DOI:
10.1039/C5SC03903G
(Edge Article)
Chem. Sci., 2016,
7, 1383-1387
β-Arylation of oxime ethers using diaryliodonium salts through activation of inert C(sp3)–H bonds using a palladium catalyst†
Received
14th October 2015
, Accepted 11th November 2015
First published on 11th November 2015
Abstract
An efficient method of selective β-arylation of oxime ethers was realized by using a palladium catalyst with diaryliodonium salts as the key arylation reagents. The reaction proceeded smoothly through the activation of inert C(sp3)–H bonds to give corresponding ketones and aldehydes. This convenient procedure can be successfully applied to construct new C(sp3)–C(sp2) bonds on a number of complex molecules derived from natural products and thus serves as a practical synthetic tool for direct late-stage C(sp3)–H functionalization.
Introduction
Arylation via direct activation of inert C–H bonds has emerged as a fascinating field, which could provide useful aromatic compounds with high atom- and step-economy.1 In the past decade, significant progress has been made in the development of transition-metal catalyzed arylation on C(sp2)–H which enables coupling a large range of substrates to various aromatic reagents,2 including more challenging work on enantioselective construction of stereo-centers published most recently.2j–l In comparison, arylation on an inert C(sp3)–H bond (simple alkyl C–H bond) is much less explored, with a scope of limited substrates reported.3 One of the most successful strategies is transition-metal catalyzed-arylation of carboxylate derivatives, including carboxylic acids, esters and amides.4 Assisted by big auxiliary groups, alkyl amines could also be selectively arylated on the chain.5
In light of these advances, we were encouraged to develop new arylation reactions through direct activation on alkyl C–H bonds with a wider scope of substrates which offers unprecedented opportunities to efficiently synthesize valuable aromatic compounds.6 With regard to chelation-assisted C–H bond functionalization, facile introduction and removal of the directing group could enlarge the scope of substrates from pre-designed molecules and consequently enable the protocol to be applicable to various natural products, generating new and attractive sources for bioactive compounds with high diversity and functionality.7 With this ultimate goal in mind, we successfully developed a Pd-catalyzed β-arylation reaction on the inert C(sp3)–H bond of oxime ethers to give useful products, which could be easily converted to important β-aryl ketones, amines and so on.8 The reaction proceeds smoothly via C(sp3)–H activation with diaryliodonium salts as the key coupling partner reagents (Scheme 1).
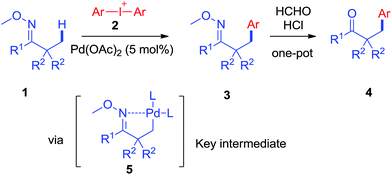 |
| Scheme 1 Pd-catalyzed β-arylation reaction of oxime ethers via C(sp3)–H bond activation. | |
Results and discussion
As revealed by known work, the main issues of transition-metal catalyzed functionalization on C(sp3)–H bonds not only resulted from the inertness and abundance of C(sp3)–H bonds in organic compounds but, more essentially, from the inherent instability of in situ generated catalytic metal species, which may easily undergo β-hydrogen elimination or side reactions.9 To some extent, a good solution is adjusting electronic and coordination effects of the catalytic species, but this generally required additional modification of the substrates.10 Hence, a more straightforward solution might be to accelerate the transformation of the catalytic metal species by accomodating the proper coupling reagents with an appropriate chelating group.11 With this strategy, we successfully realized the β-arylation reaction on the C(sp3)–H bond of oxime ethers with Pd(OAc)2 as the catalyst. During the study of C–C bond formation on inert C–H bonds, we initiated the investigation with arylating 2-methylcyclohexanone O-methyl oxime, which is quantitatively synthesized from α-methylcyclohexanone. To begin with, when PhBr or PhI was used as the coupling reagent under various conditions, it always failed to give the desired arylation product, 3aa, and dehydrogenation side products 1a′ and 1a′′ were observed with the formation of Pd-black. This implied that the putative Pd-species 5 was generated from 1a with Pd(OAc)2, but due to the instability, PhBr or PhI could not be coupled to species 5 before it underwent β-hydrogen-elimination. In order to facilitate the desired arylation reaction, Ph2I+PF6−, 2a·PF6− (1 equiv.) was chosen as the coupling reagent and 3aa was formed, albeit in 17% yield (Table 1).12 The addition of a base slightly increased the yield of 3aa, but the best yield was only 27% when Ag2CO3 (2 equiv.) was employed. By adding pivalic acid, the starting materials were fully converted to produce 3aa with 50% yield.13 In order to further accelerate the transfer step of the phenyl group, we added the polar solvent, hexafluoroisopropanol (HFIP) to the reaction, resulting in an increase to 82% yield of 3aa. The use of 2a·OTf− gave an even better result (87%, entry 17, isolated in 83%), while 2a·BF4− failed to generate 3aa. For further modification, when the reaction was completed under the optimized condition, entry 17, the mixture was treated with formaldehyde in an acidic system,14 and 2-benzyl cyclohexanone, 4aa, was isolated in 80% yield (for experimental details, see ESI†). In accordance with the initial proposal, ketoximes and ketones can be easily interconverted, making this method an efficient approach to β-aryl ketones.
