DOI:
10.1039/C6RA27307F
(Paper)
RSC Adv., 2017,
7, 3888-3893
Photocatalyzed iron-based ATRP of methyl methacrylate using 1,3-dimethyl-2-imidazolidinone as both solvent and ligand
Received
25th November 2016
, Accepted 30th November 2016
First published on 16th January 2017
Abstract
Photochemistry serves as a wonderful means to facilitate various chemical reactions and is indeed unique in its powerful ability to meet the energetic requirements for conducting processes that would not be accomplished using thermal counterparts. In this work, a novel photocatalyzed Fe-based atom transfer radical polymerization (ATRP) of methyl methacrylate (MMA) was performed under UV irradiation, using ethyl 2-bromophenylacetate (EBPA) as the initiator, FeBr2 as the catalyst and 1,3-dimethyl-2-imidazolidinone (DMI) as both the solvent and ligand. The rate of the polymerization was relatively fast, as monomer conversion reached 91.2% at room temperature under UV irradiation (900 μW cm−2) at 360 nm within 26 h. Even when the target degree of polymerization was up to 1000, the molecular weight distribution obtained remained narrow and the molecular weight (Mn,GPC) was close to the corresponding theoretical value (Mn,th). The polymerization kinetics was studied in detail and the “living” features of this system were confirmed by performing successful chain extension experiments.
1. Introduction
Since its discovery, reversible deactivation radical polymerization (RDRP)1 has been used to synthesize well-defined polymers with various complex architectures. Atom transfer radical polymerization (ATRP),2 as one of the most widely used methods of RDRP, has gained more intensive attention. In addition to normal ATRP, several heterogeneous ATRP reactions have been reported to date, such as reverse ATRP,3 activators generated by electron transfer (AGET) ATRP,4 initiators for continuous activator regeneration (ICAR) ATRP,5 and the very recently developed photo-induced metal-free ATRP.6 The key process in ATRP is the reversible redox reaction between the alkyl halide and the active catalysts. Taking a metal complex as an example, a low oxidation transition metal complex (activator) reacts with the initiator, and generates an active radical and a corresponding high oxidation metal complex (deactivator). The active radical acts on the monomer and forms a propagating radical, and therefore the propagating radical continuously proliferates until it has been deactivated by the deactivator. The polymerization process can be controlled due to the dynamic equilibrium of the redox reaction between the low and high oxidation transition metal complexes, thus maintaining a low concentration of propagating radicals in the polymerization system.
Many metal catalysts can be applied in ATRP, the most common metals being Cu,7 Ni,8 Ru7a,b and Fe.9 Compared to other metal catalysts, Fe-based catalysts have many attractive characteristics. Iron is the most plentiful metal on earth and possesses a low toxicity. Therefore, it is not limited in large-scale industrial production, especially in the field of biomedical materials. Typical ligands used in iron catalysts are phosphorus-containing ligands,10 nitrogen-containing ligands,9c,11 organic acids12 and polyethylene glycols.13 In addition, some polar solvents can also be employed as the ligand in Fe-based ATRP systems.14
Photochemistry serves as a wonderful technique to enable various chemical reactions and is indeed unique in its powerful ability to meet the energetic requirements for conducting processes that would not be accomplished using thermal counterparts. Recently, photo-induced polymerization has attracted the attention of a large number of scientists, since the ability to perform photochemical reactions under mild conditions may overcome certain problems, such as the exorbitant temperature required in thermal polymerization. In 2012, Hawker's group reported a living radical polymerization through external visible light stimulation using Ir(ppy)3 as the catalyst.15 In the same year, Matyjaszewski's group reported a photo-induced ATRP using a Cu complex as the catalyst.16 In 2015, a simple photo-induced Fe-based ATRP was reported by Matyjaszewski's group.17 However, the hyper-toxic solvent (MeCN) used in the polymerization reaction makes the whole system imperfect. Furthermore, the polymerization rate was a little slow (monomer conversion was less than 60% after polymerization for 28 h) and the molecular weights were lower than the theoretical values, especially in the later stages of the polymerization reaction.
We know that 1,3-dimethyl-2-imidazolidinone (DMI)18 is an aprotic polar solvent due to the strong electron-withdrawing effect of the carbonyl group. It exhibits low toxicity and is nearly harmless to the human body. Moreover, it exhibits good solubility and excellent stability, and has therefore been used widely in chemical synthesis.
Herein, the “green” polar solvent 1,3-dimethyl-2-imidazolidinone (DMI) was employed both as the solvent and ligand (without any other additional ligands) in a photo-induced Fe-based ATRP at room temperature, using FeBr2 as the catalyst, ethyl 2-bromophenylacetate (EBPA) as the initiator and methyl methacrylate (MMA) as the model monomer under UV irradiation (900 μW cm−2). The polymerization system exhibited a relatively fast rate of polymerization and could be controlled well, even under a 1000 target degree of polymerization.
