DOI:
10.1039/C6RA25934K
(Paper)
RSC Adv., 2017,
7, 6849-6855
Effect of humins from different sediments on microbial degradation of 2,2′,4,4′,5,5′-hexachlorobiphenyl (PCB153), and their polyphasic characterization
Received
27th October 2016
, Accepted 12th January 2017
First published on 20th January 2017
Abstract
This study was carried out to evaluate the activity of humins from four different sediments as electron mediators for microbial reductive dechlorination of 2,2′,4,4′,5,5′-hexachlorobiphenyl (PCB153) and to analyze the electron-mediating characteristics of humins. All the humins showed stable electron-mediating activity in microbial dechlorination with the total removal of PCB153 in the range 0.19–0.32 mg L−1. Among the humins examined, that extracted from Qizhen Lake sediment, which was the richest in carbon (4.79%) and contained the highest concentration of Fe (11.04 mg g−1 sample), showed the best performance. Fourier transform infrared analysis showed that all the humins exhibited similar spectra with varying absorbance intensity. A prominent band detected near 1635 cm−1 indicated the presence of C
C and C
O bonds, which are generally considered to be associated with redox reactions. Cyclic voltammetry analysis confirmed the presence of redox-active moieties in all the humins, with the estimated redox potentials ranging from −0.4 to 0.3 V (versus a standard hydrogen electrode). The polyphasic characterization of humins and the observed stable microbial community composition regardless of humin origin indicated that microbial dechlorination activity is associated with multiple properties of humins, notably Fe content and the presence of C
O and C
C bonds and redox-active moieties.
1. Introduction
Naturally occurring humic substances (HSs) are redox-active organic matters that are widely distributed in the environment, including soils, sediments, peatlands, and wetlands.1 HSs consist of the tissue residues of plants, animals, and microbes at different stages of decay,2 and various types of heterogeneous organic matter closely combined with inorganic matter.3 HSs are considered to be recalcitrant organic materials; however, they have been reported to be dynamically involved in carbon and electron flow in anaerobic environments,4 which implies their potential role in the transformation and detoxification of pollutants.5 During the past two decades, the function of HSs as electron mediators has been studied extensively using soluble HSs (such as humic acid) and their quinoid analogues.6,7
From 1929 to 1978, polychlorinated biphenyls (PCBs), a typical group of highly recalcitrant chlorinated organic pollutants, were widely used in electrical capacitors, plasticizers, transformers, flame retardants, solvent extenders, and dielectric fluids for industrial applications because of their favorable dielectric properties and chemical stability.8 Despite longstanding prohibitions on their manufacture and usage, PCBs are still widespread and persistently distributed throughout global ecosystems, particularly in the bottom sediments of rivers, lakes, and marine environments.9–12 PCBs pose a significant threat to ecosystems and to human health because of their extraordinary stability and high toxicity, e.g., accumulation and metabolism of fat-soluble PCBs in humans can lead to developmental and reproductive toxicity, endocrine effects, dermal toxicity, carcinogenesis, and hepatotoxicity.10 According to the International Council for the Exploration of the Seas (ICES), the presence of PCBs, particularly the isomers 101, 118, 138, and 153, is regarded as a marker of the degree of industrial contamination.13 2,2′,4,4′,5,5′-Hexachlorobiphenyl (PCB153) is a high-chlorinated PCB congener and one of the most prevalent PCB congeners associated with environmental contamination.14 In terms of the microbial decontamination of PCB congeners, aerobic microorganisms can only degrade the low-chlorinated PCB congeners (i.e., those with ≤5 Cl atoms). Some anaerobic microorganisms, however, are able to use high-chlorinated PCBs as electron acceptors.15 Anaerobic bioremediation has accordingly attracted attention as a potential cost-effective technology for detoxifying high-chlorinated PCBs.16 However, it is known that PCB-dechlorinating cultures typically require soils or sediments to sustain dechlorination activities.11,17 To date, however, the roles of soils or sediments in PCB dechlorination activity have been poorly analyzed.
Recently, we reported for the first time that solid-phase humin, a fraction of HSs that is insoluble under all pH conditions, serves as an electron mediator for maintaining microbial reductive dechlorination of pentachlorophenol (PCP) and reductive debromination of tetrabromobisphenol A (TBBPA).18–20 In these cultures, soil was successfully replaced with humin to maintain microbial dehalogenation activity.18–20 Solid-phase humin, being a substance of natural origin, is suitable for utilization as an electron mediator in in situ remediation because of its non-toxic nature and effective retention within the system. These findings and the ubiquity of humin suggest that this naturally occurring material might be useful as a solid-phase redox mediator for dehalogenation of highly halogenated aromatic compounds. Therefore, in this study, we aimed to investigate whether humins can sustain PCB153 dechlorination activity by serving as electron mediators, and to determine the influence of natural sources of humins on their role as solid-phase electron mediators.