Table 1 Optimization of reaction conditions for 3aaa
With the optimized conditions established, the scope of diaryliodonium salts was examined for β-arylation of 1a. As shown in Scheme 2, diaryliodonium salts with a range of substituents were efficiently coupled using these conditions. Most of the products were isolated in the ketone form (4) rather than oxime ethers (3), since ketones were considered to be more synthetically useful. The diaryliodonium triflates with F−, Cl− and Br− substituents at the para position (2b–2d) reacted smoothly with 1a, generating corresponding products (4ab–4ad) in good yields. The use of methyl and tbutyl substituted diphenyliodonium triflates (2e–2f) and 1a provided products (4ae–4af) in slightly lower yields. Diaryliodonium triflates bearing a strong electron-withdrawing group (–CO2Me and –CF3) or strong electron-donating group (–OMe) also yielded desired products (4ag–4ai) while 3ah was obtained under a lower temperature of 70 °C. The reaction of 1a with ortho-substituted diaryliodonium triflates (2j–2l) also afforded expected products (4aj–4al) in satisfactory yields. However, the use of asymmetric diaryliodonium salts Ar–I+-MesX− failed to give products. A number of selected oximes (3aa, 3ad, 3 ag, 3ah, 3ai, 3aj, and 3ak) were isolated to demonstrate the original efficiency.
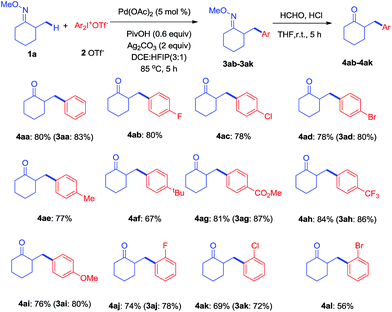 |
| Scheme 2 Scope of diaryliodonium salts to form desired β-arylated products. | |
To further investigate, a series of substituted acylic oxime substrates were examined to explore the regioselectivity of the arylation with di(4-bromophenyl)iodonium 2d. As shown in Scheme 3, ketoximes 1b–1d all reacted with 2d to give mono-arylated products at the α-methyl group in good yields, while the generation of bi-arylated compounds remained at trace amounts. The use of aldoxime 1e only produced 4ed in moderate yield while the bulky ketoxime 1f with four α-methyl groups afforded the mono-arylated product 4fd in good yield. In addition, we examined the effect of substituents on cyclohexyl oxime ethers and 4nd was isolated in 83% yield from 1n.
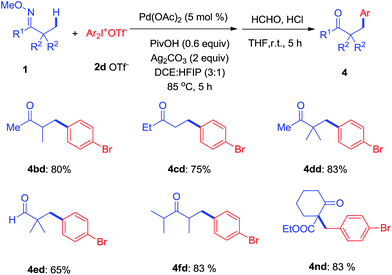 |
| Scheme 3
β-Arylation of selected oxime ethers with 2d. | |
It is known that the activation of methylene C–H bonds is harder than of methyl C–H bonds in transition-metal catalyzed reactions because C–H bonds in the methylene position are more hindered.15 As shown herein, some of the ketoximes with the appropriate configuration, for instance 1g, could successfully be arylated with 1d to give 4gd in 42% yield. Similarly, it also worked with an oxime ether containing a 1-adamantanyl group, 1h, to produce 3hg in 47% yield (Scheme 4).
