DOI:
10.1039/C6MT00128A
(Paper)
Metallomics, 2017,
9, 61-68
Comparison of the metabolism of inorganic and organic selenium species between two selenium accumulator plants, garlic and Indian mustard†
Received
7th June 2016
, Accepted 8th September 2016
First published on 8th September 2016
Abstract
The metabolism of selenomethionine (SeMet) in two major selenium (Se) accumulator plants, garlic and Indian mustard, was compared to that of stable isotope labeled selenate. Indian mustard more efficiently transported Se from roots to leaves than garlic. In addition, Indian mustard accumulated larger amounts of Se than garlic. γ-Glutamyl-Se-methylselenocysteine (γ-GluMeSeCys) and Se-methylselenocysteine (MeSeCys) were the common metabolites of selenate and SeMet in garlic and Indian mustard. Indian mustard had a specific metabolic pathway to selenohomolanthionine (SeHLan) from both inorganic and organic Se species. SeMet was a more effective fertilizer for cultivating Se-enriched plants than selenate in terms of the production of selenoamino acids.
Significance to metallomics
Selenium (Se) is an essential element in animals but not plants. Nevertheless, Se metabolism in plants is an exciting topic because Se metabolites in plants are beneficial to human health. Considering the environmental circulation of Se, plants may be exposed to not only inorganic but also organic Se species, particularly, selenomethionine (SeMet), a naturally occurring selenoamino acid. In this study, the metabolism of SeMet in two major Se accumulator plants, garlic and Indian mustard, was compared to that of selenate. As a result, we clearly showed the differences in Se metabolism between SeMet and selenate in the plants.
|
Introduction
Selenium (Se) is a trace essential element in animals, and 25 genes encoding selenoproteins have been found in the human genome.1 It has been proposed that Se reduces the risk of cancer and cardiovascular diseases.2,3 Meat and seafood are the main sources of Se. However, some vegetables can also be appropriate Se sources. Although Se is not an essential element in plants, plants in the Liliaceae family, such as garlic, onion, and leek, and the Brassicaceae family, such as Indian mustard, are well-known Se accumulators.4 These plants have a large metabolic capacity for sulfur. As Se shares the same metabolic pathway with sulfur, which belongs to the same group in the periodic table as Se, the plants can produce several kinds of Se-containing amino acids (selenoamino acids) as secondary metabolites. For instance, Se-methylselenocysteine (MeSeCys), γ-glutamyl-Se-methylselenocysteine (γ-GluMeSeCys), and selenohomolanthionine (SeHLan) are produced in the plants via the sulfur assimilation pathway.5–7 These selenoamino acids are reported to be effective against tumors and sepsis.8,9 Therefore, selenized vegetables can function as chemopreventive or medicinal foods.
In a plethora of past studies, inorganic Se compounds, such as selenate and selenite, have been used for growing selenized vegetables because massive amounts of inorganic Se compounds are more easily available than organic Se compounds for the purpose of fortification.10,11 Microorganisms, fungi in particular, are well known Se accumulators.12,13 Contrary to Se accumulator plants, fungi accumulate Se as selenomethionine (SeMet).12,14 Hence, it can be assumed that plants are exposed to not only inorganic but also organic Se species in the ecosystem.
The bioavailability as well as the pharmacological and toxicological effects of Se in animals markedly depends on the chemical form of the ingested Se. In plants, Se metabolism could also depend on the chemical form of the fortified Se. Indeed, some differences in absorption, translocation, and metabolism by chemical form were reported.15–17 However, those differences were evaluated between inorganic species, i.e., selenite and selenate. At present, very few reports addressing Se metabolism in plants fortified with organic Se species are available.18
In this study, we aimed to reveal Se metabolism in two Se accumulator plants, Allium sativum (garlic) and Brassica juncea (Indian mustard), exposed to SeMet. We used stable isotope [76Se]-labeled selenate for simultaneous exposure with non-labeled SeMet. This experimental design allowed us to discuss the metabolism of SeMet in the plants by precise comparison to the metabolism of selenate. We also discussed differences in Se metabolism between garlic and Indian mustard.
