DOI:
10.1039/C6RA26087J
(Paper)
RSC Adv., 2017,
7, 5315-5321
Magnetic damping and perpendicular magnetic anisotropy in Pd-buffered [Co/Ni]5 and [Ni/Co]5 multilayers
Received
31st October 2016
, Accepted 31st December 2016
First published on 17th January 2017
Abstract
We systematically studied the influence of Pd underlayer on the magnetic properties of perpendicular [Co/Ni]5 and [Ni/Co]5 multilayers by time-resolved magneto-optical Kerr effect. We found that the saturated magnetic damping constant α0 increases continuously with increasing the Pd thickness tPd, showing no linear correlation with the perpendicular magnetic anisotropy constant Ku for both series of samples. As compared to the Co/Ni sample with the same tPd, the Ni/Co film shows lower saturation magnetization, weaker Ku but larger α0, which can be ascribed to the presence of more paramagnetic spins at the Pd/Ni interface due to the weak exchange coupling stiffness between Ni atoms. By analyzing extrinsic damping contributions from magnetic inhomogeneities, two-magnon scattering and spin pumping effects, the intrinsic damping of perpendicular [Ni/Co]5 is quantitatively determined to be 0.023. This study should provide deep understanding and effective control of magnetic damping for designing high performance magnetic memory devices.
Introduction
In recent years, magnetic multilayers or thin films with strong perpendicular magnetic anisotropy (PMA) have been widely studied due to their potential applications in spin-transfer torque magnetic random access memory (STT-MARM) and other spintronic devices.1–4 In order to increase the STT-memory density and lower the switching energy, reducing the critical switching current density Jc is a major concern. The Jc is known to be proportional to the effective perpendicular magnetic anisotropy energy and the magnetic damping constant. And it is commonly believed that the perpendicular magnetic thin films with high Ku usually has a larger damping because of the strong spin–orbit interaction.5 For instance, a linear relationship between intrinsic Gilbert damping constant α0 and PMA strength has been found in some perpendicular thin films of Pt/Co/Pt sandwich, [Co/Pd]8 multilayers, and L10-FePd1−xPtx ternary alloy.6–8 However, such a correlation doesn't apply to the perpendicular Ta/CoFeB/MgO or Co/Pt multilayer systems.9,10 The conflicting opinions, we speculate, are most probably caused by the incomprehensive consideration for various damping contributions. As we know, in addition to the intrinsic Gilbert damping resulting from spin–orbit coupling of the ferromagnetic materials, the local fluctuations of magnetization, magnetic anisotropy, as well as the magneto static fields at different sample locations would give rise to some extrinsic contributions. These extrinsic damping αex caused by inhomogeneous magnetic properties strongly relies on the surface/interface roughness and other film defects.11,12 Moreover, at the ferromagnet (FM)/non-magnetic (NM) metal layer interface, the magnetization precession will transfer spins to the adjacent NM layers, which can also dissipate spin energy and therefore enhance the effective damping constant via spin-pumping effect.13–15 In order to effectively control the magnetization switching dynamics, magnetic damping terms resulting from different origins should be carefully considered and controlled for practical applications.
Among the various perpendicular magnetic film structures, Co/Ni multilayer has attracted a great deal of interest due to its high spin polarization and low magnetic damping. Generally, a nonmagnetic underlayer with a preferred fcc (111) orientation is indispensable for achieving PMA in Co/Ni multilayers. Compared with the Cu, Au and Ti underlayer materials,16–19 the Pd or Pt is rarely employed because incorporation of such heavy metal with strong spin–orbit coupling would introduce a large extra spin pumping term to the magnetic damping, which is undesirable since it would enhance the critical STT switching current. However, more recently, the Pd and Pt layers have gained great attentions in some new emerging spin-dependent research areas, e.g. current-induced spin–orbit torque (SOT) switching, which is being considered as a potential new writing method for switching a perpendicular magnetic memory bit.20,21 A commonly used SOT device is based on a perpendicular multilayer stack consisting of heavy metal/ferromagnet (FM)/oxide layers, which allows for lower switching energy as compared with the conventional STT-MRAM. The SOT effect, arising from the spin–orbit coupling of the adjacent non-magnetic heavy metal layer such as Pt, Pd, Ta or W layer with a large spin Hall angle,22–24 can generate a pure spin current to exert strong torques on the magnetic layer. In order to develop high performance SOT-MRAMs and other SOT-related devices, it will be of vital importance to carry out a systematic study on magnetization dynamics for heavy metal-buffered perpendicular magnetic thin films.
