DOI:
10.1039/C6AY02179D
(Critical Review)
Anal. Methods, 2017,
9, 10-17
The application of capillary electrophoretic on-line preconcentration in alkaloid analysis
Received
1st August 2016
, Accepted 28th October 2016
First published on 28th October 2016
Abstract
In this paper, the principles of capillary electrophoretic on-line preconcentration are introduced. The application of these techniques and their combinations in alkaloid analysis is reviewed. The trends of capillary electrophoretic on-line preconcentration in alkaloid analysis are discussed.
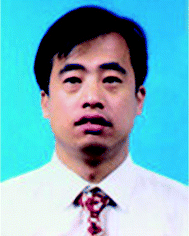 X. Hou | Xiangqian Hou is a post-doctoral student at the Department of Pharmacology, Shandong University. His research is focused on the application of capillary electrophoresis and related technologies in pharmacological studies. |
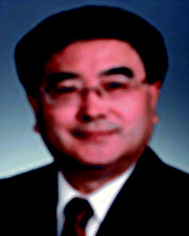 X. Zhang | Xiumei Zhang is a professor at the Department of Pharmacology, Shandong University. His research is focused on pharmacological studies. |
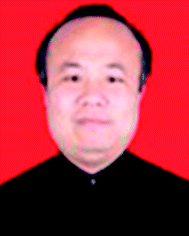 Y. Lu | Yuanqi Lu is a professor at the School of Chemistry and Chemical Engineering, Dezhou University. His research is focused on the application of capillary electrophoresis in traditional Chinese Medicine research. |
1. Introduction
With the advantages of high efficiency, short analysis time, low reagent and sample consumption, capillary electrophoresis (CE) has attracted the attention of analysts around the globe.1 But due to the small sample injection volume and short optical path length, it suffers from low sensitivity when compared to more traditional liquid chromatography, especially when an absorbance detector is applied. Although more sensitive detectors, such as a laser induced fluorescence detector (LIF),2 mass spectrometer (MS)3 and electrochemiluminescence detector,4 can overcome this problem, they are more expensive than an absorbance detector and have some limitations of their own.
To address this issue and to extend CE to trace constituents, a number of on-line preconcentration methods have been developed. These are based on migration changes of analytes in the sample zone and the background electrolyte or through association with additives moving through the sample such as surfactants, and various combinations of these two approaches. These on-line preconcentration techniques can enhance the sensitivity of CE up to several orders of magnitude, which make CE comparable to liquid chromatography in sensitivity and promote its application. As the sample and reagent consumption of CE is much lower than those of HPLC, which is good for the environment and conforms to the green development concept, capillary electrophoretic on-line preconcentration has been intensively investigated in recent years.
Alkaloids are natural products of metabolism found mainly in plants. Their diversified pharmaceutical activities as medical agents and presence in nutritional products demand the development of reliable and sensitive analytical tools.5 However, alkaloids often occur at very low levels, which is beyond the ability of capillary electrophoresis and, therefore, on-line preconcentration techniques are often used in alkaloid analysis. In this paper, the application of capillary electrophoretic on-line preconcentration techniques in alkaloid analysis is introduced and future developments are anticipated. Preconcentration methods for alkaloids have been discussed by Dziomba5 and Gotti,6 but they are not comprehensive and do not cover the literature published over the past three years.