Materials and methods
Chemicals
Sodium selenate, glutathione reduced form (GSH), nitric acid, and ammonium acetate were purchased from Wako (Osaka, Japan). Se-L-methylselenocysteine (MeSeCys), L-selenomethionine (SeMet), and γ-glutamyl-Se-L-methylselenocysteine (γ-GluMeSeCys) were purchased from Acros Organics (Geel, Belgium), Sigma (St. Louis, MO, USA), and Pharmase (Austin, TX, USA), respectively. Selenohomolanthionine (SeHLan) was synthesized in our laboratory using a previously reported method.6 The metallic form of 76Se (99.9% enriched) was purchased from Isoflex USA (San Francisco, CA, USA). 76Se-enriched selenate was prepared by oxidation of 76Se-enriched metallic Se. Metallic Se was oxidized by dissolving it in concentrated metal-free nitric acid containing hydrogen peroxide (H2O2
:
conc. HNO3, 4
:
1 v/v) and subsequently neutralizing it with 1 M sodium hydroxide. Otsuka salt mixture-A for the preparation of cultivation medium was purchased from Otsuka Agri Techno Co., Ltd (Tokyo, Japan). Deuterium gas (D2, >95%) was purchased from Showa Denko (Tokyo, Japan). All reagents used in this study were of the highest or analytical grade. Deionized water (18.3 MΩ cm) was used throughout.
Plant growth and sample preparation
Brassica juncea (Indian mustard) seeds and Allium sativum (garlic) bulbs were purchased from Sakata (Kanagawa, Japan) and Sawada Farm, Inc. (Aomori, Japan), respectively. The seeds and bulbs were germinated and hydroponically grown in cultivation medium in a growth chamber (LH-55-RDS; Nihonikakikai, Osaka, Japan) with a photoperiod of 14 h light (8000 lux)/10 h dark at 25.0 °C for 30 and 14 days for the Indian mustard and garlic, respectively. The cultivation medium was sterilized prior to use. Then, the plants were exposed to medium containing 76Se-enriched selenate and SeMet at a concentration of 30 μmol L−1 for 7 days. After the exposure, the plants were immediately harvested, gently washed with deionized water, and divided into roots, leaves, and bulbs (garlic only). Each part was lyophilized and milled to obtain homogenized powder. A 0.1 g portion of the lyophilized root powder was incubated in 1.0 mL of deionized water for 1 h at room temperature. Water extracts were obtained by ultracentrifugation of the mixture at 105
000g for 60 min.
Determination of Se concentration
The lyophilized root powder (40 mg) and the water extract (100 μL) were wet-ashed with 200 μL of nitric acid overnight. The ashed samples were diluted with deionized water, and the Se concentration in each sample was determined using ICP-MS (Agilent 7500ce, Agilent Technologies, Hachioji, Japan) equipped with an octopole reaction system (ORS) at m/z 76 and 77, with m/z 128 for tellurium as the internal standard. D2 gas was used at a flow rate of 2.0 mL min−1.19 Mass calibration and parameter optimization for ICP-MS were performed daily using a tuning solution containing 10 ng mL−1 each of lithium, yttrium, cerium, and thallium (Agilent Technologies) prior to use. The concentration of each Se isotope was determined using an external calibration method. The concentration of Se originating from selenate was calculated from the signal intensity obtained by the following equation: [signal intensity of Se originating from selenate] = [total signal intensities of 76Se] – [signal intensity of 77Se from SeMet] × (0.0936/0.0763), whereas the concentration of Se originating from SeMet was calculated from the signal intensity at m/z 77. To validate our Se measurement, dogfish muscle (DORM2, National Research Council, Ottawa, Canada) was used as the standard reference material (SRM). We obtained 102.9 ± 8.6% to the certified value of the SRM.
Data are presented as means ± S.D. Statistical analysis included the one-way analysis of variance followed by the Student's t-test. The level of significance was set at p < 0.05 and is indicated by an asterisk (*).
Speciation analysis of Se in the sample using HPLC-ICP-MS
A 20 μL aliquot of the water extract and 1.0 μg Se mL−1 of the standard solution of selenate, SeMet, MeSeCys, SeHLan, and γ-GluMeSeCys were applied to HPLC coupled with ICP-MS to analyze the distribution of Se. The HPLC system (Prominence, Shimadzu, Kyoto, Japan) consisted of an on-line degasser, an HPLC pump, a Rheodyne six-port injector, and a column. The eluate was directly introduced into the nebulizer of the ICP-MS to detect Se. A multi-mode size exclusion column (Shodex Asahipak GS-320HQ, 7.5 i.d. × 300 mm with a guard column, 7.5 i.d. × 75 mm, Showa Denko, Tokyo) was mainly used in this study. This column was eluted with 50 mM ammonium acetate, pH 6.5, at a flow rate of 0.6 mL min−1. A sample of plant exposed to 76Se-enriched selenate was detected at m/z 76 and 77 under the D2 reaction mode, and the elution profile of Se originating in 76Se-enriched selenate was depicted using the calculation mentioned above. A sample of plant exposed to SeMet was detected at m/z 82 without a reaction/collision mode. Triplicate data of the Se distribution in the extracts were obtained, and a typical value of them is shown.