In this work, we have fabricated Pd-buffered [Co/Ni]5 and [Ni/Co]5 perpendicular multilayers with either Co or Ni in contact with Pd, and investigated the influences of Pd thickness and multilayer deposition sequence on the perpendicular magnetic anisotropy and magnetic damping by means of time-resolved magneto-optical Kerr effect (TR-MOKE) technique.25 By analyzing and comparing the experimental results with those of Cu-buffered [Ni/Co]5 samples, the intrinsic magnetic damping constant of Co/Ni multilayer and spin-pumping contribution from Pd underlayer are identified.
Experiments
Using a Kurt J. Lesker magnetron sputtering system, multilayer films were deposited on amorphous Corning glass substrates at room temperature (RT) under a base pressure better than 5 × 10−8 Torr. By varying the magnetic layer deposition sequence, two series of samples have been fabricated: glass/Ta(3)/Pd(tPd)/[Co(0.26)/Ni(0.6)]5/Ta(3) and glass/Ta(3)/Pd(tPd)/[Ni(0.6)/Co(0.26)]5/Ta(3) (thickness in unit of nm), where the thickness of Pd layer tPd varies from 2.5 to 40 nm. For comparison, another series of samples with a structure of glass/Ta(3)/Cu(tCu)/[Ni(0.6)/Co(0.26)]5/Ta(3) were also prepared, where tCu is in the range of 2.5–20 nm. The film crystallinity was checked by X-ray diffraction and atomic force microscopy. The static magnetic properties and magnetization dynamic behaviors were respectively measured by a vibrating sample magnetometer (VSM) and a TR-MOKE system.26 The TR-MOKE measurement was performed by using a pulsed Ti:sapphire laser with a central wavelength of 800 nm, a pulse duration of 150 fs and a repetition rate of 1 kHz. A variable external magnetic field Hex was applied at an angle θH of 65° with respect to the film normal. The respective spot diameters of pump and probe laser pulses were about 1.0 and 0.2 mm, at almost perpendicular incidence to the film plane. For better Kerr signal and negligible heating effect, in this experiment the pump and probe laser fluences were chosen as F = 1.5 mJ cm−2 and 0.07 mJ cm−2, respectively.
Results and discussion
Fig. 1(a) and (b) representatively show the in-plane and out-of-plane magnetic hysteresis loops measured by VSM for the samples of Pd(5)/[Co(0.26)/Ni(0.6)]5 and Pd(5)/[Ni(0.6)/Co(0.26)]5. Clearly, all the samples exhibit PMA. Nevertheless, both the in-plane saturation magnetic field Hs and perpendicular coercivity Hc of [Ni/Co]5 series are smaller than those of [Co/Ni]5 series, implying the PMA strength is closely related to the adjacent magnetic layer material deposited on top of the Pd underlayer. In order to clearly interpret the variation trends of PMA strength, the saturation magnetization Ms and uniaxial magnetic anisotropy constant Ku are derived and plotted in Fig. 1(c) and (d) as a function of tPd. The Ku value is calculated according to the relationship of Ku = MsHs/2 + 2πMs2, where Ms is the saturation magnetization and Hs is the effective perpendicular anisotropy field obtained from the in-plane hysteresis loop. As shown in Fig. 1(c), apart from the initial enhancement arising from the induced polarization of Pd layer at tPd ≤ 5 nm,27 the Ms value decreases considerably with increasing the Pd layer thickness for both series of samples. We consider the reduction of Ms is due to the increased thickness of magnetically dead layer formed at the Pd/Co or Pd/Ni interface. The thicker Pd layer has a rougher surface,28 and thereupon would bring a thicker dead layer in which the magnetic spins are in a disordered (paramagnetic) state possibly due to intermixing or alloy formation.29 Fig. 1(d) shows the tPd dependence of Ku, it firstly increases and then decreases after reaching its maximum value at tPd = 10 nm. Such