2. Stacking based on migration changes of analytes between the sample zone and background electrolyte
2.1 Field amplified sample stacking (FASS) and large volume sample stacking (LVSS)
In this approach, analytes migrate much slower when they pass from the sample zone to the background electrolyte, thus they are stacked at the interface between the two zones. The migration changes can be caused by ionic strength differences. When the sample has a lower conductivity than the background electrolyte, this is called field-amplified sample stacking (FASS) and is the easiest on-line preconcentration method to perform. Although it is very simple and convenient, there are two major drawbacks to this stacking approach. The first is that since the concentration depends on a difference in the electric field strength, it is usually applied to the low conductivity sample. The other main limitation is that the sample injection is limited to about 5% of the capillary volume. Once the volume is over 5%, there will be a difference between the local EOF of the sample and the buffer, which will produce a flow pressure in the capillary and cause band broadening and a decrease in the resolution.7 In alkaloid analysis, the sensitivity enhancement of FASS is often below 30-fold due to the limited sample volume that can be injected.8–11 When the analytes are electrokinetically injected, it is called field-amplified sample injection (FASI). If the pH of the sample matrix is low enough, the EOF will be negligible during electrokinetic injection (EKI) and that will allow for a very long EKI time which is called selective exhaustive injection (SEI).12 In FASI, as the analytes can migrate more quickly into the background electrolyte solution, it is often more sensitive than FASS. Alkaloids are cyclic nitrogen containing compounds with pKa values above 5;6 they will exist in the protonated form when the pH is below their pKa values. At the same time, as the EOF is very low, a large amount of the positively charged alkaloids can be conveniently introduced using positive voltage which permits the sensitivity enhancements from several times to as high as 3 to 4 orders of magnitude.13–33 In FASI, a solvent plug is often introduced by pressure before sample introduction. The low conductivity of the solvent plug is able to produce a very high electric field in the capillary which can enhance the stacking at the interface between the solvent plug and the background electrolyte solution. Furthermore, with a solvent plug, the results are often more reproducible.16–21,24–28 In FASI, if the analytes were solved in the ACN aqueous solution, it is called ACN induced FASI.15,22 The conductivity of the sample is much lower than that of the background electrolyte (BGE) solution, resulting in enhanced electric field strength at the injection end. The analytes in the sample zone with a high migration speed can decelerate sharply at the boundary between the sample zone and the running buffer, and therefore stack at the interface between the low-conductivity sample zone and the running buffer.15,22 Zhu et al. have concentrated ephedrine and pseudoephedrine in human urine with FASS in flow injection-capillary electrophoresis (FI-CE) with a 50-fold sensitivity enhancement and achieved limits of detection (LODs) from 0.21 to 0.23 μg mL−1.16 FASI can be coupled to on-line microextraction to further enhance the sensitivity of alkaloids. Fang et al. have combined headspace solid-phase microextraction (SPME) with a sol–gel derived butyl methacrylate/silicone fiber and FASI for ephedrine derivatives.30 At the same time, more sensitive detectors such as a mass spectrometer (MS)32 and electrochemiluminescence detector (ECL)33 have also been applied to capillary electrophoretic on-line concentration to further enhance the sensitivity of alkaloids in CE analysis.
