DOI:
10.1039/C6RA19989E
(Paper)
RSC Adv., 2016,
6, 96138-96146
Leukemia stem cells, direct targeting of CD123 based on the nano-smart polymer PMBN
Received
7th August 2016
, Accepted 26th September 2016
First published on 28th September 2016
Abstract
Usually, conventional chemotherapy of leukemia is not successful, as hematopoietic stem cells change to cancer type. So, it seems designing a new targeting system is necessary to overcome this problem. A nano smart polymer, PMBN conjugated with interleukin 3 as a ligand of the CD123 receptor, which is overexpressed on leukemia stem cells, was used with incorporated paclitaxel or ganoderic acid A, a fungal metabolite, to directly target and treat stem cell cancer. The rate of conjugation was about 75% and rate of loading by PTX and GA-A were about 88% and 77%, respectively. Toxicity and apoptosis analyses show that the nano carrier is more effective than free agents or non-targeted polymers which increase anti-proliferation of cells. The nano targeted polymer has no significant toxicity towards cells that do not express CD123. Generally, the PMBN-IL3-GA conjugate could be an interesting substitution for conventional therapy methods based on chemotherapy agents.
Introduction
Expression of CD123 or α chain of interleukin 3 receptor (IL-3Rα) has been observed in many different blood cell diseases. Jordan et al.1 and Testa et al.2 have discovered high expression of CD123 in acute myeloid leukemia, AML. Also, Muñoz et al.3 have revealed the expression of this receptor in acute lymphoblastic leukemia, type B, B-ALL illness. Conversely, some investigations have shown no expression of CD123 in T-ALL disease.2,3 In some disorders such as CLL, chronic lymphocytic leukemia, and hairy cell leukemia, HCL, the influence of IL-3Rα expression on a cell surface is not as much as in other ones, but it is still interesting.3 So, this biomarker can be used as a valuable target in different applications such as diagnosis, treatment and evaluation of diseases. By the way, an interesting issue is overexpression of CD123 by leukemia stem cells, LSCs, whereas in normal or hematopoietic stem cells, HSCs, little expression of this marker has been found.1 Traditional treatments such as chemotherapy or radiotherapy are not very successful for AML. Researchers found that AML leukemia stem cells (AML LSCs) can be responsible for drug resistance and recurrence of AML illness. Resistance of AML to cell cycle-specific cytotoxic agents,4 toxins that are responsible for the expression of ATP-associated transporters,5 and apoptotic stimulator drugs6 could be due to LSCs. By targeting LSCs very selectively, AML disease could be cured completely without any relapse. In conclusion, targeting of CD123, which is overexpressed on the surface of leukemia cells, by natural ligand IL-3, and incorporation with effective toxins and drugs, would be a “magic bullet” for treatment, as Liu et al. have proposed.7 In research by Li et al.,8 trying to treat the AML cells by targeting CD123 based on adenoviruses was successful and satisfying. In the present study, a new nano carrier was designed that would be able to target the AML LSCs based on CD123 receptors.
Recently, there has been much interest in biopolymers and biocompatible polymers, because of their great specificity. One of the most important parameters is their ability to be used in bio-applications such as drug delivery systems (DDSs) and biosensors. Interestingly, their side effects can be decreased or even eliminated.9–13 Also, the problem of rejection of artificial organs or scaffolds could be reduced more by utilizing them.14–16 The 2-methacryloyloxyethyl phosphorylcholine (MPC) polymer has the same polar group (phosphorylcholine or PC group) as biomembranes and possesses excellent biocompatibility, i.e., it lacks protein adsorption and platelet adhesion, is soluble in liquid phases, is stable at a wide range of pH and does not show any immune reaction.17–19 MPC polymers have been utilized as surface modifiers in many medical devices in order to improve their biocompatibility.20–22 Interestingly, the PMB polymer, poly (MPC-BMA), has n-butyl methacrylate (BMA) monomer which is extremely hydrophilic and it can be dissolved in water.23,24 MPC polymers with hydrophobic monomer units can solubilize hydrophobic drugs or even be used for gene therapy, for instance, PLCG/PMB,25 PMBN,26 poly(MPC-block-DEA),27 poly(DMAPAA-co-MPC-co-SA),28 and poly(MPC-co-DAMA).29
In a review article by Goda et al.,30 the roles and specifications of MPC polymers, which have PC groups, were described very carefully. Having regard to the plasma membranes of eukaryotes, which have PC groups, MPC polymers were synthesized to present bio-inert surfaces due to bipolar PC groups in their structure. Also, these molecules could be easily made into other polymers with a variety of useful and functional properties. The most important property of the PMBN polymer, amphiphilic ter-polymer poly[2-methacryloyloxyethyl phosphoryl choline (MPC)-co-n-butyl methacrylate (BMA)-co-p-nitrophenyloxycarbonyl poly(ethylene glycol-methacrylate) (MEONP)], is solubility in water. The MEONP monomer is responsible for conjugation of the polymer to biomolecules with free –NH2 groups, due to the active ester group of the monomer. This ability makes the polymer a suitable carrier to use for targeting in drug delivery systems and nano-biosensors. In addition, this amphiphilic polymer could directly penetrate the cell membrane of cells of interest without any toxicity.30 The reason for this phenomenon is that when the PMBN polymer is close to the cell membrane and linked to it by the linker, the MPC unit will bind to the hydrophilic side of the plasma membrane, and the hydrophobic block of the polymer, the BMA monomer, will fuse to the hydrophobic core of the cell membrane to escape from the aqueous environment. Consequently, the polymer enters the cell directly without energy consumption. This phenomenon is based on the amphiphilic nature of the polymer. This capability is individual, since almost all synthetic polymers are unable to cross the plasma membrane, which acts as a “bio-dam”, without destroying peptide layers or changing the well-defined protection biosystem of membranes.