Here, a sediment-dependent PCB-dechlorinating culture was used as an inoculum source for examining the activity of humins from different natural sediments as electron mediators. We also performed chemical, spectroscopic, and electrochemical analyses of humins, and microbial community analysis in order to gain insights into the electron-mediating nature of humins.
2. Materials and methods
2.1. Sediment samples
Sediment samples used in this study were obtained from four sources (Lingqiao river sediment [29°51′59′′N, 121°33′35′′E], Xixi wetland sediment [30°15′′27′′N, 120°3′25′′E], Qizhen lake sediment [30°18′28′′N, 120°5′3′′E], and Huzhou pond sediment [30°46′38′′N, 120°9′5′′E]) in Zhejiang Province, China. These samples were air dried and stored at 4 °C in a refrigerator until use.
2.2. Extraction and purification of humins
Humins were obtained from sediments as previously described.18 Briefly, air-dried sediments were extracted with 2% hydrofluoric acid (HF) to remove the clay minerals and silicate. The HF-treated residue was then extracted at least 10 times with 0.1 M NaOH until there was no obvious dark color in the extract. The sediment residue remaining after alkaline extraction was further treated with 2% HF to concentrate the organic matter. Finally, the residue was thoroughly rinsed with distilled water to neutralization and freeze-dried as the humin. All the solutions and distilled water used during the extraction were flushed with N2, and all the centrifugation steps were carried out at 8000 × g for 10 min. Humins extracted from sediment collected from the Lingqiao river (LQ), Xixi wetland (XX), Qizhen lake (QZ), and Huzhou pond (HZ) were designated as LQ-HM, XX-HM, QZ-HM, and HZ-HM, respectively.
2.3. Influence of humin on microbial reductive PCB153 dechlorination
An anaerobic PCB153-dechlorinating humin culture was enriched from a sediment-dependent PCB153-dechlorinating culture (unpublished data). The sediment culture had been maintained through serial transfers of 5% (v/v) of the inoculum for three generations before transferring to fresh medium using humin as a substitute for sediment.
For preparation of the humin culture, we used 50 mL serum bottles containing 20 mL of mineral medium, 0.25 g of freeze-dried humin, 0.2 μm filter-sterilized vitamin solution,21 and 20 mM formate, all flushed with nitrogen gas. A stock solution of PCB153 (100 mg L−1 in acetone) was added to culture, yielding a final concentration of 0.5 mg L−1. The bottles were sealed using Teflon-coated butyl rubber stoppers and aluminum seals, and autoclaved at 121 °C for 30 min. The cultures were incubated statically at 30 °C in the dark for 90 days. The mineral medium consisted of (per liter) 1.0 g of NH4Cl, 0.05 g of CaCl2·2H2O, 0.1 g of MgCl2·6H2O, 0.4 g of K2HPO4, 1 mL of trace element SL-10 solution, 1 mL of Se/W solution, 15 mM MOPS buffer (pH 7.2), and 50 mg L−1 resazurin-Na.22
The influence of the humins from different sediments on the microbial PCB153 dechlorination activity was examined by conducting an incubation experiment using the same procedure as that used for culture maintenance. Humins were added at the same concentration and autoclaved with the medium. After appropriate incubation periods, 1 mL of the culture was sampled, and then PCB153 and its metabolites in the culture were extracted with acetone and n-hexane (1
:
9) and analyzed using a gas chromatography-mass spectrometry system (Agilent Technologies, GCMSD5975, United States) equipped with an HP-5MS column (Agilent Technologies, United States). The cultures incubated with different humins were transferred (5%, v/v) to fresh medium (as described above) containing 0.5 mg L−1 of PCB153 after the same incubation period (approx. 90 days). A biotic control without the addition of humin, but with inoculum, and an abiotic control without inoculum but with QZ-HM were also analyzed. Recovery rates of PCB153 (0.5 mg L−1) during the extraction process were also examined. The influence of humins on dechlorination was assessed after at least two transfers of the culture into new medium. All of the experiments were performed in duplicate sets, and each set was repeatedly examined at least three times to confirm the results.
2.4. Chemical characterization
The contents of carbon, hydrogen, nitrogen, and sulfur in humins of different sediments were determined using a Yanaco MT-5 CHN-corder (Yanaco New Science Inc., Kyoto, Japan), with antipyrine used as a standard. Metals in humins were analyzed using inductively coupled plasma atomic emission spectroscopy (Optima 3300DV, PerkinElmer, Yokohama, Japan) after digestion with perchloric acid and nitric acid.