Results and discussion
Comparison of Se accumulation in garlic and Indian mustard exposed to selenate and SeMet
Se concentration in the roots was far higher than the concentration in other parts of the plants, such as in the bulbs and leaves, and there were no apparent differences between inorganic (selenate) and organic (SeMet) Se concentrations in the roots, bulbs, and leaves of garlic (Fig. 1a). On the other hand, the difference in Se concentrations between the roots and leaves in Indian mustard was not as large as that observed in garlic. The concentration of Se originating from SeMet was significantly higher than that originating from selenate in the roots, but tended to be lower than that originating from selenate in the leaves (Fig. 1b). Regardless of the plant part, Se concentrations in Indian mustard were higher than those in garlic. Taking biomass into consideration, Se was found to accumulate more in Indian mustard than in garlic (Table 1). These results indicate that Indian mustard has a larger accumulation capacity for Se than garlic. In particular, the leaf accumulation capacity for Se is larger in Indian mustard than in garlic. However, the accumulation capacity for Se was species-specific in Indian mustard, that is, SeMet was more preferably accumulated in the roots than selenate. It was reported that selenate was incorporated via sulfate transporter(s),20,21 and SeMet was incorporated via amino acid transporter(s).22,23 It is reported that Se is transformed into volatile species, such as dimethyldiselenide and dimethylselenide, in Se accumulator plants.24,25 SeMet is efficiently transformed into volatile Se species in the leaves; thus, the Se concentration originating from SeMet is higher than that from selenate in Indian mustard. In addition to the accumulation capacity for Se, the metabolism of selenate and SeMet in garlic and Indian mustard was also evaluated using speciation analyses.
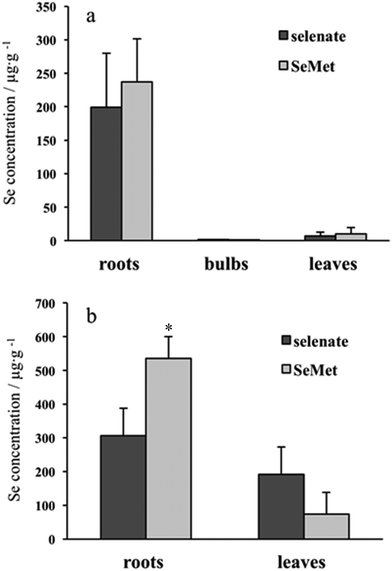 |
| Fig. 1 Se concentrations in roots, bulbs, and leaves of garlic and Indian mustard exposed to [76Se]-enriched selenate and SeMet. Garlic (a) and Indian mustard (b) were cultivated in medium containing [76Se]-enriched selenate and SeMet at a concentration of 30 μM for 7 days. Columns and bars indicate means ± standard deviations for 3 samples. | |
Table 1 Se amounts in garlic and Indian mustard exposed to [76Se]-enriched selenate and SeMet
|
Se amount (μg) |
Garlic |
Indian mustard |
Garlic and Indian mustard were cultivated in medium containing [76Se]-enriched selenate and SeMet at a concentration of 30 μM for 7 days. Values are means ± standard deviations for 3 samples. |
Selenate origin |
35 ± 18 |
208 ± 10 |
SeMet origin |
42 ± 18 |
113 ± 1.0 |
Metabolism of Se from selenate and SeMet in garlic and Indian mustard
Three apparent peaks of Se originating from SeMet were detected in the water extract of the garlic leaves at the retention times of 16.7 (2), 19.9 (4), and 20.9 (5) min (Fig. 2a). The retention times matched those of the authentic standards Se-methylselenocysteine (MeSeCys), and SeMet. The structures and retention times of γ-glutamyl-Se-methylselenocysteine (γ-GluMeSeCys) found in garlic were confirmed using electrospray ionization mass spectrometry (ESI-MS) in our previous study.7 Three peaks of Se originating from selenate were detected at the retention times of 15.6 (1), 16.7 (2), and 19.9 (4) min (Fig. 2b). The peak at the retention time of 15.6 min corresponded to selenate; this was determined on the basis of the retention time that matched that of the selenate authentic standard. The elution profiles of the authentic standards of selenate, MeSeCys, SeMet and SeHLan are shown in Fig. S1 (ESI†). Hence, SeMet and selenate were commonly metabolized to γ-GluMeSeCys and MeSeCys. Contrary to garlic, an additional peak of Se originating from both SeMet and selenate was detected in the water extracts of Indian mustard