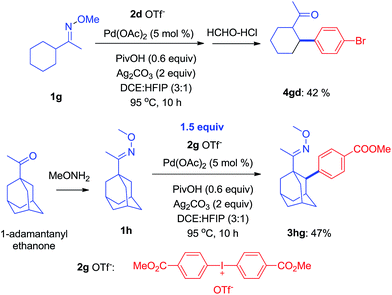 |
| Scheme 4 The activation of methylene C–H bonds to form C–C bonds. | |
Since the direct modification of natural products at a normally inert position has attracted much attention due to the potential to access new bioactive compounds from “starmolecules”,1a,16 some complex substrates with natural product backbones were examined in our new arylation reactions for reactivity, selectivity and tolerance of functional groups (Scheme 5). Oxime 1i, derived from naturally-occurred fenchone which contains three methyl groups, could be selectively arylated on an exo-methyl group with 2g under standard conditions, giving 3ig in 65% yield. Naturally abundant in many essential oils, (+)-carvone can be easily converted to α,β-unsaturated ketone oxime with α-methyl group 1j. This can then can be arylated with 2g to give 3jg under the synthetic method established, albeit in 38% yield. However, with 1 equivalent of diaryliodonium salt 2g, the mono-arylated product of 1k derived from lanosterol was observed as two inseparable isomers in a relevantly low yield. Alternatively, when 2 equivalents of 2g were employed in the transformation, the bis-arylated product 3kg was successfully obtained in 73% isolated yield. The structure of 3kg was confirmed by XRD analysis, shown in Fig. 1A. β-Glycyrrhetinic acid is a major metabolite of glycyrrhizin, one of the main constituents of licorice, and has been shown to exhibit anti-ulcerative, anti-inflammatory, and immunomodulatory properties. Substrate 1l, derived from glycyrrhetinic acid, could also be bis-arylated on both methyl C–H bonds to form 3lg with 69% isolated yield. Besides natural product-like compounds, the synthetic reagent, methasterone was converted to oxime 1m, which was then successfully arylated with 2d to give 4md in high yield, with the hydroxyl group unchanged during the reaction. In addition, oxime ethers can be easily converted to corresponding amines which are useful building blocks with a range of potential applications (Scheme 6).17
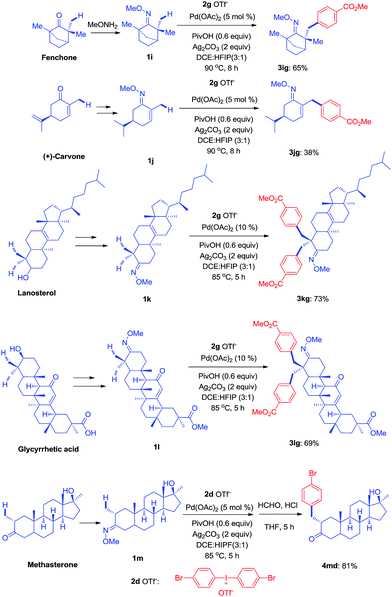 |
| Scheme 5 Modification of complex molecules derived from natural products (see ESI† for experimental details). | |
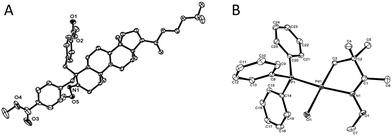 |
| Fig. 1 A (left), Crystal structure of compound 3kg; B (right), crystal structure of palladation intermediate 6 (see ESI† for detailed date of the crystals). | |
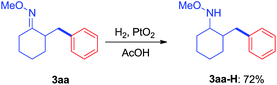 |
| Scheme 6 Hydrogenation of 3aa to generate 3aa-H. | |
In terms of the mechanism study, we proposed that the process was initiated by a cyclometalated complex.18 Using 1a as the starting backbone, the isolation of the palladation intermediate always failed due to the strong tendency of β-H elimination of 5a. When treating 1d with Pd(OAc)2, the existence of palladation intermediate 5d was proved. By converting 5d to 6 (Scheme 7),19 the crystal structure of 6 was identified, indicating that palladium was bound with CH2 and oxime-nitrogen atom as a cyclometalation species (Fig. 1B). In order to confirm the catalytic competency, complex 6 was treated with 1 equivalent of 2d and 2 equivalents of Ag2CO3, analogously to the standard reaction conditions, and 3dd was observed in 80% yield by in situ NMR (Scheme 7).