2.5. Spectroscopic characterization
Fourier transform infrared (FTIR) spectra of humins were determined using the KBr pellet technique in the 400–4000 cm−1 range at a resolution of 4 cm−1 and eight accumulation modes using a JASCO FT/IR-6100 spectrometer (JASCO, Tokyo, Japan). Correction of spectra was performed using pure KBr and ambient air.
2.6. Electrochemical analysis
Cyclic voltammetry (CV) measurements were obtained under anaerobic conditions using a potentiostat (HSV-110; Hokuto Denko Inc., Osaka, Japan), consisting of a twisted platinum (Pt) working electrode and counter electrode (0.8 mm × 1 m; Nilaco, Tokyo, Japan), and an Ag/AgCl reference electrode (6 mm × 15 cm; Fusheng Analytical Instruments Co., Shanghai, China). For the CV analysis, 0.25 g of humin sample was suspended in 50 mL of dimethyl sulfoxide (DMSO) containing 1 mM NaClO4 as an electrolyte.23 The scan rate was 10 mV s−1, with a potential range of −2.0 to 0.0 V (versus Ag/AgCl). All test solutions were flushed with high-purity N2 prior to the measurement. Background scans were performed before each measurement.
2.7. Denaturing gradient gel electrophoresis (DGGE)
The microbial communities in the PCB153-dechlorinating cultures containing different humins were characterized by PCR-DGGE. Bacterial 16S rRNA genes were amplified with the universal primers 357f with a GC clamp and 517r.24 Resolved bands were excised from the DGGE gel and sequenced as described previously.20 Sequence similarity searches were performed in the GenBank data library using the BLAST program.
3. Results
3.1. The influence of humins on microbial reductive PCB153 degradation
Fig. 1 shows the PCB153 dechlorination in cultures containing different humins after incubation for 90 days. All humins obtained from the four different sediments stably maintained the microbial PCB153 dechlorination activity as electron mediators. The major identified dechlorination products were 2,2′,5,5′-tetrachlorobiphenyl (PCB52) and 2,2′,4,5,5′-pentachlorobiphenyl (PCB101). PCB101 is an intermediate product that is further dechlorinated soon after generation, and thus typically does not accumulate. PCB52, derived via the removal of two chlorines from PCB153, was not further transformed, and remained as the final dechlorination product. After 90 days, 0.19, 0.27, 0.32, and 0.26 mg L−1 of PCB153 was dechlorinated, with the production of 0.15, 0.25, 0.31, 0.23 mg L−1 of PCB52 in cultures containing LQ-HM, XX-HM, QZ-HM, and HZ-HM, respectively. The biotic control without the addition of humin showed very weak dechlorination activity, with the production of only 0.02 mg L−1 of PCB52. The abiotic control without inoculum did not show any dechlorination activity. Collectively, these results indicated that PCB dechlorination could be attributed to microbial activity and that humins functioned as electron mediators for PCB dechlorination. QZ-HM showed the best performance, followed in descending order by XX-HM, HZ-HM, and LQ-HM. Of the initial 0.5 mg L−1 PCB153 in cultures, approximately 0.18 mg L−1 remained in the cultures containing QZ-HM after incubation for 90 days. The XX-HM culture had a dechlorination rate similar to that of the HZ-HM culture, whereas the LQ-HM culture exhibited the slowest dechlorination rate. The recovery rate of PCB153 during the extraction process was in the range 90–105%.
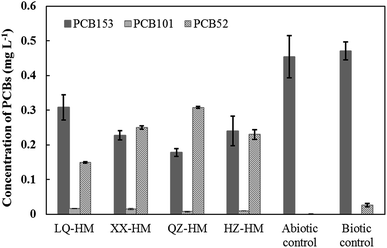 |
| Fig. 1 Comparison of dechlorination of PCB153 in cultures containing different humins after incubation for 90 days. Data show the mean values of duplicate cultures, and vertical bars show the difference in duplicate samples. | |
3.2. Chemical characterization of humins
The yield (by weight), nonmetalloid element contents (C, H, N, and S), and metal element contents (Fe, Cu, Mn, Zn, Cd, Cr, and Pb) of the examined humins are shown in Tables 1 and 2. The yield of humins varied from 44.9 to 537.9 mg per g sediment. The high yield of HZ-HM may be attributed to the fact that the source sediment was sampled from the bottom of an aquaculture pond used for rearing black carp, the excrement and feces of which contain large amounts of organic materials that contribute to HS formation.