non-monotonic variation behavior comes from the competition between the roles of (111) texture and Pd surface roughness. In order to verify our interpretations, the XRD patterns for the Pd(tPd)/[Ni(0.6)/Co(0.26)]5 samples and the surfaces AFM images of glass/Pd(tPd) have been plotted respectively in Fig. 1(e) and (f)–(h). When tPd increases from 2.5 nm to 10 nm, the Ni/Co (111) peak enhances gradually and plays a dominant role on the initial increase of PMA strength. However, when tPd is over 10 nm, the peak intensity becomes decreasing mostly owing to the increased Pd surface roughness. As shown in Fig. 1(h), the root-mean-square roughness (Rq) of tPd = 40 nm is as large as 0.30 nm, which should be responsible for the subsequent degradation of Ms and Ku. In particular, it should be noted that for the samples with the same tPd, the Pd/[Ni/Co]5 structure owns much lower Ms and Ku than the Pd/[Co/Ni]5 one. This is understandable since the exchange coupling stiffness and Curie temperature of Ni layer is rather low as compared to the Co layer, leading to seriously disordered spins at the Pd/Ni interface.30
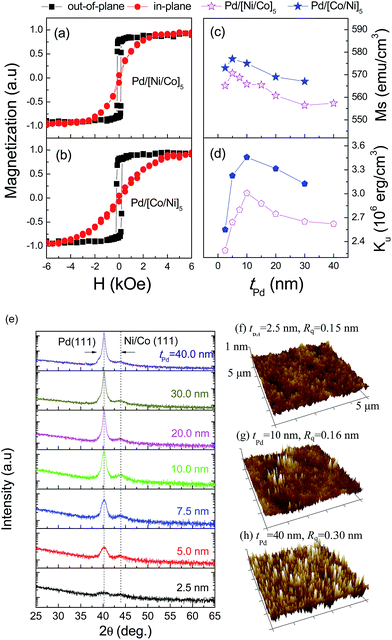 |
| Fig. 1 The in-plane and out-of-plane magnetic hysteresis loops for samples of (a) Pd(5)/[Ni(0.6)/Co(0.26)]5 and (b) Pd(5)/[Co(0.26)/Ni(0.6)]5. (c) The saturation magnetization Ms and (d) the uniaxial magnetic anisotropy energy density Ku as a function of Pd underlay layer thickness tPd for the two types of samples. (e) The XRD patterns for the [Ni(0.6)/Co(0.26)]5 samples with different tPd. (f)–(h) The AFM images measured on the sample surfaces of glass/Pd(tPd) with tPd = 2.5, 10 and 40 nm. | |
Ultrafast magnetization dynamics were measured by TR-MOKE to understand the influence of Pd underlayer on the magnetic damping constant. Fig. 2(a) displays the measured TR-MOKE data points measured at Hex = 11 kOe for Pd(tPd)/[Co/Ni]5 samples with various tPd. All the curves exhibit a precession and damping relaxation behavior, which were fitted by using the following equation,7,25
|
θk = a + b exp(t/t0) + c sin(2πft + φ)exp(−t/τ),
| (1) |
where the parameter
a corresponds to the background signal,
b and
t0 result from the slow magnetization recovery process,
c,
f,
φ, and
τ represent the precession amplitude, frequency, initial phase, and decay time of the magnetization dynamics, respectively. The fitted precession frequency
f and decay time
τ are respectively plotted in
Fig. 2(b) and (c) as a function of
Hex for the cases of
tPd = 5, 10, 20 nm. Apparently, the precession frequency
f increases monotonically with the
Hex. The discrete data points are fitted with the Kittel formula, 2π
f =
γ(
H1H2)
1/2, where
γ = 18.9 Mrad s
−1 Oe
−1 is the gyromagnetic ratio,
H1 =
Hex![[thin space (1/6-em)]](https://www.rsc.org/images/entities/char_2009.gif)
cos(
θH −
θ) +
Hkeff![[thin space (1/6-em)]](https://www.rsc.org/images/entities/char_2009.gif)
cos
2
θ and
H2 =
Hex![[thin space (1/6-em)]](https://www.rsc.org/images/entities/char_2009.gif)
cos(
θH −
θ) +
Hkeff![[thin space (1/6-em)]](https://www.rsc.org/images/entities/char_2009.gif)
cos
![[thin space (1/6-em)]](https://www.rsc.org/images/entities/char_2009.gif)
2
θ. The magnetization equilibrium angle
θ satisfies the energy-minimized equation of sin
![[thin space (1/6-em)]](https://www.rsc.org/images/entities/char_2009.gif)
2
θ = (2
Hex/
Hkeff)sin(
θH −
θ). As shown in
Fig. 2(b), all the data points are well fitted by the solid lines, indicating that the magnetization precesses coherently in a uniform mode. The fitted effective anisotropy field
Hkeff is very close to the measured VSM data, suggesting the employed laser fluence is appropriate and the sample properties are not affected by laser pumping.