The loss in efficiency due to the presence of the sample matrix and the EOF mismatch between the sample matrix and the electrolyte can be overcome by removing the sample matrix with EOF. When anions migrate faster than the EOF, they can be stacked and separated without changing the polarity and it is called large volume sample stacking with EOF pump (LVSEP).34 If the anions migrate slower than the EOF, most of the sample matrix must be removed first by a negative EOF and then the polarity is switched to positive for separation, which is called large volume sample stacking with polarity switching (LVSSPS). As the pKa values of alkaloids are larger than 5.0 and most of them are positively charged or neutral under large pH ranges, LVSEP is not applicable for these alkaloid compounds. But for some alkaloids, such as sesquiterpene type alkaloids, they can also be negatively charged under high pH. For these compounds, they can be concentrated by LVSSPS. Cong has applied LVSSPS in microemulsion electrokinetic chromatography (MEEKC) to concentrate peritassine A, triptonine B, wilfordine, wilforgine and wilforine from Tripterygium wilfordii Hook with sensitivity enhancement from 13 to 23-fold and achieved LODs of 0.001–0.003 ng mL−1.35
2.2 Transient isotachophoresis (tITP)
In traditional ITP, sample ions are positioned between leading and terminating electrolyte ions. Upon application of the applied voltage, an electric field gradient is established over the sample and electrolyte zones with the field strength indirectly proportional to the mobility of the ion in that zone. Due to difference of conductivity between leading and terminating electrolytes, the analytes will be divided into various zones based on their different effective mobility and the length of each zone is defined by the Kohlrausch regulating function and the initial concentration of the leading ion. In order to make ITP an effective method for on-line enrichment in capillary electrophoresis, the ITP step needs to be transient ITP (tITP). In other words, the ITP system must be destroyed at a suitable time and changed to another CE mode, usually capillary zone electrophoresis, for separation.36 Feng et al. have applied tITP to concentrate 2-amino-1-phenylethanol, berberine, norephedrine, synephrine and atropine by introducing ions with a long hydrophobic chain as terminator in nonaqueous capillary electrophoresis. They have achieved 8 to 10-fold sensitivity enhancements and LODs of 0.14–0.29 mg mL−1.37 Sometimes the analytes were dissolved in acetonitrile or its solvent mixture that could act as a terminator when voltage was applied, creating a pseudo-isotachophoresis system.38
2.3 pH based stacking
In dynamic pH junction (DypH), due to the acidity of the sample zone and the different BGE, alkaloid cations pass from the sample zone (lower pH) to the BGE (higher pH), resulting in their ionization change and their migration velocity also changes which can cause them to accumulate at the boundary.39 pH-mediated stacking is a simple and useful on-column preconcentration technique for the analysis of high ionic strength samples. Acid stacking is used for cationic analytes while base stacking is used for anionic analytes. During pH-mediated stacking, the BGE consists of a salt of a weak base (base stacking) or weak acid (acid stacking). A large volume of sample injection is made before injection of the strong acid (acid stacking) or after injection of a strong base (base stacking). This results in the titration of BGE cations (base stacking) or BGE anions (acid stacking) by hydroxyl ions (base stacking) or hydronium (acid stacking) and creates a low conductivity zone where anions (base stacking) and cations (acid stacking) move faster and stack at the interface of the sample and the highly conductive background electrolyte.40 Meng et al. applied acid base stacking to concentrate morphine, codeine and 6-monoacetyl morphine in human saliva sample analysis,41 having achieved a 1000-fold sensitivity enhancement and an LOD of 7 ng mL−1. Moving reaction boundary (MRB) induced stacking is very similar to pH-mediated stacking. It can also stack analytes in a high-salt sample matrix. Wang et al. applied MRB to stack oxymatrine and matrine in rat plasma.42 In their work, a stacking buffer was introduced between the sample phase and the separation buffer, and higher than 60-fold sensitivity enhancements have been obtained. The LODs of oxymatrine and matrine were 0.26 μg mL−1 and 0.19 ng mL−1, respectively. Meng applied MRB to stack sophoridine,43 in which 40 mM HCOONa (pH 2.6) was used as the separation and stacking phase and 100 mM HCOONa (pH 10.8, adjusted with ammonia) as the sample phase, achieving a 12-fold sensitivity enhancement and an LOD of 0.492 μg mL−1.