leaves at a retention time of 18.0 (3) min (Fig. 2c and d). The peak was assigned to selenohomolanthionine (SeHLan) because its retention time matched that of the SeHLan authentic standard (Fig. S1, ESI†), and the structure of SeHLan found in a Brassicaceae family plant was confirmed using ESI-MS in our previous work.6 SeHLan is a specific Se metabolite of Indian mustard and can be biosynthesized from both organic (SeMet) and inorganic (selenate) Se compounds. SeHLan was firstly identified in pungent radish, Raphanus sativus, a Brassicaceae family plant.6 This suggests that Indian mustard shows a specific metabolic pattern to SeHLan from SeMet and selenate. It is speculated that SeMet is demethylated to form selenohomocysteine (SeHCys), and SeHCys is reacted with O-acetylhomoserine for the biosynthesis of SeHLan.26 In our previous work, we found that a Se-methylselenyl moiety (CH3Se-) was firstly cleaved from SeMet and then conjugated with glutathionine (GSH) to form GSSeCH3 in another Se accumulator Brassicaceae family plant, Brassica rapa var. perviridis.18 As an alternative pathway, SeMet is transformed into MeSeCys via selenohomocysteine (SeHCys), selenocystathionine, and selenocysteine (SeCys). This pathway is known as the reverse transselenation pathway.26 In this pathway, γ-GluMeSeCys is recognized as the final metabolite. Contrary to that pathway, S-alkylcysteine is transformed from Cys via GSH and γ-GluCys via sulfur metabolism,27 and Se can share the same metabolic pathway with sulfur. If Se is metabolized in the pathway, MeSeCys is the final metabolite. At present, it is difficult to decide which metabolite, γ-GluMeSeCys or MeSeCys, is the final metabolite of Se in the plants. The peak corresponding to selenate was detected in Indian mustard at m/z 76 (Fig. 2c). This seems to have originated not from SeMet but from [76Se]-selenate contaminating [77Se]-selenate. The enrichment of [76Se]-selenate used in this study was 99.9%. Because the signal intensity of selenate at m/z 76 was one thousand times higher than that at m/z 77, the peak of selenate at m/z 77 could originate from the contaminants (0.01%) of [76Se]-selenate.
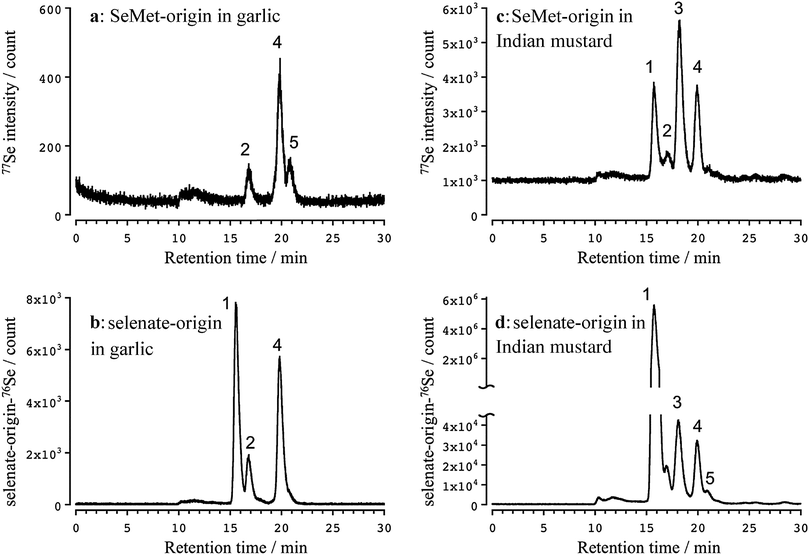 |
| Fig. 2 Elution profiles of Se in the water extracts of garlic and Indian mustard leaves exposed to [76Se]-enriched selenate and SeMet. Garlic (a and b) and Indian mustard (c and d) were hydroponically cultivated and then exposed to [76Se]-enriched selenate and SeMet at a concentration of 30 μM for 7 days. A 20 μL aliquot of the water extract of the leaves were applied to HPLC equipped with a multi-mode size exclusion HPLC column (GS-320HQ). The column was eluted with 50 mM ammonium acetate, pH 6.5, at a flow rate of 0.6 mL min−1. The eluate was directly introduced into the nebulizer of the ICP-MS to detect Se at m/z 76 and 77 for monitoring of the Se originating in selenate (b and d) and SeMet (a and c), respectively, in the D2 reaction mode. 1: selenate, 2: γ-GluMeSeCys, 3: SeHLan, 4: MeSeCys, 5: SeMet. | |
Although Se concentrations in the roots were higher than those in the leaves, the metabolisms quite resembled each other except for the amount of γ-GluMeSeCys (Fig. 3), namely MeSeCys and γ-GluMeSeCys originating from SeMet and selenate detected in garlic and Indian mustard. The relative amount of γ-GluMeSeCys to that of MeSeCys in the roots was smaller than that in the leaves. In addition, SeHLan was specifically detected in the water extracts of Indian mustard roots (Fig. 3c and d). The peak corresponding to SeMet was detected in the water extracts of Indian mustard roots at m/z 77. These indicate that selenate is metabolized into SeCys, and a part of SeCys is transformed into metabolic intermediates, such as selenocystathionine and SeHCys, via the forward transselenation pathway. Then, SeHCys is converted into SeHLan and SeMet. The forward transselenation pathway could be specific to Indian mustard.