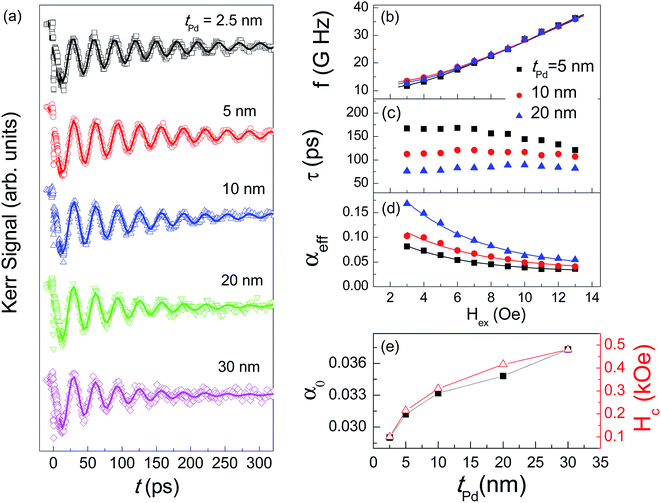 |
| Fig. 2 (a) The laser-induced magnetization dynamic Kerr signals (open squares) and the fitting curves (solid lines) for Pd(tPd)/[Co/Ni]5 multilayer films with various tPd measured at the Hex = 11 kOe and θH = 65°. (b–d) respectively shows magnetic field dependences of precession frequency f, decay time τ, and effective Gilbert damping constant αeff for tPd = 5, 10 and 20 nm. (e) The extracted magnetic damping constant α0 and the perpendicular magnetic coercivity Hc as a function of tPd. | |
Based on the fitted f and τ, the effective damping factor αeff can be derived from the simple approximate equation of αeff = 1/(2πfτ),17,25 which includes an intrinsic damping term α0 and an extrinsic damping term αex. It is usually believed that the extrinsic αex contains contributions from magnetic inhomogeneities, two-magnon scattering, and spin pumping effect of the adjacent nonmagnetic metal layer. The two-magnon scattering results from defects or inhomogeneities which scatter the uniform precession mode into the degenerate spin wave modes with finite wave vectors. The damping contribution due to two-magnon scattering is field angle dependent and becomes inoperative when the magnetization is oriented at an angle over 45° with the film plane. By applying a sufficiently high external magnetic field Hex, the extrinsic damping terms that results from inhomogeneous distributions of magnetization and/or magnetic anisotropy can be effectively eliminated whereas the spin pumping and two-magnon scattering contributions still exist, since the field angle is 25° with respect to the film plane in our TR-MOKE measurement. As shown in Fig. 2(d), all the αeff values decrease gradually with increasing the Hex. Nevertheless, they are still not saturated even at the maximum applied field of Hex = 13 kOe, implying the 13 kOe field is still not strong enough to completely remove the influence of magnetic inhomogeneities. In order to accurately determine the relationship between PMA strength and intrinsic magnetic damping, we utilize a decaying exponential function, αeff = αex0
exp(−Hex/H0) + α0, to fit the αeff data. The α0 here corresponds to the αeff value at an infinite Hex, note that it still contains the extrinsic spin pumping and two-magnon scattering contributions. The fitted α0 are displayed in Fig. 2(e) as a function of Pd layer thickness. Interestingly, distinctly different from the non-monotonic variation behavior of Ku shown in Fig. 1(c), the α0 value keeps increasing with the increase of tPd, implying that there must be some other factors responsible for the observed increase in α0, particularly at tPd > 10 nm where the Ku starts to decrease. The Pd layer is known to own a short spin diffusion length around 10 nm, the extra damping induced by spin pumping effect will get saturated at tPd ∼ 10–15 nm.31 As we know, thicker Pd layer has a rougher surface, which gives rise to reduced exchange coupling between the interfacial magnetic atoms, as seen from the serious reduction of Ms and Ku shown in Fig. 1(c) and (d). The larger inhomogeneities and the resultant weaker exchange interaction at the Pd/FM interface, will surely enhance the two-magnon scattering effect and lead to a higher α0 value at thicker tPd case. In order to verify our assumption, the perpendicular coercivity Hc was also measured and plotted in Fig. 2(e) for comparison. The Hc and α0 should exhibit identical variation trends because a rough interface can not only give a thick paramagnetic dead layer, but can enhance the pinning effect of local magnetic moments as well.19,30 As expected, the increasing tendencies of α0 and Hc are very alike, indicating that the α0 increase may have the same origin as Hc, i.e. the increased PMA strength and underlayer surface roughness.