3. Micelle based stacking
3.1 Sweeping
Sweeping is a simple on-line concentration technique which can be used for both charged and uncharged analytes. It is the picking up and accumulation of analyte molecules by the pseudostationary phase as they penetrate the sample zone.44 The mechanism relies on the interaction between the additive (pseudostationary phase) in the buffer solution and the analytes. The higher the interaction, the higher the enrichment. Wang et al. analyzed strychnine and brucine in traditional Chinese medicines with sweeping-MEKC. Around 100-fold sensitivity enhancements and LODs of 0.05–0.07 μg mL−1 have been obtained.45 Sun et al. have developed a sweeping method to improve the sensitivity of berberine, coptisine and palmatine by micellar electrokinetic chromatography (MEKC),46 achieving a 500-fold sensitivity enhancement and an LOD of 2.5 ng mL−1. Cahours et al. have developed a sweeping method based on borate complexation and a 35-fold sensitivity enhancement has been obtained.47 Sweeping-MEKC has been used to analyze papaverine, narceine, noscapine, thebaine, reticuline and oripavine in opium and poppy straw48 as well as colchicine in milk and human urine.49 At the same time, sweeping has been coupled to single drop microextraction (SDME) and hollow fiber liquid phase microextraction (HF-LPME). In human urine analysis, the sensitivity of berberine, palmatine and tetrahydropalmatine has been improved 1600–3550-fold with LODs of 0.2–1.5 ng mL−1 by SDME-sweeping.50 Also from human urine, the sensitivity of strychnine and brucine has been enhanced 35 to 50-fold with LODs of 1–2 ng mL−1 by HF-LPME-sweeping.51,52
Palmer et al. found that when the salt concentration in the sample matrix is higher than the BGE concentration, it resulted in a heterogeneous electric field through the column, wherein a lower apparent field is experienced by the sample matrix relative to the field experienced by the BGE. This field difference results in stacking of micelles on the detector side of the sample zone.53 This increased micelle concentration causes the analytes to concentrate or stack, which is called micelle stacking. Giordano et al. have studied the conditions that affect micelle stacking. They found that the enrichment strongly depends upon the relative conductivities of the sample matrix and BGE, the relative column length of the sample plug, and the mobility of the ions involved in the stacking process regardless of electric field conditions.54
3.2 Analyte focusing by micelle collapse (AFMC)
In this technique, sample molecules are transported inside micelles under an applied electric field in a micellar electrolyte solution containing an anionic surfactant and an additional anion having high electrophoretic mobility. With an increase in the concentration of the added electrolyte salt in the sample, the surfactant micelles are continuously diluted and collapsed into a liquid phase zone, thereby releasing and accumulating the transported molecule.55 Yin applied AFMC to concentrate oxymatrine and matrine in Sophora flavescens Ait and its Chinese medicine preparations with sensitivity enhancement factors from 24 to 48-fold and obtained LODs of 0.2–0.3 μg mL−1.56
3.3 Micelle to solvent stacking (MSS)
In MSS, the sample is prepared in a micellar solution without an organic solvent, and the BGE is prepared with an organic solvent or its aqueous solution. Because the organic solvent in the BGE affects the micelle interaction with the analytes, the effective electrophoretic directions of the analytes will reverse at the micelle to solvent stacking boundary (MSSB), therefore causing analyte focusing.56,57 Zhu et al. have achieved MSS stacking of ephedrine and berberine in urine by introducing a co-solvent plug (BGE solved in the methanol/water mixture) between the sample matrix and the BGE. The sensitivity of ephedrine and berberine was improved 300 to 1036-fold and their LODs were in the 0.002 to 0.003 μg mL−1 range.58 Coupling methanol assisted micelle collapse with sample matrix removal by polarity switching, this group applied MSS to NACE (MSS-NACE) for on-line preconcentration for the first time. The sensitivity of berberine and jatrorrhizine was improved 128 to 153-fold and their LODs were in the 0.5–1.1 ng mL−1 range.59 Yin et al. determined strychnine and brucine in traditional Chinese medicinal preparations by capillary zone electrophoresis with MSS concentration60 and about 40-fold sensitivity enhancements have been obtained with LODs ranging from 0.02 to 0.05 μg mL−1. Zhang et al. concentrated berberine, palmatine and jatrorrhizine in human plasma with MSS concentration and sensitivity enhancements of 47 to 53-fold and LODs of 0.01–0.02 μg mL−1 have been achieved.61