For designing a new nano carrier, two different agents were utilized individually. The first one is ganoderic acid A, GA-A, a fungal metabolite of Ganoderma lucidum that has been used as a supplement in Japan and China for centuries. Actually, this fungal metabolite is a kind of triterpenoid with more than 130 different types. It has been extracted from Ganoderma and its activity as anti-tumor and anti-cancer agent has been discussed in some research reports.31–33 Calviño et al. discovered the ability of ganoderic acids to induce apoptosis in human blood cell cancers.34 In another investigation, anti-proliferation properties of these metabolites were studied on 26 different sensitive blood cell lines, like HL-60 and Blin-1; the results indicated apoptosis induction to 92%.35 The second agent, paclitaxel or PTX, is a conventional chemotherapy agent for treatment of different cancers and can be used for comparison of toxicity results. Both ganoderic acids and PTX are highly hydrophobic but are soluble in ethanol.
In this work, targeting of LSCs by conjugation of PMBN with interleukin-3, IL-3, which is a ligand of the CD123 receptor, was studied for the first time, we believe. In addition, PTX and GA-A were incorporated with the conjugated polymer to enhance their water solubility. The loading of GA-A on PMBN polymer was performed, for the first time to our knowledge. So, if the nano smart carrier PMBN-IL3-GA is effective for treatment of AML LSCs, a new route of therapy will be introduced without utilizing chemotherapy agents.
Experimental
Materials
Interleukin 3, PTX, RPMI 1640 cell culture, fetal bovine serum (FBS), MTT kit and ethanol were sourced from Sigma-Aldrich Co., Germany. Also, an Amicon filter device was obtained from Merck Milipore Co., USA. Ganoderic acid A was purchased from BOC Science Co., USA, and the Annexin V kit from Pharmingen Inc., USA. Kg-1 and Molt 4 cell lines were obtained from the cell line bank of the Pasteur Institute, Iran.
Methods
Preparation of PMBN conjugated with IL-3. PMBN was prepared by P. Motamed Fath, University of Tehran, with a composition of 40 mol% MPC, 50 mol% BMA and 10 mol% MEONP, and a molecular weight of about 52
000 daltons. Then the conjugate was prepared by dissolving 2 mg PBMN in 2 mL PBS (pH = 7.8) a day before 30 μL of IL-3 was added to solution. Then, after mixing gently for 48 h at 4 °C, an Amicon Ultra centrifugal filter device with cutoff 30
000 was used at 5000g for 13 min at 4 °C, to purify the PMBN-IL3 conjugate and remove free IL-3 and p-nitrophenol. For checking the efficiency of purification, the filtered solution was analyzed at 270 and 404 nm by spectrophotometer (Nanodrop 2000C, Thermo Scientific Co., USA), these wavelengths being specific to free IL-3 and p-nitrophenol. Three different solutions were prepared to measure efficiency of conjugation. Solution (a) comprised 1 mg PMBN in 1 mL NaOH 0.2 mol mL−1, solution (b) 1 mg PMBN in distilled water, and solution (c) 1 mg of PMBN-IL3 conjugate in 1 mL distilled water. The absorbance of each solution was measured at 404 nm.
Incorporation of PTX and GA-A into the conjugated polymer. The desired concentrations of PTX and GA-A were dissolved in ethanol, and then were added to the PMBN-IL3 conjugate in PBS solution for 90 min at room temperature. Ethanol was removed by evaporation to obtain PMBN-IL3 combined with each agent. For analysing efficiency of loading, each concentration of PTX and GA-A was measured by spectrophotometry at 227 and 255 nm before incorporation and after filtration by the Amicon filter device, as in the previous procedure. The difference was taken as the amount incorporated.
Cell lines and cytotoxicity. Kg-1 and Molt 4 were incubated in RPMI 1640 with 10% FBS in 96-well plates under standard conditions to reach 105 cells per well. KG-1 is an AML cell line that overexpresses CD123 marker on its cell membrane, and Molt 4 is an ALL cell line without expression of CD123. Different concentrations of PTX, GA-A, PMBN, PMBN-IL3, PMBN-PTX, PMBN-GA, PMBN-IL3-PTX, and PMBN-IL3-GA were added to wells under physiological conditions after incubation overnight at 37 °C. The cytotoxicity was assessed using 3-(4,5-dimethylthiazol-2-yl)-2,5-diphenyl tetrazolium bromide (MTT) assay. After the agents were added, cells were washed with fresh PBS, and fresh MTT and sodium succinate were added to dissolve the MTT formazan. Absorbance of each well was measured by microplate reader (Epoch, Biotek Co., USA) at 570 nm as a test wavelength and 630 nm as a reference wavelength.