We then measured the magnetic damping of Pd/[Ni/Co]5 samples to further explore the underlying mechanism regarding the α0 enhancement with tPd. Fig. 3(a)–(c) shows the Hex dependences of precession frequency f, decay time τ, and the calculated effective damping factor αeff for tPd = 5, 15 and 30 nm, respectively. Their variation behaviors are very consistent with the case of Pd/[Co/Ni]5 structure, all the αeff values decrease with the increase of Hex, and aren't equal for different tPd at any applied field. The Pd thickness dependence of the extracted α0 is shown in Fig. 3(d), it also increases monotonically and coincides well with the perpendicular Hc, suggesting that the results are quite consistent.
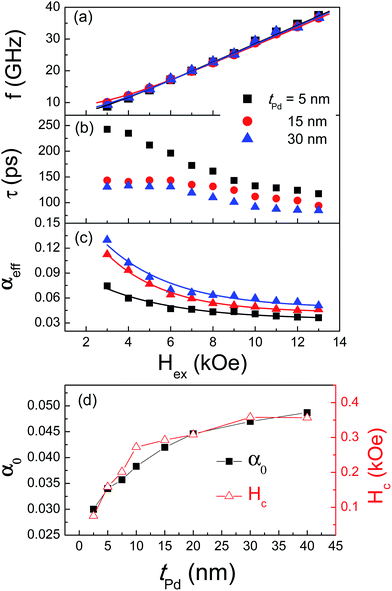 |
| Fig. 3 (a–c) corresponds to the magnetic field dependences of magnetization precession frequency f, decay time τ, and effective Gilbert damping αeff for the samples of Pd/[Ni/Co]5 with tPd = 5, 15 and 30 nm. (d) The extracted damping parameter α0 and the perpendicular magnetic coercivity Hc as a function of tPd. | |
In order to clearly compare the different influences of Pd underlayer, the PMA strength Ku, the inverse of saturation magnetization (1/Ms) and the saturated magnetic damping α0 are respectively shown in Fig. 4(a)–(c) for the two series samples of Ni/Co and Co/Ni. From the much higher Ku value displayed in Fig. 4(a), we know the Pd/[Co/Ni]5 sample has stronger spin orbit coupling at the Pd/Co interface, which in principle should provide a larger spin pumping contribution to the magnetic damping. But on the contrary, we find that the α0 value of Co/Ni is much lower than that of Ni/Co samples with the same Pd thickness, again revealing that it is the weakly-exchange coupled or even decoupled interfacial spins which play an important role in the observed additional increase in α0. The disordered spins can not only reduce the Ms, but also enhance the dissipation of spin energy via two-magnon scattering. In this sense, the 1/Ms and α0 may follow a similar variation trend. As expected, Fig. 4(b) and (c) indicates both of them increase gradually with the Pd layer thickness, since more paramagnetic disordered spins appear at the thicker Pd/Ni or Pd/Co interface.29,30,32 In contrast to the Co/Ni series, the Pd layer influences on the magnetic properties are more significant for Ni/Co samples, their 1/Ms and α0 values are relatively larger just because the exchange interaction between Ni atoms is rather weak and magnetic spins at the Pd/Ni interface are much more disordered.