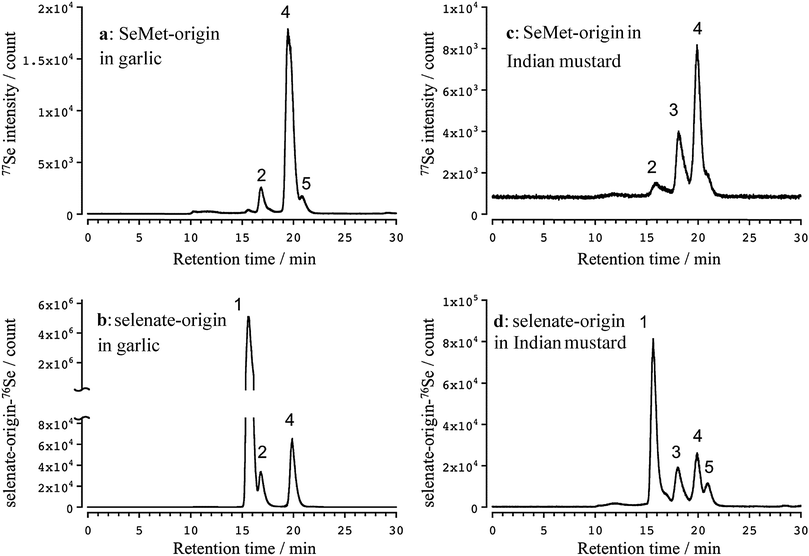 |
| Fig. 3 Elution profiles of Se in the water extracts of the garlic and Indian mustard roots exposed to [76Se]-enriched selenate and SeMet. Garlic (a and b) and Indian mustard (c and d) were hydroponically cultivated and then exposed to [76Se]-enriched selenate and SeMet at a concentration of 30 μM for 7 days. A 20 μL aliquot of the water extract of the roots were applied to HPLC equipped with a multi-mode size exclusion HPLC column (GS-320HQ). The column was eluted with 50 mM ammonium acetate, pH 6.5, at a flow rate of 0.6 mL min−1. The eluate was directly introduced into the nebulizer of the ICP-MS to detect Se at m/z 76 and 77 for monitoring of the Se originating in selenate (b and d) and SeMet (a and c), respectively, in the D2 reaction mode. 1: selenate, 2: γ-GluMeSeCys, 3: SeHLan, 4: MeSeCys, 5: SeMet. | |
SeMet was more efficiently metabolized into selenoamino acid derivatives, such as γ-GluMeSeCys, MeSeCys, and SeHLan, than selenate in garlic and Indian mustard. It has been reported that γ-GluMeSeCys and MeSeCys have anticarcinogenic and antitumor activities.2 SeMet was also expected to be an anticarcinogenic agent; an intervention study, however, revealed that the effects of SeMet were ambiguous.28 A source of SeMet supplement is biologically synthesized in yeast. The results of this study show that pharmaceutically valuable Se species, such as γ-GluMeSeCys and MeSeCys, are efficiently biosynthesized in Se accumulator plants fortified with SeMet.
Selenate concentration in the cultivation medium was decreased after 7 days due to absorption by the plant (Fig. 4a and b). No SeMet was detected in the cultivation medium after 7 days, although two peaks were detected at retention times of 15.6 (1) and 17.1 min (Fig. 4c and d). Although the former one was assignable to selenate by its chromatographic behavior, the latter one was not identified. We also evaluated the stability of SeMet in the cultivation medium without any plants under the cultivation conditions. SeMet was stable for 7 days under the cultivation conditions (data not shown). This suggests that SeMet is not decomposed chemically or biologically by microorganisms in the cultivation medium, namely, selenate and the unidentified Se compound seem to be the products of SeMet degradation by the roots of Indian mustard or Se excreted by the roots. By clarifying the unknown Se metabolite, the precise metabolism of Se would be revealed.