Apoptosis and necrosis analysis. Induction of apoptosis and necrosis by PMBN, PMBN-IL, PMBN-IL3-PTX, PMN-IL3-GA, free PTX, and free GA-A in positive and negative cell lines was examined under physiological conditions by Annexin V kit using a flow cytometry instrument (FACSAria™, BD Co., USA). In apoptosis, phosphatidylserine (PS) which was in the inner plasma membrane came to the outside. To distinguish apoptosis from necrosis, cells were stained with FITC-annexin V and propidium iodide (PI), according to protocol. The first dye combines with external PS, and the second one binds to cellular DNA in necrosis and the early stage of apoptosis.
Statistical analysis. All data were analyzed by Mann–Whitney U test. Data were considered significant at P < 0.05. All tests and experiments were performed in triplicate and repeated at last three independent times.
Results and discussion
PMBN conjugation to IL-3 and incorporation of PTX and GA-A
The absorbance analysis of the Amicon-filtered PMBN-IL3 conjugate solution at 270 and 404 nm, wavelengths specific for IL-3 and p-nitrophenol, respectively, showed that the filtration was absolute and neither compound was present in the filtered solution. To determine the efficiency of conjugation of PMBN polymer with IL-3, three different solutions were prepared and analyzed by spectrophotometry. PMBN in NaOH solution reacted with all ester groups of the MEONP monomer, releasing p-nitrophenol. The absorbance was more than 2, so this peak was considered as the reference absorption. Although PMBN in distilled water released p-nitrophenol by hydrolysis, the amount released was not as much as for the previous solution. Finally, PMBN-IL3 conjugate in distilled water released residual p-nitrophenol groups, which indicated the number of ester groups that had not combined with IL-3. According to the following simple equation, the efficiency of conjugation was about 75%, which is a great achievement for the preparation of a targeting nano carrier (Scheme 1).
 |
| Scheme 1 Efficiency determination of PMBN-IL3 conjugate by using absorbance values Aa, Ab, and Ac at wavelength 404 nm (spectrum of p-nitrophenol), where Aa is the absorption of PMBN in water, Ab is absorption of PMBN-IL3 in water and Ac is the absorption of PMBN in NaOH. | |
To measure the amount of PMBN-IL3 incorporating PTX and GA-A, their absorbance was analyzed before and after loading. According to the results, the loading rates were 86.6% and 77% for PTX and GA-A, respectively (data not shown). Very interestingly, considering that these agents are hydrophobic and their solubility in water is less than 1%, these fractions of incorporation indicated PMBN could solubilize hydrophobic drugs.
Characteristics of synthesized nano smart carrier
According to DLS,† SEM,‡ and TEM§ analysis, the size of the PMBN polymer was about 40 nm, with a rod-shaped structure, which makes it a good choice for drug delivery systems. The conjugated and drug-loaded polymer were analyzed by field emission scanning electron microscopy (FESEM)¶ and differential light scattering (DLS), which confirmed the size of both nano systems to be about 150 ± 30 nm (data not shown).
Inhibition of AML cell line proliferation by PMBN-IL conjugates
Toxicity and anti-proliferation activities of PMBN-IL-PTX and PMBN-GA were measured on the Kg-1 cell line which overexpressed CD123 (positive control), and the Molt 4 cell line as a no-CD123 expression cell line (negative control). As shown in Fig. 1 and 2 and Table 1, targeting of nano carrier with IL-3 led to higher toxicity than the conventional PTX. The half-maximal inhibitory concentration (IC50) decreased to about 70% after 24 hour treatment, and to 56.5% after 48 hour treatment. Interestingly, the IC50 of the designed carrier was less in the positive control cell line than in the negative control. The incorporation of agent into PMBN was also analyzed by previous researchers.36,37 On the other hand, by comparing IC50 of PMBN-IL-PTX with PMBN-PTX, there was no toxicity increase of PMBN-PTX carrier compared with the nano smart carrier, around 20%, but the difference between PMBN-IL-PTX and PMBN-PT was 61.3% and 46.1% at 24 and 48 h of treatment, respectively (Table 1). These results confirmed the importance of targeting by IL-3, and indicated targeting of drug delivery to the cells was successful. Therefore, two factors are significant for increasing the anti-proliferation activity of PMBN-IL3 with incorporated PTX, (1) the effect of agent loading by solubility enhancement of hydrophobic drug, and (2) the effect of targeting by IL-3. In contrast, treatment on Molt 4 cells as negative control showed no reduction of IC50 for PMBN-IL-PTX compared with free PTX or PMBN-PTX (Table 1). The reason would be the lack of expression of CD123 receptor. Fascinatingly, by considering Fig. 3 and 4, and comparing IC50 results, the toxicity rate of GA-A, a fungal metabolite, was increased when PMBN-IL conjugate was about 80% for 24 hour treatment and 75% after 48 hours. Furthermore, comparison of PMBN-IL-GA with PMBN-GA showed around 75% reduction of IC50 on average. In this case, as was mentioned above, the important factors for increasing toxicity of the nano smart carrier loaded with GA-A were cell targeting and solubilization of the hydrophobic agent.38 According to previous studies, the PMBN polymer is not of itself toxic to cells.26 As shown in Fig. 5 and 6, there was no evidence for toxicity of PMBN and the PMBN-IL3 conjugate. Generally, by MTT assay, in each well that contained the highest sample concentration, the percentage of viable cells was greater than 70%, from which it was concluded that the sample had no anti-proliferation activity. Another remarkable point is the role of IL-3 ligand as an inducer of blood cell proliferation. Fig. 6 shows percentage viability of the Kg-1 cell line, as a positive control, which was 95.8% at the highest concentration of PMBN-IL3 (1000 μg mL−1). However, this amount for Molt 4, as a negative control cell line, was 70.3%. So, the targeting of PMBN has been achieved successfully.