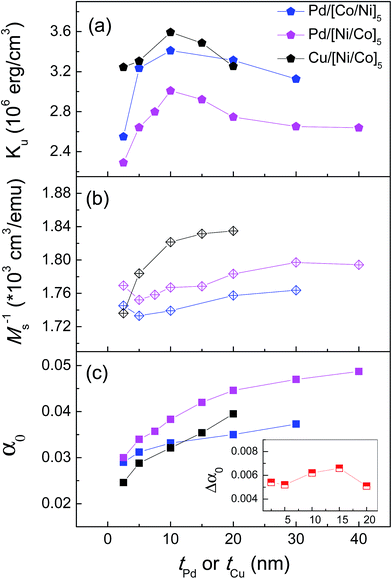 |
| Fig. 4 The uniaxial magnetic anisotropy energy density Ku, inverse of saturation magnetization (1/Ms) and magnetic damping constant α0 as a function of Pd or Cu underlayer thickness for the three types of samples. The inset of (c) shows the extra damping contribution Δα0 induced mainly by spin pumping effect of Pd layer as a function of the underlayer thickness. | |
Note that for the two types of Pd-buffered multilayer samples at the thinnest Pd thickness of 2.5 nm, their α0 values around 0.029 are the smallest and nearly equal. Nevertheless, such small value cannot be taken as the intrinsic damping constant, since the spin pumping effect of Pd layer is remarkable and not excluded. It is known the Cu layer has a quite longer spin diffusion length over 200 nm at RT, spin dephasing will not occur at the Cu thickness in a range of 0–20 nm, so the α0 increase induced by spin pumping of Cu can be neglected.33 As a result, in order to numerically determine the intrinsic damping and the spin pumping enhancement, we also measured and plotted the Ku and α0 of the Cu-buffered [Ni/Co]5 films in Fig. 4, with the same deposition layer sequence and sublayer thicknesses as those of Pd-buffered [Ni/Co]5 samples. As shown in Fig. 4(c), although there is no spin pumping contribution for the Cu-buffered sample, the α0 value also increases continuously with increasing tCu, owing to the increased thickness of interfacial magnetic dead layer as we discussed previously. Since the surface roughness of Cu buffer layer increases more rapidly with tCu than that of Pd buffer layer, a much faster and larger increase of 1/Ms for Cu-buffered [Ni/Co]5 samples can be found in Fig. 4(b). The observed increasing tendency of α0 for the Cu-buffered samples is nearly in parallel with that of Pd/[Ni/Co]5. However, their difference of α0, defined as Δα0, cannot be simply considered as the spin pumping contribution of Pd layer because the two-magnon scattering contribution of Cu is much serious than that of Pd. The inset in Fig. 4(c) shows the calculated Δα0 versus tPd, it increases very slightly and even drops at tPd = 20 nm, where the spin pumping enhancement is already saturated. This variation behavior is just because the Cu layer has a rougher surface than the Pd with the same thickness,12 which gives a more prominent two-magnon scattering contribution. For the samples with a very thin Pd or Cu under layer, the roughness difference is not so big, so the Δα0 value of 0.0055 at tPd = 2.5 nm can be approximately taken as the spin pumping contribution of a 2.5 nm thick Pd layer. Accordingly, the intrinsic magnetic damping constant of our perpendicular [Ni/Co]5 multilayer is estimated to be around 0.023.
Conclusion
In summary, we have studied the Pd underlayer influences on the magnetic damping, saturation magnetization and perpendicular magnetic anisotropy of [Co/Ni]5 and [Ni/Co]5 multilayers by time-resolved magneto-optical Kerr effect. It is found the Ku value firstly rises with tPd and then decreases after showing a maximum at tPd = 10 nm for both kinds of samples, owing to the competition between the roles of fcc (111) texture and magnetic dead layer at Pd/Co (or Ni) interface which are considered also responsible for the gradual reduction of Ms and monotonic increase of α0. Interestingly, the Pd/[Ni/Co]5 sample owns obviously smaller Ku and Ms but rather higher α0, which is attributed to the presence of more disordered paramagnetic spins at Pd/Ni interface. By comparing the α0 of Pd- and Cu-buffered [Ni/Co]5 samples at t = 2.5 nm, the spin pumping contribution of Pd layer and intrinsic damping of perpendicular [Ni/Co]5 are quantitively determined to be ∼0.0055 and 0.023, respectively. These results may be helpful to understand the reported different relationships between PMA and magnetic damping, and to promise potential practical applications in high-performance SOT-memories.
Acknowledgements
This work is supported by the National Basic Research Program of China (No. 2014CB921104) and the National Natural Science Foundation of China (Grant No. 51671057, 11474067, 51222103, 11274113, 11674095 and 51201081).
References
- S. Mangin, D. Ravelosona, J. A. Katine, M. J. Carey, B. D. Terris and E. E. Fullerton, Current-induced magnetization reversal in nanopillars with perpendicular anisotropy, Nat. Mater., 2006, 5, 210–215 CrossRef CAS.
- X. Li, Z. Zhang, Q. Y. Jin and Y. Liu, Domain nucleation mediated spin-transfer switching in magnetic nanopillars with perpendicular anisotropy, Appl. Phys. Lett., 2008, 92, 122502 CrossRef.
- A. Capua, S. H. Yang, T. Phung and S. S. P. Parkin, Determination of intrinsic damping of perpendicularly magnetized ultrathin films from time-resolved precessional magnetization measurements, Phys. Rev. B: Condens. Matter Mater. Phys., 2015, 92, 224402 CrossRef.