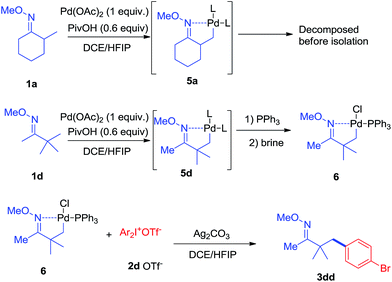 |
| Scheme 7 Preparation of the palladation intermediate and its reaction with 2d. | |
Based on the above results and literature reports, a plausible mechanism is proposed in Scheme 8. First, the reaction of oxime ether 1 with PdII species (Pd(OAc)2 or other palladium salts generated in situ) would produce cyclopalladation species 5. And the oxidative addition of diaryliodonium salt 2 to 5 would afford PdIV intermediate 7.11 The reductive elimination of 7 would give product 3, and release the PdII species into the catalytic cycle.
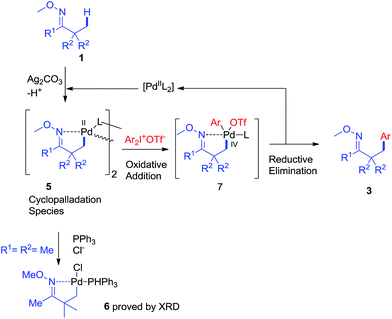 |
| Scheme 8 A plausible mechanism. | |
Conclusions
In summary, we have developed a novel β-arylation reaction on inert C(sp3)–H bonds of oxime ethers. The reaction offers new opportunities to prepare useful β-arylated oximes from/to ketones and aldehydes via simple transformation with good efficiency and step-economy. The easy manipulation and good tolerance of functional groups enable the method to be used to modify many complex compounds derived from natural backbones. Further investigations on the scope, mechanism, and synthetic application of this new reaction are under way in our laboratory.
Acknowledgements
This work was supported by National Natural Science Foundation of China (21102080 and 21290194), Tsinghua University Initiative Scientific Research Program (2011Z02150) and The National Key Basic Research Program of China (973 program) (2012CB933402).
Notes and references
-
(a) K. Godula and D. Sames, Science, 2006, 312, 67 CrossRef CAS PubMed;
(b) T. W. Lyons and M. S. Sanford, Chem. Rev., 2010, 110, 1147 CrossRef CAS PubMed;
(c) O. Baudoin, Chem. Soc. Rev., 2011, 40, 4902 RSC;
(d) J. Yamaguchi, A. D. Yamaguchi and K. Itami, Angew. Chem., Int. Ed., 2012, 51, 8960 CrossRef CAS PubMed;
(e) P. B. Arockiam, C. Bruneau and P. H. Dixneuf, Chem. Rev., 2012, 112, 5879 CrossRef CAS PubMed;
(f) G. Rouquet and N. Chatani, Angew. Chem., Int. Ed., 2013, 52, 11726 CrossRef CAS PubMed.