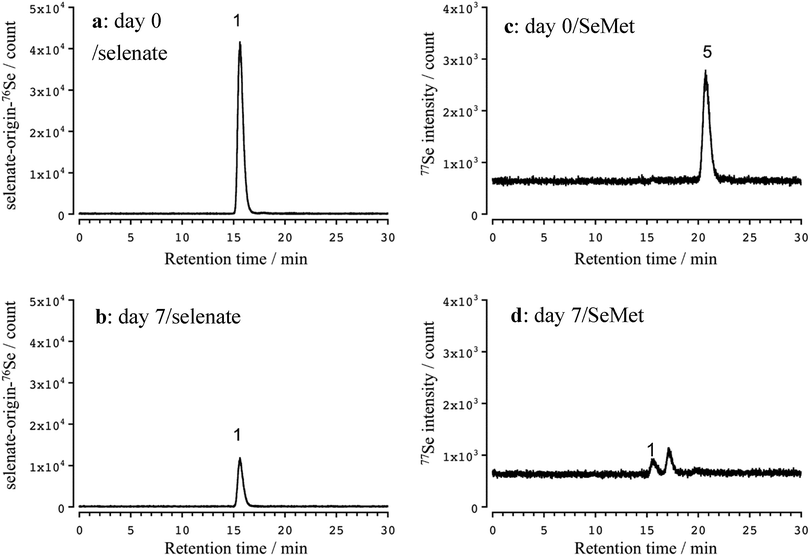 |
| Fig. 4 Time-dependent changes in the elution profiles of Se in a cultivation medium containing [76Se]-enriched selenate and SeMet. A small portion of the cultivation medium of Indian mustard was collected on day 0 (a and c) and day 7 (b and d). A 20 μL aliquot of the medium containing [76Se]-enriched selenate and SeMet was applied to HPLC equipped with a multi-mode size exclusion HPLC column (GS-320HQ). The column was eluted with 50 mM ammonium acetate, pH 6.5, at a flow rate of 0.6 mL min−1. The eluate was directly introduced into the nebulizer of the ICP-MS to detect Se at m/z 76 and 77 for monitoring of the Se originating in selenate (a and b) and SeMet (c and d), respectively, in the D2 reaction mode. 1: selenate, 5: SeMet. | |
In conclusion, two major Se accumulator plants showed different Se accumulation characteristics. The proposed metabolic pathway is depicted in Fig. 5. Indian mustard more efficiently transported Se from the roots to the leaves than garlic. In addition, Indian mustard accumulated larger amounts of Se than garlic. γ-GluMeSeCys and MeSeCys were the common metabolites of selenate and SeMet in both garlic and Indian mustard. Indian mustard showed a specific metabolic pattern to SeHLan from both inorganic and organic Se species. SeMet is a more effective fertilizer for cultivating Se-enriched plants than selenate in terms of the production of selenoamino acids.
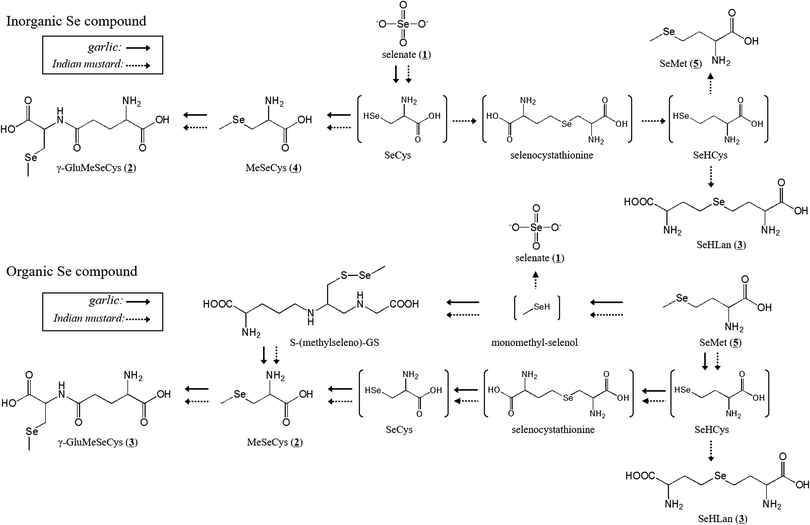 |
| Fig. 5 Proposed metabolic pathways of SeMet and selenate in garlic and Indian mustard. | |
Funding
This study was supported by JSPS KAKENHI Grant Numbers 25870739, 26293030, 15K14991 and 16H05812.