4. Combination of different on-line concentration strategies
In recent years, great attention has been paid to the combined use of the above methods to achieve further sensitivity enhancements for alkaloid analysis.
4.1 Field amplified sample injection-pseudo-isotachophoresis-acid stacking (FASI-pITP-acid stacking)
Zhang et al. have developed a FASI-pITP-acid stacking method to concentrate alkaloid cations in a high-salt sample matrix in capillary electrophoresis. Fig. 1 shows the schematic illustration of its sample injection and stacking. It was found that adding acetonitrile (70%) to a sample solution with a high-salt sample matrix not only induced field-amplified sample stacking by decreasing conductivity but also acted as a termination reagent in the succeeding pseudo-ITP. After sample injection had been completed, a plug of H+ was injected electrokinetically and a neutralization reaction between H+ and tartrate from the buffer solution produced a low conductivity zone, in which the injected analyte cations were further concentrated. With the sequential preconcentration method, a 1400-fold sensitivity increase and LODs of 0.1–0.3 ng mL−1 could be observed compared with the conventional electrokinetic injection method.38
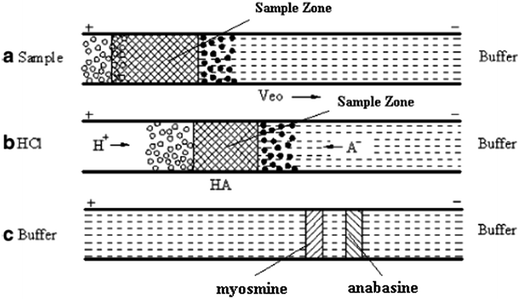 |
| Fig. 1 Schematic illustrations of field-amplified sample injection induced by acetonitrile and pseudo-isotachophoresis (ITP)-acid stacking: (a) sample injection; (b) introduction of HCl; (c) capillary zone electrophoresis separation. A− tartrate, white circles acetonitrile, black circles Na+ (modified from [ref. 38] with Springer permission). | |
4.2 Coupling sweeping and other concentration techniques
In CE analysis of alkaloids, sweeping can be coupled to many on-line stacking methods including cation-selective exhaustive injection (CSEI),12,62 dynamic pH junction (DypH),39 micelle to solvent stacking (MSS),63 field amplified sample injection (FASI)64 and large volume sample stacking (LVSS).65,66 Chiang et al. coupled CSEI and sweeping to concentrate 6 kinds of ephedra-alkaloids in MEKC analysis of Ephedra herba. The sensitivity was improved 2.64 × 104 to 4.58 × 104-fold and LODs of 3.04–32.5 ng mL−1 has been achieved.12 Huang and Hsieh62 have applied CSEI-sweeping to concentrate tobacco alkaloids in microemulsion electrokinetic chromatography analysis. Fig. 2 shows the schematic illustrations of sample injection and stacking. They have achieved 180 to 540-fold sensitivity enhancements and LODs of 0.010–0.020 μg mL−1. Yu et al. have coupled DypH to sweeping to concentrate berberine, coptisine and palmatine in Kuan Donghua samples (Fig. 3 shows its working procedures) with a 24–90-fold sensitivity enhancement and achieved an LOD as low as 30 ng mL−1.39 Yang et al. have developed an on-line sample preconcentration method by sweeping and MSS in CZE to determine strychnine and brucine in traditional Chinese herbal medicines (Fig. 4 shows its working procedures). Compared with normal CZE normal injection, 51–38-fold improvement in concentration sensitivity and an LOD of 0.01 μg mL−1 have been achieved.63 Li et al. have coupled electrostacking with sweeping in MEEKC to concentrate matrine and sophoridine in Sophora flavescens,64 achieving sensitivity enhancements of 80 to 157-fold with LODs of 1.11–2.41 ng mL−1. Zhu et al. coupled LVSS to sweeping to concentrate caffeine and theophylline in tea analysis by MEKC with enhancements of 9–18-fold and achieved LODs of 30.9–32.9 ng mL−1 (Fig. 5 shows the schematic illustrations of sample injection and stacking).65 Kosina et al. have coupled LVSS to sweeping to concentrate sanguinarine in rat hepatocytes, human gingival fibroblasts, feed, porcine faeces, body fluids and tissue analysis by MEKC and an LOD of 0.40 ng mL−1 has been achieved.66