- For selective examples, see:
(a) D. A. Culkin and J. F. Hartwig, Acc. Chem. Res., 2003, 36, 234 CrossRef CAS PubMed;
(b) H. M. L. Davies, Angew. Chem., Int. Ed., 2006, 45, 6422 CrossRef CAS PubMed;
(c) B.-F. Shi, N. Maugel, Y.-H. Zhang and J.-Q. Yu, Angew. Chem., Int. Ed., 2008, 47, 4882 CrossRef CAS PubMed;
(d) K. Godula and D. Sames, Science, 2009, 312, 67 CrossRef PubMed;
(e) R. J. Phipps and M. J. Gaunt, Science, 2009, 323, 1593 CrossRef CAS PubMed;
(f) M. P. Doyle, R. Duffy, M. Ratnikov and L. Zhou, Chem. Rev., 2010, 110, 704 CrossRef CAS PubMed;
(g) C.-L. Sun, B.-J. Li and Z.-J. Shi, Chem. Rev., 2011, 111, 1293 CrossRef CAS PubMed;
(h) H. Li, B.-J. Li and Z.-J. Shi, Catal. Sci. Technol., 2011, 1, 191 RSC;
(i) K. Shin, S.-W. Park and S. Chang, J. Am. Chem. Soc., 2015, 137, 8584 CrossRef CAS PubMed;
(j) B. J. Stokes, L.-Y. Liao, A. M. Andrade, Q.-F. Wang and M. S. Sigman, Org. Lett., 2014, 16, 4666 CrossRef CAS PubMed;
(k) T.-S. Mei, H. P. Harshkumar and M. S. Sigman, Nature, 2014, 508, 340 CrossRef CAS PubMed;
(l) E. W. Werner, T.-S. Mei, A. J. Burckle and M. S. Sigman, Science, 2012, 338, 1455 CrossRef CAS PubMed;
(m) E. Cahard, H. P. J. Male, M. Tissot and M. J. Gaunt, J. Am. Chem. Soc., 2015, 137, 7986 CrossRef CAS PubMed.
-
(a) A. E. Allen and D. W. C. MacMillan, J. Am. Chem. Soc., 2011, 133, 4260 CrossRef CAS PubMed;
(b) Y. Takeda, Y. Ikeda, A. Kuroda, S. Tanaka and S. Minakata, J. Am. Chem. Soc., 2014, 136, 8544 CrossRef CAS PubMed;
(c) M. T. Pirnot, D. A. Rankic, D. B. C. Martin and D. W. C. MacMillan, Science, 2013, 339, 1593 CrossRef CAS PubMed.
-
(a) M. Jørgensen, S. Lee, X.-X. Liu, J. P. Wolkowski and J. F. Hartwig, J. Am. Chem. Soc., 2002, 124, 12557 CrossRef;
(b) V. G. Zaitsev, D. Shabashov and O. Daugulis, J. Am. Chem. Soc., 2005, 127, 13154 CrossRef CAS PubMed;
(c) Y. Feng, Y. Wang, B. Landgraf, S. Liu and G. Chen, Org. Lett., 2010, 12, 3414 CrossRef CAS PubMed;
(d) A. Renaudat, L. Jean-Gerard, R. Jazzar, C. E. Kefalidis, E. Clot and O. Baudoin, Angew. Chem., Int. Ed., 2010, 49, 7261 CrossRef CAS PubMed;
(e) M. Wasa, K. M. Engle, D. W. Lin, E. J. Yoo and J.-Q. Yu, J. Am. Chem. Soc., 2011, 133, 19598 CrossRef CAS PubMed;
(f) S. Aspin, A. S. Goutierre, P. Larini, R. Jazzar and O. Baudoin, Angew. Chem., Int. Ed., 2012, 51, 10808 CrossRef CAS PubMed;
(g) R. Shang, L. Ilies, A. Matsumoto and E. J. Nakamura, J. Am. Chem. Soc., 2013, 135, 6030 CrossRef CAS PubMed;
(h) F. Pan, P.-X. Shen, L.-S. Zhang, X. Wang and Z.-J. Shi, Org. Lett., 2013, 15, 4758 CrossRef CAS PubMed.