Competing financial interest
The authors declare no competing financial interest.
References
- V. N. Gladyshev and D. L. Hatfield, Selenocysteine-containing proteins in mammals, J. Biomed. Sci., 1999, 6, 151–160 CrossRef CAS PubMed.
- C. Ip, C. Hayes, R. M. Budnick and H. E. Ganther, Chemical form of selenium, critical metabolites, and cancer prevention, Cancer Res., 1991, 51, 595–600 CAS.
- C. Benstoem, A. Goetzenich, S. Kraemer, S. Borosch, W. Manzanares, G. Hardy and C. Stoppe, Selenium and its supplementation in cardiovascular disease–what do we know?, Nutrients, 2015, 7, 3094–3118 CrossRef CAS PubMed.
- P. C. Uden, H. T. Boakye, C. Kahakachchi and J. F. Tyson, Selective detection and identification of Se containing compounds – review and recent developments, J. Chromatogr. A, 2004, 1050, 85–93 CrossRef CAS PubMed.
- Y. Dong, H. E. Ganther, C. Stewart and C. Ip, Identification of molecular targets associated with selenium-induced growth inhibition in human breast cells using cDNA microarrays, Cancer Res., 2002, 62, 708–714 CAS.
- Y. Ogra, T. Kitaguchi, K. Ishiwata, N. Suzuki, Y. Iwashita and K. T. Suzuki, Identification of selenohomolanthionine in selenium-enriched Japanese pungent radish, J. Anal. At. Spectrom., 2007, 22, 1390–1396 RSC.
- Y. Ogra, K. Ishiwata, Y. Iwashita and K. T. Suzuki, Simultaneous speciation of selenium and sulfur species in selenized odorless garlic (Allium sativum L. Shiro) and shallot (Allium ascalonicum) by HPLC-inductively coupled plasma-(octopole reaction system)-mass spectrometry and electrospray ionization-tandem mass spectrometry, J. Chromatogr. A, 2005, 1093, 118–125 CrossRef CAS PubMed.
- P. D. Whanger, Selenium and its relationship to cancer: an update, Br. J. Nutr., 2004, 91, 11–28 CrossRef CAS PubMed.
- M. W. Angstwurm, L. Engelmann, T. Zimmermann, C. Lehmann, C. H. Spes, P. Abel, R. Strauss, A. Meier-Hellmann, R. Insel, J. Radke, J. Schüttler and R. Gärtner, Selenium in Intensive Care (SIC): results of a prospective randomized, placebo-controlled, multiple-center study in patients with severe systemic inflammatory response syndrome, sepsis, and septic shock, Crit. Care Med., 2007, 35, 118–126 CrossRef CAS PubMed.
- T. C. Seo, J. E. Spallholz, H. K. Yun and S. W. Kim, Selenium-enriched garlic and cabbage as a dietary selenium source for broilers, J. Med. Food, 2008, 11, 687–692 CrossRef CAS PubMed.
- T. Tsuneyoshi, J. Yoshida and T. Sasaoka, Hydroponic cultivation offers a practical means of producing selenium-enriched garlic, J. Nutr., 2006, 136, 870S–872S CAS.
- V. Díaz Huerta, A. Sanz-Medel and M. L. Fernández Sánchez, An attempt to differentiate HPLC-ICP-MS selenium speciation in natural and selenised Agaricus mushrooms using different species extraction procedures, Anal. Bioanal. Chem., 2006, 384, 902–907 CrossRef PubMed.
- J. Falandysz, Selenium in edible mushrooms, J. Environ. Sci. Health, Part C: Environ. Carcinog. Ecotoxicol. Rev., 2008, 26, 256–299 CrossRef CAS PubMed.
- V. Gergely, K. M. Kubachka, S. Mounicou, P. Fodor and J. A. Caruso, Selenium speciation in Agaricus bisporus and Lentinula edodes mushroom proteins using multi-dimensional chromatography coupled to inductively coupled plasma mass spectrometry, J. Chromatogr. A, 2006, 1101, 94–102 CrossRef CAS PubMed.
- Q. Huang, Y. Yu, Q. Wang, Z. Luo, R. Jiang and H. Li, Uptake kinetics and translocation of selenite and selenate as affected by iron plaque on root surfaces of rice seedlings, Planta, 2015, 241, 907–916 CrossRef CAS PubMed.