Table 1 The physicochemical properties of humin samples
Humins |
Yield (mg humin per g sediment) |
Elemental composition (%) |
C/N |
H/C |
H/N |
C |
H |
N |
S |
LQ-HM |
44.9 |
2.90 |
1.14 |
0.17 |
0.10 |
17.39 |
0.39 |
6.84 |
XX-HM |
174.2 |
2.66 |
1.55 |
0.18 |
0.10 |
14.44 |
0.58 |
8.40 |
QZ-HM |
49.0 |
4.79 |
1.90 |
0.29 |
0.38 |
16.30 |
0.40 |
6.46 |
HZ-HM |
537.9 |
1.51 |
1.19 |
0.09 |
0.12 |
16.55 |
0.78 |
12.97 |
Table 2 Concentrations of metals in humin samples (mg per g humin)b
Origin of sediment |
Extracted huminsa |
Fe |
Cu |
Mn |
Zn |
Cr |
Cd |
Pb |
Humins were extracted from sediment collected in Lingqiao river (LQ-HM), Xixi wetland (XX-HM), Qizhen lake (QZ-HM), and Huzhou pond (HZ-HM). N.D denotes below the detection limit. |
Lingqiao river |
LQ-HM |
9.93 |
0.43 |
0.19 |
0.10 |
0.09 |
N.D |
N.D |
Xixi wetland |
XX-HM |
10.19 |
0.48 |
0.21 |
0.10 |
0.10 |
N.D |
N.D |
Qizhen lake |
QZ-HM |
11.04 |
0.88 |
0.48 |
0.46 |
0.07 |
0.01 |
0.04 |
Huzhou pond |
HZ-HM |
10.56 |
0.52 |
0.34 |
0.10 |
0.07 |
N.D |
N.D |
The C, H, N, and S contents (Table 1) varied in the range of 1.51–4.79% for C, 1.14–1.90% for H, 0.09–0.29% for N, and 0.10–0.38% for S. To obtain more detailed information on humins, the atomic ratios of C/N, H/C, and H/N were calculated from the elemental composition contents. The atomic ratios H/C, C/N, and H/N differed widely among the different humins: from 0.39 to 0.78, 14.44 to 17.39, and 6.46 to 12.97, respectively.
All the humins contained high concentrations of Fe (9.93–11.04 mg per g humin) and Cu (0.43–0.88 mg per g humin) (Table 2). Concentrations of Mn, Zn, and Cr were detected ranging from 0.19 to 0.48, 0.10 to 0.46, and 0.07 to 0.10 mg per g humin, respectively. The concentrations of Cd and Pb were very low in QZ-HM, and they were below the limit of detection in LQ-HM, XX-HM, and HZ-HM.
3.3. Spectroscopic properties of humins
FTIR spectra of humins are shown in Fig. 2. It can be seen that bands near 3673 and 3618 cm−1 as well as a broad peak at 3409 cm−1 are present in all humins, which were assigned to the ν(OH) vibration of carboxylic or phenolic groups.25 The peaks appearing at approximately 2919 and 2844 cm−1 can be attributed to the symmetric and asymmetric vibrations of aliphatic groups (CH), respectively.25 The band appearing at 1635 cm−1, which was most prominent in QZ-HM, was assigned to the stretching of phenyl-conjugated C
C and C
O bonds, whereas another band near 1476 cm−1 was assigned to CH3 deformation and CH2 bending. The band appearing at approximately 1371 cm−1 was assigned to symmetric stretching of COO− and to the bending vibrations (δ) of aliphatic groups.25 The band at 900 cm−1 was assigned to aromatic CH deformation.19
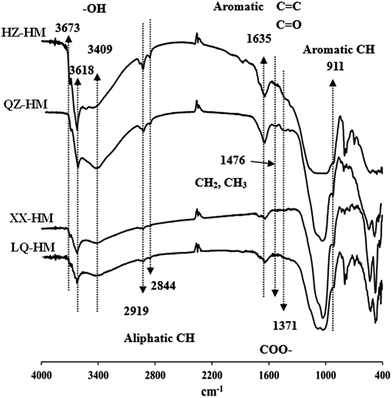 |
| Fig. 2 Fourier transform infrared (FTIR) spectra of humins extracted from four different sediments. Abbreviated names of the humins are the same as those presented in Table 1. | |
3.4. Electrochemical properties of humin
To assess the redox-active moieties in the humins, CV experiments were carried out using DMSO as a solvent. Humic substances generally produce cyclic voltammograms with little or no useful structures because of the lack of electrode activity, DMSO was applied to increase the reactivity between the humin and the electrode.18 A redox couple was observed in all the humin samples (Fig. 3), and was particularly pronounced in QZ-HM. The redox potential of humin was estimated from CVs in DMSO, and the potential in DMSO versus Ag/AgCl was approximately equal to the potential in H2O versus a standard hydrogen electrode (SHE) plus 0.45 V.23 This had previously been confirmed in our research on soil humins.18 On the basis of this correction, the redox potentials of humins were estimated to be −0.38 V (versus SHE) for LQ-HM, −0.40 V for XX-HM, −0.35 V for QZ-HM, and −0.30 V for HZ-HM.