-
(a) B. V. S. Reddy, L. R. Reddy and E. J. Corey, Org. Lett., 2006, 8, 3391 CrossRef CAS PubMed;
(b) N. Rodriguez, J. A. Romero-Revilla, M. A. Fernandez-Ibanez and J. C. Carretero, Chem. Sci., 2013, 4, 175 RSC;
(c) K. S. L. Chan, M. Wasa, L. Chu, B. N. Laforteza, M. Miura and J.-Q. Yu, Nat. Chem., 2014, 6, 146 CrossRef CAS PubMed;
(d) K. S. L. Chan, H.-Y. Fu and J.-Q. Yu, J. Am. Chem. Soc., 2015, 137, 2042 CrossRef CAS PubMed;
(e) A. Millet, D. Dailler, P. Larini and O. Baoudoin, Angew. Chem., Int. Ed., 2014, 53, 2678 CrossRef CAS PubMed.
- W. R. Gutekunst and P. S. Baran, Chem. Soc. Rev., 2011, 40, 1976 RSC.
-
(a) N. Martin, C. Pierre, M. Davi, R. Jazzar and O. Baudoin, Chem.–Eur. J., 2012, 18, 4480 CrossRef CAS PubMed;
(b) Z.-X. Huang and G.-B. Dong, J. Am. Chem. Soc., 2013, 135, 17747 CrossRef CAS PubMed;
(c) Y. Zhu and S. L. Buchwald, J. Am. Chem. Soc., 2014, 136, 4500 CrossRef CAS PubMed;
(d) S. Janody, R. Jazzar, A. Comte, P. Holstein, J.-P. Vors, M. J. Ford and O. Baudoin, Chem.–Eur. J., 2014, 20, 11084 CrossRef CAS PubMed.
-
(a) Z.-X. Huang, Q. P. Sam and G.-B. Dong, Chem. Sci., 2015, 6, 5491 RSC;
(b) Z.-X. Huang and G.-B. Dong, J. Am. Chem. Soc., 2013, 135, 17747 CrossRef CAS PubMed.
-
(a) J. Halpern, Acc. Chem. Res., 1982, 15, 332 CrossRef CAS;
(b) Z.-G. Zhang, X.-Y. Lu, Z.-R. Xu, Q.-H. Zhang and X.-L. Han, Organometallics, 2001, 20, 3724 CrossRef CAS;
(c) A. DeAngelis, P. Panne, G. P. A. Yap and J. M. Fox, J. Org. Chem., 2008, 73, 1435 CrossRef CAS PubMed;
(d) L.-B. Huang, J. Qi, X. Wu, K.-F. Huang and H.-F. Jiang, Org. Lett., 2013, 15, 2330 CrossRef CAS PubMed.
-
(a) H.-T. Zhao, A. Ariafard and Z.-Y. Lin, Organometallics, 2006, 25, 812 CrossRef CAS;
(b) K. M. Engle, T.-S. Mei, M. Wasa and J.-Q. Yu, Acc. Chem. Res., 2012, 45, 788 CrossRef CAS PubMed;
(c) K. Shibata, T. Yamaguchi and N. Chatani, Org. Lett., 2015, 17, 3584 CrossRef CAS PubMed.
-
(a) N. M. Camasso, M. H. Perez-Temprano and M. S. Sanford, J. Am. Chem. Soc., 2014, 136, 12771 CrossRef CAS PubMed;
(b) S. D. Timpa, C. J. Pell, J. Zhou and O. V. Ozerov, Organometallics, 2014, 33, 5254 CrossRef CAS;
(c) J. J. Topczewski and M. S. Sanford, Chem. Sci., 2015, 6, 70 RSC;
(d) A. R. Zhugralin, I. J. Kobylianskii and P. Chen, Organometallics, 2015, 34, 1301 CrossRef CAS.
-
(a) V. V. Zhdankin and P. J. Stang, Chem. Rev., 2002, 102, 2523 CrossRef CAS PubMed;
(b) V. V. Zhdankin and P. J. Stang, Chem. Rev., 2008, 108, 5299 CrossRef CAS PubMed;
(c) M. Bielawski, D. Aili and B. Olofsson, J. Org. Chem., 2008, 73, 4602 CrossRef CAS PubMed;
(d) M. Bielawski and B. Olofsson, Org. Synth., 2009, 86, 308 CrossRef CAS;
(e) C.-L. Sun and Z.-J. Shi, Chem. Rev., 2014, 114, 9219 CrossRef CAS PubMed.