- J. Li, D. Liang, S. Qin, P. Feng and X. Wu, Effects of selenite and selenate application on growth and shoot selenium accumulation of pak choi (Brassica chinensis L.) during successive planting conditions, Environ. Sci. Pollut. Res. Int., 2015, 22, 11076–11086 CrossRef CAS PubMed.
- M. Longchamp, M. Castrec-Rouelle, P. Biron and T. Bariac, Variations in the accumulation, localization and rate of metabolization of selenium in mature Zea mays plants supplied with selenite or selenate, Food Chem., 2015, 182, 128–135 CrossRef CAS PubMed.
- Y. Ogra, A. Katayama, Y. Ogihara, A. Yawata and Y. Anan, Analysis of animal and plant selenometabolites in roots of a selenium accumulator, Brassica rapa var. peruviridis, by speciation, Metallomics, 2013, 5, 429–436 RSC.
- Y. Ogra, K. Ishiwata and K. T. Suzuki, Effects of deuterium in octopole reaction and collision cell ICP-MS on detection of selenium in extracellular fluids, Anal. Chim. Acta, 2005, 554, 123–129 CrossRef CAS.
- E. El Kassis, N. Cathala, H. Rouached, P. Fourcroy, P. Berthomieu, N. Terry and J. C. Davidian, Characterization of a selenate-resistant Arabidopsis mutant. Root growth as a potential target for selenate toxicity, Plant Physiol., 2007, 143, 1231–1241 CrossRef CAS PubMed.
- Y. Ogra, E. Okubo and M. Takahira, Distinct uptake of tellurate from selenate in a selenium accumulator, Indian mustard (Brassica juncea), Metallomics, 2010, 2, 328–333 RSC.
- A. M. Bakke, D. H. Tashjian, C. F. Wang, S. H. Lee, S. C. Bai and S. S. Hung, Competition between selenomethionine and methionine absorption in the intestinal tract of green sturgeon (Acipenser medirostris), Aquat. Toxicol., 2010, 96, 62–69 CrossRef CAS PubMed.
- G. N. Schrauzer, The nutritional significance, metabolism and toxicology of selenomethionine, Adv. Food Nutr. Res., 2003, 47, 73–112 CAS.
- D. R. Ellis, T. G. Sors, D. G. Brunk, C. Albrecht, C. Orser, B. Lahner, K. V. Wood, H. H. Harris, I. J. Pickering and D. E. Salt, Production of Se-methylselenocysteine in transgenic plants expressing selenocysteine methyltransferase, BMC Plant Biol., 2004, 4, 1–11 CrossRef PubMed.
- A. Tagmount, A. Berken and N. Terry, An essential role of s-adenosyl-L-methionine:L-methionine s-methyltransferase in selenium volatilization by plants. Methylation of selenomethionine to selenium-methyl-L-selenium-methionine, the precursor of volatile selenium, Plant. Physiol., 2002, 130, 847–856 CrossRef CAS PubMed.
- Y. Ogra and Y. Anan, Selenometabolomics: Identification of selenometabolites and specification of their biological significance by complementary use of elemental and molecular mass spectrometry, J. Anal. At. Spectrom., 2009, 24, 1477–1488 RSC.
- L. D. Lawson and B. G. Hughes, Characterization of the formation of allicin and other thiosulfinates from garlic, Planta Med., 1992, 58, 345–350 CrossRef CAS PubMed.
- S. M. Lippman, E. A. Klein, P. J. Goodman, M. Scott Lucia, I. M. Thompson, L. G. Ford, H. L. Parnes, L. M. Minasian, J. Michael Gaziano, J. A. Hartline, J. Kellogg Parsons, J. D. Bearden III, E. David Crawford, G. E. Goodman, J. Claudio, E. Winquist, E. D. Cook, D. D. Karp, P. Walther, M. M. Lieber, A. R. Kristal, A. K. Darke, K. B. Arnold, P. A. Ganz, R. M. Santella, D. Albanes, P. R. Taylor, J. L. Probstfield, T. J. Jagpal, J. J. Crowley, F. L. Meyskens Jr., L. H. Baker and C. A. Coltman Jr., Effect of selenium and vitamin E on risk of prostate cancer and other cancers: The selenium and vitamin E cancer prevention trial (SELECT), J. Am. Med. Assoc., 2009, 301, 39–51 CrossRef CAS PubMed.
Footnote |
† Electronic supplementary information (ESI) available. See DOI: 10.1039/c6mt00128a |
|
This journal is © The Royal Society of Chemistry 2017 |
Click here to see how this site uses Cookies. View our privacy policy here.