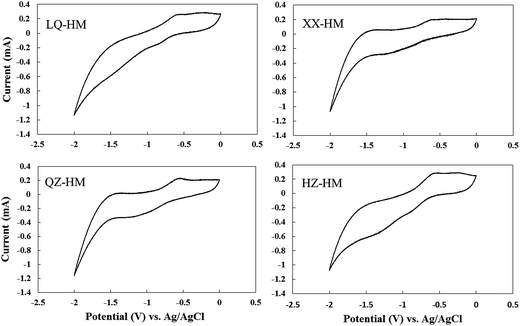 |
| Fig. 3 Cyclic voltammetry of different humins using dimethyl sulfoxide as a solvent. Abbreviated names of the humins are shown in the voltammograms, which are the same as those presented in Table 1. | |
3.5. Microbial community analysis based on 16S rRNA gene sequencing
Fig. 4 shows PCR-DGGE profiles of the microbial communities in the PCB153-dechlorinating cultures containing different humins after incubation for 90 days. The microbial communities in the different PCB153-dechlorinating cultures were found to be comparable, and there were no obvious differences in the predominant bacteria. Bands 1, 2, and 3 were, respectively, closely related to an uncultured bacterium (NCBI accession number: HQ688519; 96% similarity between the band sequence and the closely related reference sequence), Clostridium sp. (AB275141; 96% similarity), and Bacillus sp. (JX434141; 95% similarity).
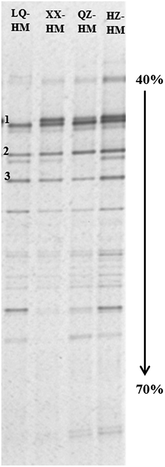 |
| Fig. 4 Denaturing gradient gel electrophoresis (DGGE) fragment patterns of bacterial 16S rRNA genes amplified from DNAs in the PCB153-dechlorinating cultures containing different humins after incubation for 90 days. The long arrow represents the direction of the gel gradient (40–70%). The bands that migrated to a similar position in the gel were presumed to have similar sequences. Bands (1–3) were excised from the gel and sequenced. | |
4. Discussion
In this study, we successfully replaced sediment with humin in a sediment-dependent PCB153-dechlorinating culture. All humins used in this study showed stable electron-mediating activity in microbial reductive PCB153 dechlorination, although with varying effectiveness. The major identified dechlorination products were PCB52 and PCB101. PCB52, which is derived from PCB153 via the removal of two chlorines, did not undergo further transformation and remained as the final dechlorination product. This finding is not exceptional, as many anaerobic PCB153 dechlorination cultures can only transform PCB153 to tetra- or pentachlorinated congeners.26 It is generally accepted that highly chlorinated PCB congeners can undergo reductive dechlorination in anaerobic environments, with meta- and para-chlorines being more susceptible to dechlorination than ortho-chlorines,27 and that highly chlorinated PCBs are initially anaerobically dechlorinated to lightly chlorinated PCB congeners that can subsequently be degraded by aerobic bacteria.28 It has also been demonstrated that PCB biotransformation is enhanced and accelerated in paddy fields subjected to a succession of dry and flooded conditions (an alternating oxic–anoxic environment).29 Numerous researchers have accordingly combined anaerobic and aerobic processes for effective biodegradation of PCBs.30
No dechlorination metabolites were detected in the abiotic control and the observed losses of PCB153 in this culture could be attributable to volatilization and adsorption. Compared with the cultures containing humins, the biotic control without the addition of humin showed considerably weaker dechlorination activity. The dechlorination of PCBs was thus obviously enhanced by the application of humin to cultures. These results confirmed the electron-mediating function of humins for PCB153 dechlorination and indicate that humin may function as a naturally occurring solid electron mediator for the widespread application of halorespiring microorganisms. Furthermore, all the humins may have similar characteristics regarding the structural properties that are conducive to electron mediation during the reductive dehalogenation reactions. Therefore, in order to further understand the redox activity of humins, the chemical, spectroscopic, and electrochemical properties of these materials were analyzed: elemental composition, FTIR, and CV.