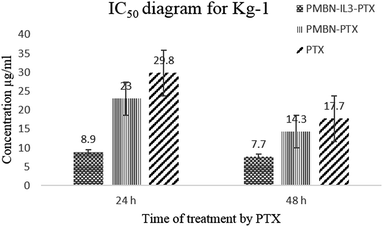 |
| Fig. 1 IC50 values for inhibition of proliferation of Kg-1 cell line by PMBN-IL3 conjugate incorporating PTX, PMBN incorporating PTX, and conventional PTX. The first conjugate polymer inhibited proliferation of the Kg-1 cell line at much lower concentration than unconjugated pure PTX. There was no significant difference between toxicity of PMBN-PTX and pure PTX. Each assay was repeated three times, and the P value was lower than 0.05 for each column according to the Mann–Whitney U test. | |
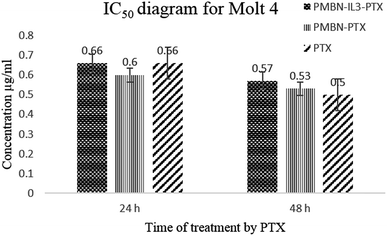 |
| Fig. 2 IC50 values for inhibition of proliferation of Molt 4 cell line by PMBN-IL3 conjugate incorporating PTX, PMBN incorporating PTX, and conventional PTX. There was no difference between their toxicity. Each assay was repeated three times, and the P value was higher than 0.05 for each column according to the Mann–Whitney U test. | |
Table 1 IC50 comparison between different treatment conditions on Kg-1 and Molt 4 cell lines after 24 and 48 hours
Cell line |
IC50 comparison |
After 24 h treatment |
After 48 h treatment |
Kg-1 |
PMBN-IL3-PTX compared with free PTX |
70% |
56.5% |
Kg-1 |
PMBN-PTX compared with free PTX |
22.8% |
19.2% |
Kg-1 |
PMBN-IL3-PTX compared with PMBN-PTX |
61.3% |
46.1% |
Molt 4 |
PMBN-IL3-PTX compared with free PTX |
0% |
−12.2% |
Molt 4 |
PMBN-PTX compared with free PTX |
9% |
−5.6% |
Molt 4 |
PMBN-IL3-PTX compared with PMBN-PTX |
−9% |
−7% |
Kg-1 |
PMBN-IL3-GA compared with GA-A |
79.5% |
75.4% |
Kg-1 |
PMBN-GA compared with free GA-A |
9% |
8.5% |
Kg-1 |
PMBN-IL3-GA compared with PMBN-GA |
77.5% |
73.1% |
Molt 4 |
PMBN-IL3-GA compared with GA-A |
13.4% |
15.8% |
Molt 4 |
PMBN-GA compared with free GA-A |
11.3% |
3.9% |
Molt 4 |
PMBN-IL3-GA compared with PMBN-GA |
2.4% |
12.1% |
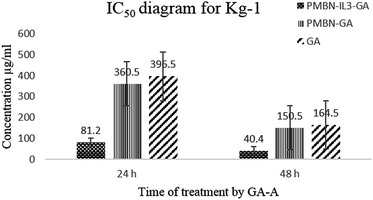 |
| Fig. 3 IC50 values for inhibition of proliferation of Kg-1 cell line by PMBN-IL3 conjugate incorporating GA-A, PMBN incorporating GA-A, and conventional GA. The first conjugate polymer inhibited proliferation of the Kg-1 cell line at much lower concentration than unconjugated pure GA-A. There was no significant difference between toxicity of PMBN-GA-A and pure GA-A. Each assay was repeated three times, and the P value was lower than 0.05 for each column according to the Mann–Whitney U test. | |
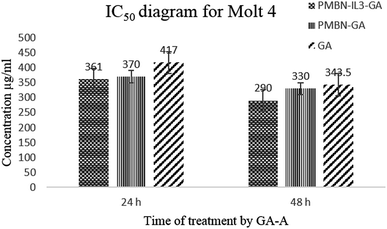 |
| Fig. 4 IC50 values for inhibition of proliferation of Molt 4 cell line by PMBN-IL3 conjugate incorporating GA-A, PMBN incorporating GA-A, and conventional GA-A. There was no difference between their toxicity. Each assay was repeated three times, and the P value was higher than 0.05 for each column according to the Mann–Whitney U test. | |
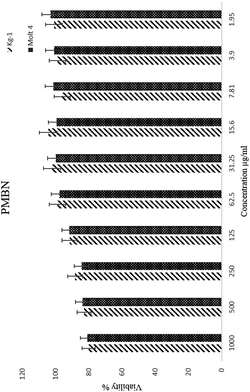 |
| Fig. 5 The inhibition of proliferation of Kg-1 and Molt 4 cell lines by PMBN alone. Free PMBN was non-toxic even at high concentration. | |
 |
| Fig. 6 The inhibition of proliferation of Kg-1 and Molt 4 cell lines by PMBN-IL3 conjugate. The PMBN-IL3 was non-toxic, and slightly increased proliferation of Kg-1 because of the presence of IL-3 as an inducer, but as expected it was not an inducer for the Molt 4 cell line since it was CD123−. | |
Apoptosis assay of cell lines by PMBN-IL3 incorporating PTX and GA-A