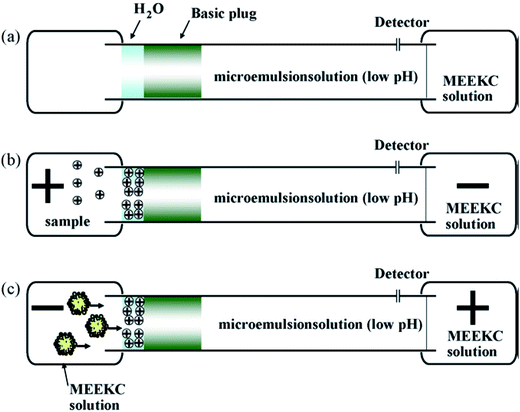 |
| Fig. 2 Schematic diagram of the CSEI-sweeping MEEKC model. (a) A capillary was conditioned with a microemulsion solution of pH 2.0. A basic plug and a short deionized water plug (0.32 mm, 50 mbar, 1 s) were then introduced hydrodynamically. (b) Electrokinetic injection for 300 s at positive polarity (+10 kV) of the analytes prepared in a phosphate solution of pH 2. (c) Microemulsion solution was placed at the inlet end of the capillary followed by the application of voltage at negative polarity (from [ref. 62] with Elsevier permission). | |
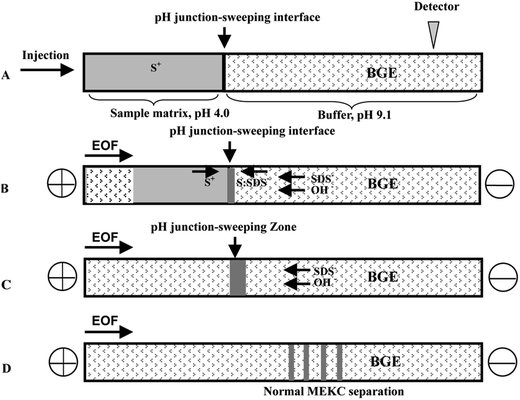 |
| Fig. 3 Schematic diagrams of the dynamic pH junction-sweeping strategy. Steps: (A) large-volume sample injection; (B) beginning of pH junction-sweeping; (C) end of pH junction-sweeping; (D) normal MEKC separation (from [ref. 39] with Wiley permission). | |
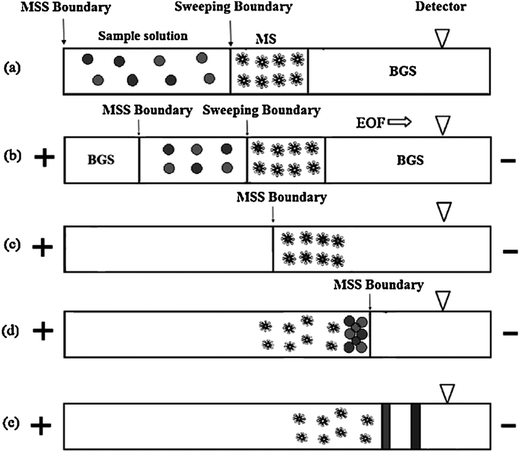 |
| Fig. 4 Illustrative diagram of sweeping-MSS in the CZE model (from[ref. 63] with Elsevier permission). | |
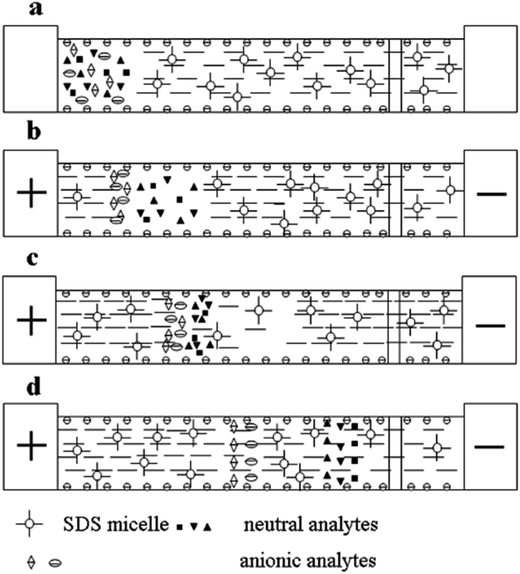 |
| Fig. 5 Schematic illustration of large volume stacking-sweeping (LVSS-sweeping): (a) after the capillary was filled with BGE, the sample plug was introduced hydrodynamically. (b) As the positive voltage was applied, anionic analytes focused at the boundary between the sample zone and BGE while neutral analytes migrated in the same direction and velocity as EOF and were not stacked. (c) Continuous application of positive voltage allowed micelles and other electrolyte ions to migrate into the sample zone. After sweeping neutral analytes, the micelles focused at the boundary that still existed between the sample zone and BGE at this point. (d) All the analytes were completely swept by micelles and were separated via MEKC (from [ref. 65] with Wiley permission). | |
5. Conclusion
In this paper, the principles and application in alkaloid analysis of different capillary electrophoretic on-line concentration techniques were discussed. With the development of CE and related technologies, great attention has been paid to the combination of different capillary electrophoretic on-line concentration technologies as well as on-line microextraction, liquid phase extraction and solid-phase extraction to acquire additional sensitivity enhancement in recent years. Furthermore, more sensitive and selective detectors such as a mass spectrometer (MS), electrochemiluminescence detector (ECL) and AD (amperometric detector) have also been used for this purpose. At the same time, analysts have begun to take advantage of CE on-line concentration techniques to achieve 2D-CE to analyze trace components in more complex samples,67 which could be the trend of CE on-line alkaloid concentration in the future.
Conflict of interest
The authors have declared no conflict of interest.
Acknowledgements
This work was supported by funding from the Science Foundation of Shandong Province (No. ZR2014JL007). The authors thank Prof. Michael C. Breadmore for his wonderful comments on the manuscript.
References
- J. Hou, G. Li, Y. Wei, H. Lu, C. Jiang, X. Zhou, F. Meng, J. Cao and J. Liu, J. Chromatogr. A, 2014, 1343, 174 CrossRef CAS PubMed.
- Q. Liu, Y. Liu, M. Guo, X. Luo and S. Yao, Talanta, 2006, 70, 202 CrossRef CAS PubMed.
- J. Chen, H. Zhao, X. Wang, F. S. C. Lee, H. Yang and L. Zheng, Electrophoresis, 2008, 29, 2135 CrossRef CAS PubMed.
- H. Guo, X. Wu, A. Wang, X. Luo, Y. Ma and M. Zhou, New J. Chem., 2015, 39, 8922 RSC.
- S. Dziomba, M. Belka, P. Kowalski, A. Plenis and T. Bączek, Biomed. Chromatogr., 2013, 27(special), 1312 CrossRef CAS PubMed.
- R. Gotti, Nat. Prod., 2013, 36, 1153 Search PubMed.
- R. L. Chien and D. S. Burgi, Anal. Chem., 1992, 64, 1046 CrossRef CAS.
- L. Sanchez-Hernandez, M. Castro-Puyana, M. L. Marina and A. L. Crego, Electrophoresis, 2011, 32, 1394 CrossRef CAS PubMed.
- L. Mercolini, R. Mandrioli, T. Trere, F. Bugamelli, A. Ferranti and M. A. Raggi, J. Sep. Sci., 2010, 33, 2520 CrossRef CAS PubMed.
- L. Zhang, R. Wang, Y. Zhang and Y. Yu, J. Sep. Sci., 2007, 30, 1357 CrossRef CAS PubMed.
- S. Kreft, J. Zel, M. Pukl, A. Umek and B. Strukelj, Phytochem. Anal., 2000, 11, 37 CrossRef CAS.
- H. Y. Chiang and S. J. Sheu, Electrophoresis, 2004, 25, 670 CrossRef CAS PubMed.