Humins extracted from different sediment origins showed different yields and elemental compositions. This is consistent with the notion that HSs of different origin exhibit heterogeneity in their structural and compositional characteristics.31 The H/C ratios clustered around 1.0 suggest that their chemical structures mainly comprise an aromatic framework.32 Therefore, the low H/C ratios (from 0.39 to 0.78) detected in the present study imply the predominant aromatic nature of the extracted humins and their low degree of aliphatic condensation.
The analysis of metals in humin showed that the Fe content was positively correlated with the microbial PCB153 dechlorination rate. QZ-HM facilitated the best dechlorination performance, with 0.32 mg L−1 of PCB153 being removed after incubation for 90 days, indicating that the redox functions inherent in the humin from Qizhen lake sediment may be more active and can more effectively facilitate the electron-mediating process for dechlorination. This humin was richest in C (4.79%), N (0.29%), and S (0.38%), and contained the highest concentrations of metals, particularly Fe (11.04 mg per g humin) and Cu (0.88 mg per g humin). Consistently, LQ-HM, which had the lowest concentration of Fe (9.93 mg per g humin), showed the lowest dechlorination rate. It has previously been demonstrated that Fe in humin is very important for the electron-mediating activity of humin and that Fe can activate and stabilize the electron-mediating groups harbored in HSs.33,34 Consequently, the high Fe concentration in QZ-HM may explain the good performance of this humin as an electron mediator.
Fig. 2 shows that the four different sediment humins examined in the present study exhibit similar FTIR spectra with different absorbance intensity, indicating that these humins possess similar structures and may contain the same classes of functional groups regardless of their origin. The band near 1635 cm−1 indicates the presence of C
O and C
C double bonds, which are generally considered to be associated with redox reactions. This is consistent with the dechlorination performance of the humins, as QZ-HM exhibited the most prominent band. Moreover, QZ-HM had the broadest peak at 3409 cm−1, which was assigned to the ν(OH) vibration of carboxylic or phenolic groups.25 These groups might primarily contribute to the redox activity of humin, probably in the form of organometallic compounds, particularly those complexed with Fe.33 In addition, some studies have shown that the immobilization of alumina nanoparticles or complexation of metal on humic substances could enhance the bioremediation of contaminants as the electron mediator.33–36 Moreover, nitrogen and sulfur functional groups, which have been reported as non-quinone redox sites, may also be considered the redox-active functional groups harbored within humins.37,38
Redox-active moieties were observed in all the sediment humins used in this study, and the estimated redox potential of these humins ranged from −0.4 to −0.3 V (versus SHE), as determined by an analysis of the redox peaks obtained in the CV results (Fig. 3). The intermediate values of the redox potential of humins, between that of formate oxidation (−430 mV)39 and reductive dechlorination with para-chlorines of PCBs (range of 274–472 mV)40 are consistent with their function as electron mediators between formate oxidation and microbial dechlorination reactions. These data demonstrated that the redox-active moieties present in humins can be classified in the same category despite their heterogeneous nature. It has been reported that the response of quinone moieties on Pt electrodes can be improved in DMSO due to the strong chemisorption of DMSO to Pt.23 Therefore, the improved CV signals for humins in DMSO are considered to be attributed to quinone-type structures, which have been reported as redox-active moieties.41 In addition, the CV of QZ-HM exhibits a well-defined redox couple, whereas the CV of LQ-HM is narrow and the redox couple is not obvious. These observations are consistent with the dechlorination performance of humins.
On the basis of the 16S rRNA gene analysis using PCR-DGGE, the compositions of microbial communities in the PCB153-dechlorinating cultures containing different humins were both comparable and very stable (Fig. 4). The stability of the microbial composition was very important for successive subcultivation and activity maintenance. Moreover, these results further demonstrated that the differences in dechlorination activities among the cultures containing different humins were attributable to the humins, rather than to the microbial community. However, we were unable to detect a band corresponding to the dechlorination bacterium, which suggests that the functional bacterium was not dominant in these cultures. Further work is therefore required to confirm the functional bacterium for PCB dechlorination, and to more fully understand and utilize this humin-dependent PCB dechlorination culture.