The cell viability of the Kg-1 cell line without added agent or any polymer after 24 hours was 94.61%, which indicated that cells were in the logarithmic phase of growth. Apoptosis induction was measured relative to this amount. Treatment of cells with conventional PTX (31.25 μg mL−1) for 24 h led to 69.53% viable cells (Q3 zone), 25.59% of cells in apoptosis (Q4), and finally 3.86% cells in apoptosis–necrosis (Q2 zone). On treating positive cells with nano smart carrier PMBN-IL-PTX (15.62 μg mL), 51.9% of cells were alive, and 47.15% of cells were in the apoptosis and apoptosis–necrosis zones (Fig. 7A). By comparing the above-mentioned results, although the concentration ratio of nano carrier to pure PTX was 1/2, the apoptosis rate was increased by about 25%. So, it is clear that, during targeting by PMBN-IL-3, the carrier was connected to CD123 on the surface of the cell membrane and facilitated penetration of the incorporated system into the cells, which caused enhancement of the toxicity and apoptosis.36 This process was carried out for the negative cell line Molt 4, which had 94.94% viable cells. As seen in Fig. 7B, pure PTX treatment afforded 39.21% viable cells, and 59% of cells in apoptosis and necrosis, corresponding to Q3, and Q2 + Q4 zones, respectively. Fig. 8 shows that after PMBN-IL3-PTX treatment of cells with the same concentration of free PTX (1.95 μg mL−1), 45.26% of cells were alive, and 56.22% of them were in apoptosis. Consequently, by comparing these results with the previous ones, no significant differences were achieved for the Molt 4 cell line. The reason could be that CD123 ligand was not expressed on the surfaces joining to IL-3, and so the activity of the nano smart carrier was the same as that of free PTX.36
 |
| Fig. 7 (A) The apoptosis assay and (B) plot of assay for PMBN-IL3-PTX nano system compared with free PTX and control on positive cell line Kg-1. The enhancement of apoptosis and necrosis is clear. (C) The apoptosis assay and (D) plot of assay on negative cell line Molt 4. There was no enhancement of apoptosis and necrosis. | |
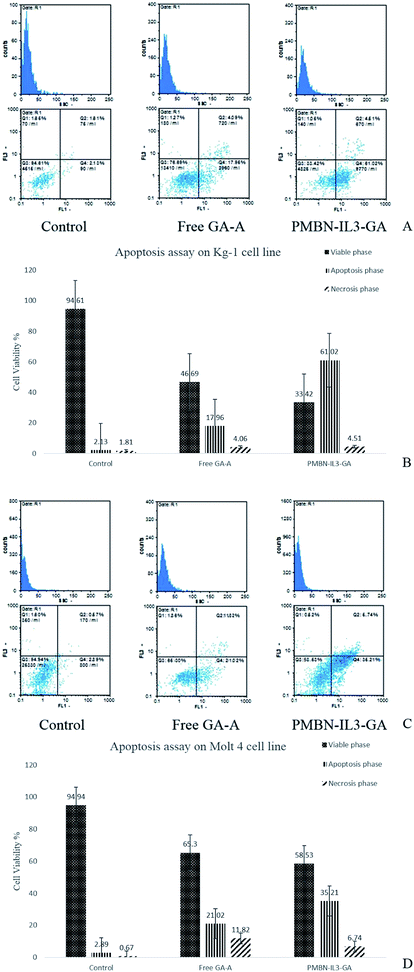 |
| Fig. 8 (A) The apoptosis assay and (B) plot of assay for PMBN-IL3-GA nano system compared with free GA-A, and control on positive cell line Kg-1. The enhancement of apoptosis and necrosis is clear. (C) The apoptosis and (D) plot of assay on negative cell line Molt 4. There is no enhancement of apoptosis and necrosis. | |
In this study, the new nano incorporated system PMBN-IL3-GA was for the first time, we believe, analyzed by apoptosis assay on positive and negative control cell lines to discover its efficiency compared with PTX. The percentage viabilities of Kg-1 and Molt 4 were as mentioned above. Fig. 8A shows the effect of GA-A treatment alone on the Kg-1 cell line. The concentration of the fungal metabolite was 625 μg mL−1, and after 24 h it caused 22.04% of cells to be in the apoptosis and necrosis zones (Q2 and Q4); 76.69% were still alive. In contrast, when PMBN-IL3-GA was added to the cells, after 24 h 65.53% of cells were in the apoptosis–necrosis phase and just 33.42% viable cells were observed (Fig. 8A). It should be taken into consideration that the concentration of conjugate was 312 μg mL−1, namely about half of the concentration of free GA-A, but apoptosis was increased by about 66%. This incredible result was due to targeting by the polymer and solubilization of the hydrophobic agent, which led to enhancement of the effectiveness of the metabolite. This assay was also utilized for Molt 4. Fig. 8B shows apoptosis analysis of Molt 4 cells treated with free GA-A and with PMBN-IL3 incorporating GA-A. The viable cells were about 65.30% for the first and 58.53% for the second (Q3 zone). Also, the necrosed cells were 32.84% and 41.95%, respectively. As the concentration in both cases was the same (625 μg mL−1 and 24 h treatment), it can be concluded that Molt 4, a CD123-negative cell line, having no CD123 receptor to connect with IL-3, causes no penetration of nano systems into cells, in agreement with previous studies.36,37
The apoptosis effect of PMBN and PMBN-IL3 on the Kg-1 cell line was analyzed, and no toxicity was observed. Just 4% viability enhancement by treatment with PMBN conjugated with IL-3 occurred compared with free PMBN, which may be a result of the effect of IL-3 as an inducer of proliferation (data not shown).