- S. Mangin, Y. Henry, D. Ravelosona, J. A. Katine and E. E. Fullerton, Reducing the critical current for spin-transfer switching of perpendicularly magnetized nanomagnets, Appl. Phys. Lett., 2009, 94, 012502 CrossRef.
- H. S. Song, K. D. Lee, J. W. Sohn, S. H. Yang, S. S. P. Parkin, C. Y. You and S. Shin, Relationship between Gilbert damping and magneto-crystalline anisotropy in a Ti-buffered Co/Ni multilayer system, Appl. Phys. Lett., 2013, 103, 022406 CrossRef.
- S. Pal, B. Rana, O. Hellwig, T. Thomson and A. Barman, Tunable magnetic frequency and damping in [Co/Pd]8 multilayers with variable Co layer thickness, Appl. Phys. Lett., 2011, 98, 082501 CrossRef.
- P. He, X. Ma, J. W. Zhang, H. B. Zhao, G. Lüpke, Z. Shi and S. M. Zhou, Quadratic Scaling of Intrinsic Gilbert Damping with Spin-Orbital Coupling in L10 FePdPt Films: Experiments and Ab Initio Calculations, Phys. Rev. Lett., 2013, 110, 077203 CrossRef CAS PubMed.
- S. Mizukami, E. P. Sajitha, D. Watanabe, F. Wu, T. Miyazaki, H. Naganuma, M. Oogane and Y. Ando, Gilbert damping in perpendicularly magnetized Pt/Co/Pt films investigated by all-optical pump-probe technique, Appl. Phys. Lett., 2010, 96, 152502 CrossRef.
- S. Iihama, S. Mizukami, H. Naganuma, M. Oogane, Y. Ando and T. Miyazaki, Gilbert damping constants of Ta/CoFeB/MgO(Ta) thin films measured by optical detection of precessional magnetization dynamics, Phys. Rev. B: Condens. Matter Mater. Phys., 2014, 89, 174416 CrossRef.
- A. Barman, S. Wang, O. Hellwig, A. Berger, E. E. Fullerton and H. Schmidt, Ultrafast magnetization dynamics in high perpendicular anisotropy [Co/Pt]n multilayers, J. Appl. Phys., 2007, 101, 09D102 CrossRef.
- A. Y. Dobin and R. H. Victora, Surface Roughness Induced Extrinsic Damping in Thin Magnetic Films, Phys. Rev. Lett., 2004, 92, 257204 CrossRef PubMed.
- S. Chen, M. Tang, Z. Zhang, B. Ma, S. T. Lou and Q. Y. Jin, Interfacial effect on the ferromagnetic damping of CoFeB thin films with different under-layers, Appl. Phys. Lett., 2013, 103, 032402 CrossRef.
- T. Nan, S. Emori, C. T. Boone, X. Wang, T. M. Oxholm, J. G. Jones, B. M. Howe, G. J. Brown and N. X. Sun, Comparison of spin-orbit torques and spin pumping across NiFe/Pt and NiFe/Cu/Pt interfaces, Phys. Rev. B: Condens. Matter Mater. Phys., 2015, 91, 214416 CrossRef.
- S. Mizukami, Y. Ando and T. Miyazaki, The Study on Ferromagnetic Resonance Line width for NM/80NiFe/NM (NM = Cu, Ta, Pd and Pt) Films, Jpn. J. Appl. Phys., 2001, 40, 580–585 CrossRef CAS.
- S. Azzawi, A. Ganguly, M. Tokaç, R. M. Rowanrobinson, J. Sinha, A. T. Hindmarch, A. Barman and D. Atkinson, Evolution of damping in ferromagnetic/nonmagnetic thin film bilayers as a function of nonmagnetic layer thickness, Phys. Rev. B, 2016, 93, 054402 CrossRef.
- M. H. Tang, Z. Zhang, S. Tian, J. Wang, B. Ma and Q. Y. Jin, Interfacial exchange coupling and magnetization reversal in perpendicular [Co/Ni]N/TbCo composite structures, Sci. Rep., 2015, 5, 10863 CrossRef CAS PubMed.
- H. S. Song, K. D. Lee, J. W. Sohn, S. H. Yang, S. S. P. Parkin, C. Y. You and S. C. Shin, Observation of the intrinsic Gilbert damping constant in Co/Ni multilayers independent of the stack number with perpendicular anisotropy, Appl. Phys. Lett., 2013, 102, 102401 CrossRef.
