. K. Varshney, J. Inorg. Nucl. Chem., 1975, 37, 1806 CrossRef CAS
. - K. S. Siddiqi, V. Islam, P. Khan, F. R. Zaidi, Z. A. Siddiqi and S. A. A. Zaidi, Synth. React. Inorg. Met.-Org. Chem., 1980, 10, 41 CrossRef CAS
. - W. M. Denton and S. A. M. Thompson, Inst. Pet. Rev., 1966, 20, 46 CAS
. - R. Q. Little Jr, J. Am. Chem. Soc., 1965, 10, D137 Search PubMed
. - R. Q. Little Jr, U.S. Patent 3,087,932 (cl. 260-302), Apr 30, 1963
. - E. K. Fields, Ind. Eng. Chem., 1957, 49, 1261 CrossRef
. - A. Bhattacharya, T. Singh, V. K. Verma and N. Prasad, Tribol. Int., 1995, 28, 189 CrossRef CAS
. - T. Ren and Q. J. Xue, Wear, 1994, 59, 172 Search PubMed
. - J. C. Zhang, Y. H. Luo and B. Y. Zhang, Acta Pet. Sin., 1997, 131 Search PubMed
. - P. C. Hamblin, D. Chasan and U. Kristen, 5th International Colloquium Additives for Lubricant Operational Fluids, Basel, Switzerland, 1986, J. Tech. Akad. Esslingen, Ostfilden, Germany, 1986 Search PubMed
. - F. O. Robert, Rubber India, 1997, 49, 19 Search PubMed
. - E. E. Lawson, H. G. M. Edwards and A. F. Johnson, Spectrochim. Acta, Part A, 1997, 53, 2571 CrossRef
. - J. J. Kester, T. E. Furtak and A. J. Becolo, J. Electrochem. Soc., 1982, 129, 1716 CrossRef CAS
. - D. Thierry and C. Leygraf, J. Electrochem. Soc., 1986, 133, 2236 CrossRef CAS
. - T. Hashemi and C. A. Hogarth, Electrochim. Acta, 1988, 33, 1123 CrossRef CAS
. - L. Huang, F. Tang, B. Hu, J. Shen, T. Yu and Q. Meng, J. Phys. Chem. B, 2001, 105, 7984 CrossRef CAS
. - T. Tatsuma, Y. Yokoyama, D. A. Buttry and N. Oyama, J. Phys. Chem. B, 1997, 101, 7556 CrossRef CAS
. - J. W. Bats, Acta Crystallogr., 1976, 32, 2866 CrossRef
. - V. T. Joy and T. K. K. Srinivasan, J. Raman Spectrosc., 2001, 32, 785 CrossRef CAS
. - E. E. Lawson, H. G. M. Edwards and A. F. Johnson, J. Raman Spectrosc., 1995, 26, 617 CrossRef CAS
. - Comprehensive Heterocyclic Chemistry: the Structure, Reactions, Synthesis, and Use of Heterocyclic Compounds, ed. A. R. Katritzki and C. W. Rees, Pergamon Press, Oxford, 1984 Search PubMed
. - S. Millefiori and A. Millifiori, J. Mol. Struct., 1987, 151, 373 CrossRef
. - H. G. M. Edwards, A. F. Johnson and E. E. Lawson, J. Mol. Struct., 1995, 351, 51 CrossRef CAS
. - H. Ling, S. Jian, R. Jun, M. Qingjin and Y. U. Tsing, Chin. Sci. Bull., 2001, 46, 1 CrossRef
. - E. Shouji, Y. Yokoyama, J. M. Pope, N. Oyama and D. A. Buttry, J. Phys. Chem. B, 1997, 101, 2861 CrossRef CAS
. - L. Huang, F. Tang, B. Hu, J. Shen, T. Yu and Q. Meng, J. Phys. Chem. B, 2001, 105, 7984 CrossRef CAS
. - L. Huanga, J. Shena, C. Yua, Q. Mengb and T. Yu, Vib. Spectrosc., 2001, 25, 1 CrossRef
. - P. Kalimuthu, P. Kalimuthu and S. Abraham John, J. Phys. Chem. C, 2009, 113, 10176 CrossRef CAS
. - S. Millefiori and A. Millifiori, J. Mol. Struct., 1987, 151, 373 CrossRef
. - H. G. M. Edwards, A. F. Johnson and E. E. Lawson, J. Mol. Struct., 1995, 351, 51 CrossRef CAS
. - J. A. Creighton, C. G. Blatchford and M. G. Albrecht, J. Chem. Soc., Faraday Trans. 2, 1979, 75, 790 RSC
. - J. Kimling, M. Maier, B. Okenve, V. Kotaidis, H. Ballot and A. Plech, J. Phys. Chem. B, 2006, 110, 15700 CrossRef CAS PubMed
. - M. J. Frisch, G. W. Trucks, H. B. Schlegel, G. E. Scuseria, M. A. Robb, J. R. Cheeseman, J. A. Montgomery Jr, T. Vreven, K. N. Kudin and J. C. Burant, et al., Gaussian 03, Revision C.02, Gaussian Inc., Wallingford CT, 2004 Search PubMed
. - A. D. Becke, J. Chem. Phys., 1993, 98, 1372 CrossRef CAS
. - Surface Enhanced Raman Scattering, ed. J. A. Creighton, R. K. Chang and T. E. Furtak, Plenum Press, New York, 1982, p. 315 Search PubMed
. - E. J. Liang, C. Engert and W. Kiefer, Vib. Spectrosc., 1993, 6, 79 CrossRef CAS
. - S. Sanchez-Cortes, J. V. Garcia-Ramos, G. Morcillo and A. Tinti, J. Colloid Interface Sci., 1995, 175, 358 CrossRef CAS
. - Y. Kiya, G. R. Hutchison, J. C. Henderson, T. Sarukawa, O. Hatozaki, N. Oyama and H. D. Abruna, Langmuir, 2006, 22, 10554 CrossRef CAS PubMed
. - S. Picart and E. Enies, J. Electroanal. Chem., 1996, 408, 53 CrossRef
.
Footnote |
† Electronic supplementary information (ESI) available: The optimized structures of all possible Ag and Au complexes of DMTD and the Raman spectra of solid DMTD and its solution at pH 1.5 from 2000–3800 cm−1 region. See DOI: 10.1039/c6ra10404e |
|
This journal is © The Royal Society of Chemistry 2016 |
Click here to see how this site uses Cookies. View our privacy policy here.