Conclusions
In the present study, AML LSCs were targeted for the first time, we believe, based on the PMBN-IL3 conjugate. The efficiency of conjugation was about 75%, which is a good achievement. Conjugate incorporating PTX as a conventional chemotherapy agent or GA-A as a fungal metabolite (for the first time, we believe) was used. In a similar work by Chiba et al., PMBN was conjugated to IL-2 and PTX was incorporated;26 the results showed reduction of IC50. In another study, in which PMBN was conjugated to epidermal growth factor, EGF, toxicity enhancement was confirmed, compared with free PTX and PMBN-PTX with no ligand.39 Nonetheless, this fungal metabolite has not been used incorporated in PMBN polymer. The efficiency of incorporation of PTX and GA-A was about 87% and 77%, respectively, which indicated, interestingly, that this polymer could solubilize these hydrophobic agents to a greater extent. According to previous studies, PMBN enters cells by direct penetration, which solves the problem of drug release by nano smart carrier close to targeted cells.30 Furthermore, this mechanism was confirmed by simulation methods like Monte Carlo.40,41 The toxicity and apoptosis analysis confirmed more than 60% enhancement of anti-proliferation for the conjugate polymer on the positive cell line. By comparing PMBN-IL3-incorporated PTX or GA-A with PMBN-incorporated agents without targeting, the IC50 was decreased more than 60%, which confirmed targeting of drug delivery to the cells was successful. Using the negative cell line, there were no differences between free agent and non-targeted polymer. As a result, PMBN has two remarkable capacities: it is targetable based on MEONP and it can penetrate the cells directly. This could be one of the best choices for DDSs, and PMBN-IL3-GA would be a good option for treatment of leukemia stem cells, because of lower side effects of this metabolite compared with chemotherapy agents like PTX. Although some studies have been performed to determine toxicity of ganoderic acids in blood cell cancer,31 none of them was specifically based on the derivative GA-A. In vivo assay and animal study of the designed nano system to find its effectiveness for successful treatment are suggested.
References
- C. Jordan, D. Upchurch, S. Szilvassy, M. Guzman, D. Howard and A. Pettigrew, et al., The interleukin-3 receptor alpha chain is a unique marker for human acute myelogenous leukemia stem cells, Leukemia, 2000, 14(10), 1777–1784 CrossRef CAS PubMed.
- U. Testa, R. Riccioni, S. Militi, E. Coccia, E. Stellacci and P. Samoggia, et al., Elevated expression of IL-3Rα in acute myelogenous leukemia is associated with enhanced blast proliferation, increased cellularity, and poor prognosis, Blood, 2002, 100(8), 2980–2988 CrossRef CAS PubMed.
- L. Muñoz, J. F. Nomdedéu, O. López, M. J. Carnicer, M. Bellido and A. Aventín, et al., Interleukin-3 receptor alpha chain (CD123) is widely expressed in hematologic malignancies, Haematologica, 2001, 86(12), 1261–1269 Search PubMed.
- Y. Guan, B. Gerhard and D. E. Hogge, Detection, isolation, and stimulation of quiescent primitive leukemic progenitor cells from patients with acute myeloid leukemia (AML), Blood, 2003, 101(8), 3142–3149 CrossRef CAS PubMed.
- M. Dean, T. Fojo and S. Bates, Tumour stem cells and drug resistance, Nat. Rev. Cancer, 2005, 5(4), 275–284 CrossRef CAS PubMed.
- M. Konopleva, S. Zhao, W. Hu, S. Jiang, V. Snell and D. Weidner, et al., The anti-apoptotic genes Bcl-XL and Bcl-2 are over-expressed and contribute to chemoresistance of non-proliferating leukaemic CD34+ cells, Br. J. Haematol., 2002, 118(2), 521–534 CrossRef CAS PubMed.