By acting as an electron mediator, solid-phase humin successfully maintained the dechlorination activity in PCB153 dechlorination culture. This has important implications for the detoxification of highly chlorinated PCBs, given the typical requirements for soils or sediments in anaerobic PCBs dechlorination cultures.11,17 Although all the sediment humins examined in the present study facilitated the microbial reductive PCB153 dechlorination as electron mediators, their activity differed. The chemical, spectroscopic, and electrochemical properties of the examined humins showed that the microbial dechlorination activity was associated with multiple properties of humin, particularly the Fe content, C
O and C
C bands, and ν(OH) vibration of carboxylic or phenolic groups, as well as redox-active moieties. Further study is warranted to elucidate the functional groups of humin that act as electron mediators, and to determine the mechanism of electron transfer between humin and microorganisms.
5. Conclusions
Four humin samples from different types of sediment were successfully applied in mediating microbial reductive PCB dechlorination and their properties were characterized polyphasically. All the humins showed stable electron-mediating activity in microbial reductive PCB153 dechlorination, although with differing effectiveness. The microbial community compositions of the examined humins were comparable, despite the different origins of the humins, which further indicated that the observed differences in dechlorination rate were attributable to the humins, rather than to the microorganisms. The polyphasic characterization of the humins indicated that they possess similar structures and may contain the same classes of functional groups, whereas the microbial dechlorination activity was affected by multiple properties of humin, particularly the Fe content, C
O and C
C bands, ν(OH) vibration of carboxylic or phenolic groups, and redox-active moieties. Our study should advance our understanding of the dechlorination mechanism in sediment-dependent PCB dechlorination culture and the role of humin as an electron mediator in the environment.
Acknowledgements
This study was supported in part by the National Natural Science Foundation of China (No. 31400096). All the authors declare that they have no conflicts of interest.
Notes and references
- Y. P. Chin, G. Aiken and E. O'Loughlin, Environ. Sci. Technol., 1994, 28, 1853–1858 CrossRef CAS PubMed.
- A. Schaeffer, P. Nannipieri, M. Kästner, B. Schmidt and J. Botterweck, J. Soils Sediments, 2015, 15, 1865–1881 CrossRef.
- A. Miltner, P. Bombach, B. Schmidt-Brücken and M. Kästner, Biogeochemistry, 2012, 111, 41–55 CrossRef CAS.
- K. H. Kang, H. S. Shin and H. Park, Water Res., 2002, 36, 4023–4032 CrossRef CAS PubMed.
- D. R. Lovley, J. D. Coates, E. L. BluntHarris, E. J. P. Phillips and J. C. Woodward, Nature, 1996, 382, 445–448 CrossRef CAS.
- P. M. Bradley, F. H. Chapelle and D. R. Lovley, Appl. Environ. Microbiol., 1998, 64, 3102–3105 CAS.
- F. R. Van der Zee and F. J. Cervantes, Biotechnol. Adv., 2009, 27, 256–277 CrossRef CAS PubMed.
- D. L. Bedard, Polychlorinated biphenyls in aquatic sediments: environmental fate and outlook for biological treatment, in Dehalogenation, Springer, 2004, pp. 443–465 Search PubMed.
- B. R. deCastro, S. A. Korrick, J. D. Spengler and A. M. Soto, Environ. Sci. Technol., 2006, 40, 2819–2825 CrossRef CAS PubMed.
- S. Safe, Environ. Health Perspect., 1993, 100, 259–268 CrossRef CAS PubMed.
- H. Yu, C. Feng, X. Liu, X. Yi, Y. Ren and C. Wei, Environ. Pollut., 2016, 211, 81–89 CrossRef CAS PubMed.
- P. Wang, Q. Zhang, T. Wang, W. Chen, D. Ren, Y. Li and G. Jiang, RSC Adv., 2012, 2, 1350–1355 RSC.
- K. A. Bekiashev and V. V. Serebriakov, International Council for the Exploration of the Sea (ICES), in International Marine Organizations, Springer, 1981, pp. 465–477 Search PubMed.
- M. P. Longnecker, M. S. Wolff, B. C. Gladen, J. W. Brock, P. Grandjean, J. L. Jacobson, S. A. Korrick, W. J. Rogan, N. Weisglas-Kuperus and I. Hertz-Picciotto, Environ. Health Perspect., 2003, 111, 65–70 CrossRef CAS PubMed.
- G. Cagnetta, G. Intini, L. Liberti, V. V. Boldyrev and O. I. Lomovskiy, J. Soils Sediments, 2015, 15, 240–248 CrossRef CAS.
- X. Xu, Q. L. Zhao and M. S. Wu, RSC Adv., 2015, 5, 62534–62538 RSC.
- H. M. Van Dort, L. A. Smullen, R. J. May and D. L. Bedard, Environ. Sci. Technol., 1997, 31, 3300–3307 CrossRef CAS.