-
S. Ye, Study on the analytical methods for Sophora flavescens Ait, Panax notoginseng and its preparation by capillary electrophoresis with on-line concentration techniques, Thesis for Master's Degree, Zhejiang University, 2007.
- X. Chen, Y. Tang, S. Wang, Y. Song, F. Tang and X. Wu, Electrophoresis, 2015, 36, 1953 CrossRef CAS PubMed.
- J. Wen, W. T. Zhang, W. Q. Cao, J. Li, F. Y. Gao, N. Yang and G. R. Fan, Molecules, 2014, 19, 4907 CrossRef PubMed.
- H. Zhu, W. Liu, H. Li, Y. Ma, S. Hu, H. Chen and X. Chen, Analyst, 2011, 136, 1322 RSC.
- H. Wang, Y. Lu, J. Chen, J. Li and S. Liu, J. Pharm. Biomed. Anal., 2012, 58, 146 CrossRef CAS PubMed.
- J. Li and Y. Jiang, Zhongguo Zhongyao Zazhi, 2008, 33, 1684 Search PubMed.
- C. Li and Y. Jiang, Zhongguo Zhongyao Zazhi, 2010, 35, 3287 CAS.
- W. Gao, S. Lin, Y. Chen, A. Chen, Y. Li, X. Chen and Z. Hu, J. Sep. Sci., 2005, 28, 639 CrossRef CAS PubMed.
- L. Li, K. Sun, M. Wu, Y. Luo and D. Hu, Fenxi Kexue Xuebao, 2013, 29, 154 CAS.
- L. S. Yu, X. Q. Xu, L. Huang, J. M. Lin and G. N. Chen, Electrophoresis, 2009, 30, 661 CrossRef CAS PubMed.
- L. Yu, X. Xu, L. Huang, J. Lin and G. Chen, J. Chromatogr. A, 2008, 1198, 220 CrossRef PubMed.
- L. Fan, Y. Cheng, Y. Li, H. Chen, X. Chen and Z. Hu, Electrophoresis, 2005, 26, 4345 CrossRef CAS PubMed.
- S. W. Sun and H. M. Tseng, J. Pharm. Biomed. Anal., 2004, 36, 43 CrossRef CAS PubMed.
- J. Zhang, S. Wang, X. Chen, Z. Hu and X. Ma, Anal. Bioanal. Chem., 2003, 376, 210 CrossRef CAS PubMed.
- X. Hu, S. Cui and J. Q. Liu, Chromatographia, 2010, 72, 993 CAS.
- S. Liu, Q. Li, X. Chen and Z. Hu, Electrophoresis, 2002, 23, 3392 CrossRef CAS PubMed.
- B. Chen and Q. Xu, Analyst, 2011, 136, 4846 RSC.
- H. Fang, M. Liu and Z. Zeng, Talanta, 2006, 68, 979 CrossRef CAS PubMed.
- H. Ye, L. Yu, X. Xu, C. Zheng, W. Lin, X. Liu and G. Chen, Electrophoresis, 2010, 31, 2049 CrossRef CAS PubMed.
- S. Wang, H. Qu and Y. Cheng, Electrophoresis, 2007, 28, 1399 CrossRef CAS PubMed.
- Q. Xiang, Y. Gao, B. Han, J. Li, Y. Xu and J. Yin, Luminescence, 2013, 28, 50 CrossRef CAS PubMed.
- T. Tábi, K. Magyar and É. Szökő, Electrophoresis, 2005, 26, 1940 CrossRef PubMed.
-
R. Cong, Application of MEEKC and MEKC for determination of active components of Tripterygium wilfordii Hook f and some enantiomers of traditional Chinese medicine, Thesis for Master's Degree, Fujian University of Traditional Chinese Medicine, 2014.
- P. Britz-Mckbbin, G. M. Bebault and Y. Chen, Anal. Chem., 2000, 72, 1729 CrossRef.
- H. T. Feng, W. S. Low, L. J. Yu and S. F. Y. Li, Chromatographia, 2006, 63, 513 CAS.
- Z. Zhang, X. Zhang, J. Wang and S. Zhang, Anal. Bioanal. Chem., 2008, 390, 1645 CrossRef CAS PubMed.
- L. Yu and S. F. Y. Li, Electrophoresis, 2005, 26, 4360 CrossRef CAS PubMed.
- M. E. Hoque, S. D. Arnet and C. E. Lunte, J. Chromatogr. B, 2005, 827, 51 CrossRef CAS PubMed.
- P. Meng, Y. Wang and L. Meng, Anal. Methods, 2012, 4, 3695 RSC.