- K. Liu, M. Zhu, Y. Huang, S. Wei, J. Xie and Y. Xiao, CD123 and its potential clinical application in leukemias, Life Sci., 2015, 122, 59–64 CrossRef CAS PubMed.
- G. Li, X. Li, H. Wu, X. Yang, Y. Zhang and L. Chen, et al., CD123 targeting oncolytic adenoviruses suppress acute myeloid leukemia cell proliferation in vitro and in vivo, Blood Canc J, 2014, 4(3), e194 CrossRef CAS PubMed.
- S. Al-Musawi, H. Naderi-Manesh, Z. Mohammad Hassan, H. Yeganeh, S. Nikzad and H. Kheiri Manjili, Construction of Polyurethane Polymeric-based Nano-carriers for Curcumin in Cancer Therapy, Modares Journal of Medical Sciences: Pathobiology, 2014, 17(4), 25–39 Search PubMed.
- H. Danafar, A. Sharafi, H. Kheiri Manjili and S. Andalib, Sulforaphane delivery using mPEG–PCL co-polymer nanoparticles to breast cancer cells, Pharm. Dev. Technol., 2016, 1–10 CAS.
- L. Ma'mani, S. Nikzad, H. Kheiri-Manjili, S. al-Musawi, M. Saeedi and S. Askarlou, et al., Curcumin-loaded guanidine functionalized PEGylated I3ad mesoporous silica nanoparticles KIT-6: Practical strategy for the breast cancer therapy, Eur. J. Med. Chem., 2014, 83, 646–654 CrossRef PubMed.
- H. K. Manjili, L. Ma'mani, S. Tavaddod, M. Mashhadikhan, A. Shafiee and H. D. Naderi-Manesh, -Sulforaphane Loaded Fe3O4@Gold Core Shell Nanoparticles: A Potential Sulforaphane Delivery System, PLoS One, 2016, 11(3), e0151344 Search PubMed.
- H. K. Manjili, A. Sharafi, H. Danafar, M. Hosseini, A. Ramazani and M. H. Ghasemi, Poly(caprolactone)–poly(ethylene glycol)–poly(caprolactone) (PCL–PEG–PCL) nanoparticles: a valuable and efficient system for in vitro and in vivo delivery of curcumin, RSC Adv., 2016, 6(17), 14403–14415 RSC.
- P. Aggarwal, J. B. Hall, C. B. McLeland, M. A. Dobrovolskaia and S. E. McNeil, Nanoparticle interaction with plasma proteins as it relates to particle biodistribution, biocompatibility and therapeutic efficacy, Adv. Drug Delivery Rev., 2009, 61(6), 428–437 CrossRef CAS PubMed.
- M. A. Phillips, M. L. Gran and N. A. Peppas, Targeted nanodelivery of drugs and diagnostics, Nano Today, 2010, 5(2), 143–159 CrossRef CAS PubMed.
- N. Wiradharma, Y. Zhang, S. Venkataraman, J. L. Hedrick and Y. Y. Yang, Self-assembled polymer nanostructures for delivery of anticancer therapeutics, Nano Today, 2009, 4(4), 302–317 CrossRef CAS.
- K. Ishihara and M. Takai, Bioinspired interface for nanobiodevices based on phospholipid polymer chemistry, J. R. Soc., Interface, 2009, 6(3), S279–S291 CrossRef CAS PubMed.
- Molecular-Integrated Phospholipid Polymer Nanoparticles with High Biofunctionality. Macromolecular symposia, ed. Matsuno R. and Ishihara K., Wiley Online Library, 2009 Search PubMed.
- Structure and Surface Properties of High-density Polyelectrolyte Brushes at the Interface of Aqueous Solution. Macromolecular symposia, ed. Kobayashi M., Yamaguchi H., Terayama Y., Wang Z., Ishihara K. and Hino M., et al., Wiley Online Library, 2009 Search PubMed.
- H. Ueda, J. Watanabe, T. Konno, M. Takai, A. Saito and K. Ishihara, Asymmetrically functional surface properties on biocompatible phospholipid polymer membrane for bioartificial kidney, J. Biomed. Mater. Res., Part A, 2006, 77(1), 19–27 CrossRef PubMed.
- T. Moro, Y. Takatori, K. Ishihara, T. Konno, Y. Takigawa and T. Matsushita, et al., Surface grafting of artificial joints with a biocompatible polymer for preventing periprosthetic osteolysis, Nat. Mater., 2004, 3(11), 829–836 CrossRef CAS PubMed.
- Y. Iwasaki and K. Ishihara, Cell membrane-inspired phospholipid polymers for developing medical devices with excellent biointerfaces, Sci. Technol. Adv. Mater., 2016, 13, 6 Search PubMed.
- T. Konno, J. Watanabe and K. Ishihara, Enhanced solubility of paclitaxel using water-soluble and biocompatible 2-methacryloyloxyethyl phosphorylcholine polymers, J. Biomed. Mater. Res., Part A, 2003, 65(2), 209–214 CrossRef PubMed.
- M. Wada, H. Jinno, M. Ueda, T. Ikeda, M. Kitajima and T. Konno, et al., Efficacy of an MPC-BMA co-polymer as a nanotransporter for paclitaxel, Anticancer Res., 2007, 27(3B), 1431–1435 CAS.