- D. Wu, S. Chen, Z. Zhang, B. Ma and Q. Y. Jin, Enhancement of perpendicular magnetic anisotropy in Co/Ni multilayers by in situ annealing the Ta/Cu under-layers, Appl. Phys. Lett., 2013, 103, 242401 CrossRef.
- H. Kurt, M. Venkatesan and J. M. D. Coey, Enhanced perpendicular magnetic anisotropy in Co/Ni multilayers with a thin seed layer, J. Appl. Phys., 2010, 108, 073916 CrossRef.
- A. V. D. Brink, G. Vermijs, A. Solignac, J. Koo, J. T. Kohlhepp, H. J. M. Swagten and B. Koopmans, Field-free magnetization reversal by spin-Hall effect and exchange bias, Nat. Commun., 2016, 7, 10854 CrossRef PubMed.
- P. P. J. Haazen, E. Murè, J. H. Franken, R. Lavrijsen, H. J. M. Swagten and B. Koopmans, Domain wall depinning governed by the spin Hall effect, Nat. Mater., 2013, 12, 299–303 CrossRef CAS PubMed.
- L. Liu, C. F. Pai, Y. Li, H. W. Tseng, D. C. Ralph and R. A. Buhrman, Spin-Torque Switching with the Giant Spin Hall Effect of Tantalum, Science, 2012, 336, 555–558 CrossRef CAS PubMed.
- K. L. Wang, J. G. Alzate and P. K. Amiri, Low-power non-volatile spintronic memory: STT-RAM and beyond, J. Phys. D: Appl. Phys., 2013, 6, 074003 CrossRef.
- T. Jungwirth, J. Wunderlich and K. Olejnik, Spin Hall effect devices, Nat. Mater., 2012, 11, 382–390 CrossRef CAS PubMed.
- A. Barman and A. Haldar, Time-Domain Study of Magnetization Dynamics in Magnetic Thin Films and Micro- and Nanostructures, Solid State Phys., 2014, 65, 1–108 Search PubMed.
- D. Wu, W. Li, M. H. Tang, Z. Z. Zhang, S. T. Lou and Q. Y. Jin, Laser heating and oxygen partial pressure effects on the dynamic magnetic properties of perpendicular CoFeAlO films, J. Magn. Magn. Mater., 2016, 409, 143–147 CrossRef CAS.
- E. P. Sajitha, J. Walowski, D. Watanabe, S. Mizukami, F. Wu, H. Naganuma, M. Oogane, Y. Ando and T. Miyazaki, Magnetization Dynamics in CoFeB Buffered Perpendicularly Magnetized Co/Pd Multilayer, IEEE Trans. Magn., 2010, 46, 2056–2059 CrossRef CAS.
- G. Nabiyouni and B. J. Farahani, Anomalous scaling in surface roughness evaluation of electrodeposited nanocrystalline Pt thin films, Appl. Surf. Sci., 2009, 256, 674–682 CrossRef CAS.
- S. Kim, J. Jeong, J. B. Kortright and S. Shin, Experimental observation of magnetically dead layers in Ni/Pt multilayer films, Phys. Rev. B: Condens. Matter Mater. Phys., 2001, 64, 052406 CrossRef.
- J. Kim, J. W. Lee, J. R. Jeong, S. K. Kim and S. C. Shin, Influence of substrate roughness on spin reorientation transition of ultrathin Co films on Pd(111), Appl. Phys. Lett., 2001, 79, 93 CrossRef CAS.
- J. M. Shaw, H. T. Nembach and T. J. Silva, Determination of spin pumping as a source of linewidth in sputtered Co90Fe10/Pd multilayers by use of broadband ferromagnetic resonance spectroscopy, Phys. Rev. B: Condens. Matter Mater. Phys., 2012, 85, 054412 CrossRef.
- N. Sato, K. P. O'Brien, K. Millard, B. Doyle and K. Oguz, Investigation of extrinsic damping caused by magnetic dead layer in Ta–CoFeB–MgO multilayers with perpendicular anisotropy, J. Appl. Phys., 2016, 119, 093902 CrossRef.
- T. Gerrits, M. L. Schneider and T. J. Silva, Enhanced ferromagnetic damping in Permalloy/Cu bilayers, J. Appl. Phys., 2006, 99, 023901 CrossRef.
|
This journal is © The Royal Society of Chemistry 2017 |
Click here to see how this site uses Cookies. View our privacy policy here.