- X. Wang, W. Zhang, L. Fan, B. Hao, A. Ma, C. Cao and Y. Wang, Anal. Chim. Acta, 2007, 594, 290 CrossRef CAS PubMed.
-
J. Meng, Model study and alkaloid detection based on moving reaction boundary, Thesis of Master's Degree, Shanghai Jiao Tong University, 2008.
- J. P. Quirino and S. Terabe, Anal. Chem., 1999, 71, 1638 CrossRef CAS.
- C. Wang, D. Han, Z. Wang, X. Zang and Q. Wu, Anal. Chim. Acta, 2006, 572, 190 CrossRef CAS PubMed.
- S. Sun and H. Tseng, J. Pharm. Biomed. Anal., 2005, 37, 39 CrossRef CAS PubMed.
- X. Cahours, Y. Daali, S. Cherkaoui and J. L. Veuthey, Chromatographia, 2002, 55, 211 CAS.
- R. G. Reid, D. G. Durham, S. P. Boyle, A. S. Low and J. Wangboonskul, Anal. Chim. Acta, 2007, 605, 20 CrossRef CAS PubMed.
- E. Bodoki, B. C. Iacob and R. Oprean, Croat. Chem. Acta, 2011, 84, 383 CrossRef CAS.
- W. Gao, G. Chen, Y. Chen, N. Li, T. Chen and Z. Hu, J. Chromatogr. A, 2011, 1218, 5712 CrossRef CAS PubMed.
- C. Wang, C. Li, X. Zang, D. Han, Z. Liu and Z. Wang, J. Chromatogr. A, 2007, 1143, 270 CrossRef CAS PubMed.
- X. H. Zang, C. R. Li, Q. H. Wu, C. Wang, D. D. Han and Z. Wang, Chin. Chem. Lett., 2007, 18, 316 CrossRef CAS.
- J. Palmer, N. J. Munro and J. P. Landers, Anal. Chem., 1999, 71, 1679 CrossRef CAS PubMed.
- B. C. Giordano, C. I. D. Newman, P. M. Federowicz, G. ECollins and D. S. Burgi, Anal. Chem., 2007, 79, 6287 CrossRef CAS PubMed.
- J. P. Quirino and P. R. Haddad, Anal. Chem., 2008, 80, 6824 CrossRef CAS PubMed.
-
X. Yin, Application of On-line Concentration of Pesticides Residues and Pharmaceuticals by Capillary Electrophoresis, Thesis for Master's Degree, Agricultural University of Hebei, 2011.
- J. P. Quirino, J. Chromatogr. A, 2009, 1216, 294 CrossRef CAS PubMed.
- H. Zhu, W. Lü, H. Li, Y. Ma, S. Hu, H. Chen and X. Chen, J. Chromatogr. A, 2011, 1218, 5867 CrossRef CAS PubMed.
- H. Zhu, C. Ren, S. Hu, X. Zhou, H. Chen and X. Chen, J. Chromatogr. A, 2011, 1218, 733 CrossRef CAS PubMed.
- X. F. Yin, Z. Li, S. H. Zhang, C. X. Wu, C. Wang and Z. Wang, Chin. Chem. Lett., 2011, 22, 330 CrossRef CAS.
- S. Zhang, R. Ma, X. Yang, C. Wang and Z. Wang, J. Chromatogr. B, 2012, 906, 41 CrossRef CAS PubMed.
- H. Y. Huang and S. H. Hsieh, J. Chromatogr. A, 2007, 1164, 313 CrossRef CAS PubMed.
- X. Yang, S. Zhang, J. Wang, C. Wang and Z. Wang, Anal. Chim. Acta, 2014, 814, 63 CrossRef CAS PubMed.
- L. Li, D. Hu, W. Gao, Y. Li and H. Kong, Fenxi Ceshi Xuebao, 2011, 30, 678 CAS.
- Z. Zhu, N. Yan, X. Zhou, L. Zhou and X. Chen, J. Sep. Sci., 2009, 32, 3481 CrossRef CAS PubMed.
- P. Kosina, J. Sevcik, M. Modriansky, A. Gavenda, P. Bednar, P. Barktak, D. Walterova and J. Ulrichova, J. Sep. Sci., 2003, 26, 679 CrossRef CAS.
- Z. Zhang, X. Du and X. Li, Anal. Chem., 2011, 83, 1291 CrossRef CAS PubMed.
Footnote |
† Professor X. Zhang and Professor Y. Lu have contributed equally to this work. |
|
This journal is © The Royal Society of Chemistry 2017 |
Click here to see how this site uses Cookies. View our privacy policy here.