- H. I. Kim, M. Takai, T. Konno, R. Matsuno and K. Ishihara, Biodegradable polymer films for releasing nanovehicles containing sirolimus, Drug Delivery, 2009, 16(4), 183–188 CrossRef CAS PubMed.
- N. Chiba, M. Ueda, T. Shimada, H. Jinno, J. Watanabe and K. Ishihara, et al., Novel immunosuppressant agents targeting activated lymphocytes by biocompatible MPC polymer conjugated with interleukin-2, Eur. Surg. Res., 2007, 39(2), 103–110 CrossRef CAS PubMed.
- X. Zhao, Z. Zhang, F. Pan, Y. Ma, S. P. Armes and A. L. Lewis, et al., DNA immobilization using biocompatible diblock phosphorylcholine copolymers, Surf. Interface Anal., 2006, 38(4), 548–551 CrossRef CAS.
- T. Kitagawa, R. Iwase, K. Ishihara, T. Yamaoka and A. Murakami, Facilitated disassembly of polyplexes composed of self-assembling amphiphilic polycations enhances the gene transfer efficacy, Chem. Lett., 2005, 34(11), 1478–1479 CrossRef CAS.
- A. M. Funhoff, C. F. van Nostrum, G. A. Koning, N. M. Schuurmans-Nieuwenbroek, D. J. Crommelin and W. E. Hennink, Endosomal escape of polymeric gene delivery complexes is not always enhanced by polymers buffering at low pH, Biomacromolecules, 2004, 5(1), 32–39 CrossRef CAS PubMed.
- T. Goda, K. Ishihara and Y. Miyahara, Critical update on 2-methacryloyloxyethyl phosphorylcholine (MPC) polymer science, J. Appl. Polym. Sci., 2015, 132(16), 10 CrossRef.
- C. W. Huie and X. Di, Chromatographic and electrophoretic methods for Lingzhi pharmacologically active components, J. Chromatogr. B: Anal. Technol. Biomed. Life Sci., 2004, 812(1), 241–257 CrossRef CAS.
- J. W. Yuen and M. D. I. Gohel, Anticancer effects of Ganoderma lucidum: a review of scientific evidence, Nutr. Cancer, 2005, 53(1), 11–17 CrossRef CAS PubMed.
- R.-Y. Chen and D.-Q. Yu, Studies on the triterpenoid constituents of the spores of Ganoderma lucidum (Curt.: Fr.) P. Karst. (Aphyllophoromycetideae), Int. J. Med. Mushrooms, 1999, 1(2), 147–152 CrossRef CAS.
- E. Calviño, J. L. Manjón, P. Sancho, M. C. Tejedor, A. Herráez and J. C. Diez, Ganoderma lucidum induced apoptosis in NB4 human leukemia cells: Involvement of Akt and Erk, J. Ethnopharmacol., 2010, 128(1), 71–78 CrossRef PubMed.
- C. I. Müller, T. Kumagai, J. O'Kelly, N. P. Seeram, D. Heber and H. P. Koeffler, Ganoderma lucidum causes apoptosis in leukemia, lymphoma and multiple myeloma cells, Leuk. Res., 2006, 30(7), 841–848 CrossRef PubMed.
- N. Misaghian, G. Ligresti, L. Steelman, F. E. Bertrand, J. Bäsecke and M. Libra, et al., Targeting the leukemic stem cell: the Holy Grail of leukemia therapy, Leukemia, 2009, 23(1), 25–42 CrossRef CAS PubMed.
- L. Yalcintepe, A. E. Frankel and D. E. Hogge, Expression of interleukin-3 receptor subunits on defined subpopulations of acute myeloid leukemia blasts predicts the cytotoxicity of diphtheria toxin interleukin-3 fusion protein against malignant progenitors that engraft in immunodeficient mice, Blood, 2006, 108(10), 3530–3537 CrossRef CAS PubMed.
- A. A. Shtil, Emergence of multidrug resistance in leukemia cells during chemotherapy: mechanisms and prevention, J. Hematother. Stem Cell Res., 2002, 11(2), 231–241 CrossRef CAS PubMed.
- T. Shimada, M. Ueda, H. Jinno, N. Chiba, M. Wada and J. Watanabe, et al., Development of targeted therapy with paclitaxel incorporated into EGF-conjugated nanoparticles, Anticancer Res., 2009, 29(4), 1009–1014 CAS.
- J.-U. Sommer, M. Werner and V. A. Baulin, Critical adsorption controls translocation of polymer chains through lipid bilayers and permeation of solvent, EPL, 2012, 98(1), 18003 CrossRef.
- M. Werner, J.-U. Sommer and V. A. Baulin, Homo-polymers with balanced hydrophobicity translocate through lipid bilayers and enhance local solvent permeability, Soft Matter, 2012, 8(46), 11714–11722 RSC.
Footnotes |
† Dynamic light scattering. |
‡ Scanning electron microscope. |
§ Transmission electron microscope. |
¶ Field emission scanning electron microscope. |
|
This journal is © The Royal Society of Chemistry 2016 |
Click here to see how this site uses Cookies. View our privacy policy here.