-
(a) M. Lafrance and K. Fagnou, J. Am. Chem. Soc., 2006, 128, 16496 CrossRef CAS PubMed;
(b) D. B. Zhao, W.-D. Wang, S. Lian, F. Yang, J. B. Lan and J.-S. You, Chem.–Eur. J., 2009, 15, 1337 CrossRef CAS PubMed.
- S. Kim and J.-Y. Yoon, J. Am. Chem. Soc., 1997, 119, 5982 CrossRef CAS.
-
(a) J. A. Labinger and J. E. Bercaw, Nature, 2002, 417, 507 CrossRef CAS PubMed;
(b) R. B. Crabtree, J. Organomet. Chem., 2004, 689, 4083 CrossRef CAS;
(c) L. V. Desai, K. L. Hull and M. S. Sanford, J. Am. Chem. Soc., 2004, 126, 9542 CrossRef CAS PubMed;
(d) V. P. Ananikov, D. G. Musaev and K. Morokuma, Organometallics, 2005, 24, 715 CrossRef CAS;
(e) W. Liu and J. T. Groves, J. Am. Chem. Soc., 2010, 132, 12847 CrossRef CAS PubMed;
(f) Z. Ren, F.-Y. Mo and G.-B. Dong, J. Am. Chem. Soc., 2012, 134, 16991 CrossRef CAS PubMed;
(g) P. Gao, W. Guo, J. Xue, Y. Zhao, Y. Yuan, Y. Xia and Z. Shi, J. Am. Chem. Soc., 2015, 137, 12231 CrossRef CAS PubMed.
-
(a) W. R. Gutekunst, R. Gianatassio and P. S. Baran, Angew. Chem., Int. Ed., 2012, 51, 7507 CrossRef CAS PubMed;
(b) H. M. Davies and J. R. Manning, Nature, 2008, 451, 417 CrossRef CAS PubMed;
(c) W. R. Gutekunst and P. S. Baran, J. Am. Chem. Soc., 2011, 133, 19076 CrossRef CAS PubMed;
(d) L. McMurray, F. Ohara and M. J. Gaunt, Chem. Soc. Rev., 2011, 40, 1885 RSC;
(e) E. M. Simmons and J. F. Hartwig, Nature, 2012, 483, 70 CrossRef CAS PubMed.
- M. Jager, M. Hartmanm, J. G. de Vries and A. J. Minnaard, Angew. Chem., Int. Ed., 2013, 52, 7809 CrossRef PubMed.
-
(a) W. P. Hong, A. V. Iosub and S. S. Stahl, J. Am. Chem. Soc., 2013, 135, 13664 CrossRef CAS PubMed;
(b) L. Menéndez-Rodríguez, E. Tomás-Mendivil, J. Francos, C. Nájera, P. Crochet and V. Cadierno, Catal. Sci. Technol., 2015, 5, 3754 Search PubMed;
(c) K. Guo, X.-L. Chen, M.-Y. Guan and Y.-S. Zhao, Org. Lett., 2015, 17, 1802 CrossRef CAS PubMed;
(d) L. V. Desai, K. L. Hull and M. S. Sanford, J. Am. Chem. Soc., 2004, 126, 9542 CrossRef CAS PubMed;
(e) J. E. Baldwin, R. H. Jones, C. Najera and M. Yus, Tetrahedron, 1985, 41, 699 CrossRef CAS;
(f) A. G. Constable, W. S. McDonald, L. C. Sawkins and B. L. Shaw, J. Chem. Soc., Chem. Commun., 1978, 23, 1061 RSC.
- A. McNally, B. Haffemayer, B. S. L. Collins and M. J. Gaunt, Nature, 2014, 510, 129 CrossRef CAS PubMed.
Footnote |
† Electronic supplementary information (ESI) available. CCDC 1057827 and 1048079. For ESI and crystallographic data in CIF or other electronic format see DOI: 10.1039/c5sc03903g |
|
This journal is © The Royal Society of Chemistry 2016 |
Click here to see how this site uses Cookies. View our privacy policy here.