- C. Zhang and A. Katayama, Environ. Sci. Technol., 2012, 46, 6575–6583 CrossRef CAS PubMed.
- C. Zhang, D. Zhang, Z. Xiao, Z. Li, D. Suzuki and A. Katayama, Chemosphere, 2015, 131, 110–116 CrossRef CAS PubMed.
- C. Zhang, Z. Li, D. Suzuki, L. Ye, N. Yoshida and A. Katayama, Chemosphere, 2013, 92, 1343–1348 CrossRef CAS PubMed.
- C. Holliger, D. Hahn, H. Harmsen, W. Ludwig, W. Schumacher, B. Tindall, F. Vazquez, N. Weiss and A. J. Zehnder, Arch. Microbiol., 1998, 169, 313–321 CrossRef CAS PubMed.
- F. Widdel, G. W. Kohring and F. Mayer, Arch. Microbiol., 1983, 134, 286–294 CrossRef CAS.
- J. T. Nurmi and P. G. Tratnyek, Environ. Sci. Technol., 2002, 36, 617–624 CrossRef CAS PubMed.
- G. Muyzer, E. C. de Waal and A. G. Uitterlinden, Appl. Environ. Microbiol., 1993, 59, 695–700 CAS.
- O. Francioso, C. Ciavatta, C. Gessa, V. Tugnoli and S. Sanchez-Cortes, Soil Sci. Soc. Am. J., 1998, 62, 181–187 CrossRef CAS.
- L. Chen, Z. Gao and Z. Wang, J. Soils Sediments, 2016, 16, 318–323 CrossRef CAS.
- J. A. Field and R. S. Alvarez, Environ. Pollut., 2008, 155, 1–12 CrossRef CAS PubMed.
- H. I. Gomes, C. Dias-Ferreira and A. B. Ribeiro, Sci. Total Environ., 2013, 445, 237–260 CrossRef PubMed.
- C. Chen, C. Yu, C. Shen, X. Tang, Z. Qin, K. Yang, M. Z. Hashimi, R. Huang and H. Shi, Environ. Pollut., 2014, 190, 43–50 CrossRef CAS PubMed.
- E. R. Master, V. W. M. Lai, B. Kuipers, W. R. Cullen and W. W. Mohn, Environ. Sci. Technol., 2001, 36, 100–103 CrossRef.
- M. Thomsen, P. Lassen, S. Dobel, P. E. Hansen, L. Carlsen and B. B. Mogensen, Chemosphere, 2002, 49, 1327–1337 CrossRef CAS PubMed.
- G. R. Aiken, D. M. McKnight, R. L. Wershaw and P. MacCarthy, Humic substances in soil, sediment, and water: geochemistry, isolation and characterization, John Wiley & Sons, 1985 Search PubMed.
- C. Zhang, D. Zhang, Z. Li, T. Akatsuka, S. Yang, D. Suzuki and A. Katayama, Environ. Sci. Technol., 2014, 48, 6318–6325 CrossRef CAS PubMed.
- A. S. Cruz-Zavala, A. M. Pat-Espadas, J. R. Rangel-Mendez, L. F. Chazaro-Ruiz, J. A. Ascacio-Valdes, C. N. Aguilar and F. J. Cervantes, Bioresour. Technol., 2016, 207, 39–45 CrossRef CAS PubMed.
- L. H. Alvarez, L. Jimenez-Bermudez, V. Hernandez-Montoya and F. J. Cervantes, Water, Air, Soil Pollut., 2012, 223, 1911–1920 CrossRef CAS.
- F. J. Cervantes, R. Gomez, L. H. Alvarez, C. M. Martinez and V. Hernandez-Montoya, Biodegradation, 2015, 26, 289–298 CrossRef CAS PubMed.
- N. Ratasuk and M. A. Nanny, Environ. Sci. Technol., 2015, 41, 7844–7850 CrossRef.
- V. Hernandez-Montoya, L. H. Alvarez, M. A. Montes-Moran and F. J. Cervantes, Geoderma, 2012, 183, 25–31 CrossRef.
- U. Schröder, Phys. Chem. Chem. Phys., 2007, 9, 2619–2629 RSC.
- J. Dolfing and B. K. Harrison, Environ. Sci. Technol., 1992, 26, 2213–2218 CrossRef CAS.
- M. Uchimiya and A. T. Stone, Chemosphere, 2009, 77, 451–458 CrossRef CAS PubMed.
Footnote |
† These authors contributed equally to this study and are joint first authors. |
|
This journal is © The Royal Society of Chemistry 2017 |
Click here to see how this site uses Cookies. View our privacy policy here.