2. Experimental section
2.1 Materials
The monomer, methyl methacrylate (MMA, +99%), was purchased from Shanghai Chemical Reagents Co. Ltd (Shanghai, China). It was purified via removing the inhibitor by passing it through a short neutral alumina column before use. The following materials were used as received: ethyl 2-bromophenylacetate (EBPA, 97%) purchased from J&K Scientific Ltd, tetrahydrofuran (THF, analytical reagent) purchased from Nanjing Chemical Reagent Co. Ltd, 1,3-dimethyl-2-imidazolidinone (DMI, 98%), iron(II) bromide (98%) and triphenylphosphine (PPh3, 98%) purchased from Energy Chemical, iron(III) acetylacetonate (Fe(acac)3, 99.95%) purchased from Aldrich (Shanghai, China), toluene (analytical reagent) purchased from Chinasun Specialty Products Co. Ltd, and ascorbic acid (AsAc) purchased from Shanghai Chemical Reagents Co. Ltd (Shanghai, China).
2.2 Characterization
The number-average molecular weight (Mn,GPC) and molecular weight distribution (Mw/Mn) values of the resultant polymers were determined by employing a TOSOH HLC-8320 gel permeation chromatograph (GPC) equipped with a refractive-index detector (TOSOH), using TSKgel guardcolumn SuperMP-N (4.6 × 20 mm) and two TSKgel SupermultiporeHZ-N (4.6 × 150 mm) with a measurable molecular weight range of 5 × 102 to 5 × 105 g mol−1. THF was used as the eluent at a flow rate of 0.35 mL min−1 and 40 °C. GPC samples were injected using a TOSOH plus autosampler and calibrated with PMMA standards purchased from TOSOH. Matrix assisted laser desorption/ionization time-of-flight mass (MALDI-TOF-MS) spectra were recorded using an UltrafleXtreme MALDI-TOF mass spectrometer equipped with a 1000 Hz smart beam-II laser. Fluorescence emission spectra were obtained using a quart cuvette on a Hitachi F-4600 fluorescence spectrophotometer at room temperature. The 1H NMR spectrum of the obtained polymers was recorded on a Bruker 300 MHz nuclear magnetic resonance (NMR) instrument using deuterated dimethyl sulphoxide (DMSO-d6) as the solvent and tetramethylsilane (TMS) as the internal standard at ambient temperature.
2.3 General procedure for photo-induced Fe-based ATRP
A typical polymerization procedure for the system with a molar ratio of [MMA]0
:
[EBPA]0
:
[FeBr2]0 = 100
:
1
:
1 is as follows. A mixture was obtained by adding FeBr2 (20.4 mg, 9.4 × 10−5 mol), MMA (1.0 mL, 9.4 × 10−3 mol), EBPA (16.6 μL, 9.4 × 10−5 mol), DMI (0.5 mL) and a magnetic stirring bar to a clean ampoule. The mixture was thoroughly bubbled with argon for 20 min to eliminate the dissolved oxygen in the reaction system, and was then flame-sealed. The polymerization reaction was carried out at room temperature under UV irradiation (900 μW cm−2) at 360 nm, and the polymerization temperature was maintained through controlled fanning. After the desired polymerization time, the ampoule was opened and the contents were dissolved in a certain amount of tetrahydrofuran (THF). The resultants were precipitated by adding the solution to a large amount of hexane (∼250 mL). The polymers obtained by filtration were dried under vacuum until they reached a constant weight at 30 °C. The monomer conversion was determined gravimetrically.
2.4 Chain extension of the resultant PMMA
A predetermined quantity of PMMA, obtained via the photo-induced Fe-based ATRP of MMA, was added to a clean ampoule, and then predetermined quantities of MMA (1.0 mL), FeBr2 (20.4 mg) and DMI (0.5 mL) were added. The mixture was thoroughly bubbled with argon for 20 min to eliminate the dissolved oxygen in the reaction system, and was then flame-sealed. The polymerization was performed under UV irradiation (900 μW cm−2) at 360 nm at room temperature. The rest of the procedure was the same as that for the polymerization of MMA described above. In addition, for the chain extension via the thermal polymerization method, a predetermined quantity of PMMA, obtained via the photo-induced Fe-based ATRP of MMA, was added to a clean ampoule, and then predetermined quantities of MMA (1.0 mL), toluene (1.0 mL), Fe(acac)3 (17.0 mg), PPh3 (37.0 mg) and AsAc (66.8 mg) were added. The mixture was thoroughly bubbled with argon for 20 min to eliminate the dissolved oxygen in the reaction system, and was then flame-sealed. Afterwards, it was transferred into an oil bath maintained at the desired temperature (90 °C) using a thermostat, to polymerize under stirring. After the desired polymerization time, the ampoule was placed into ice water to cool down. The rest of the procedure was the same as that for the polymerization of MMA described above.
3. Results and discussion
3.1 Effect of the amount of Fe catalyst
Firstly, we investigated the effect of the amount of FeBr2 on the polymerization of MMA, and the results are shown in Table 1. As the concentration of FeBr2 decreased (entries 1–4 in Table 1), the molecular weight distribution (Mw/Mn) became broader and the molecular weight (Mn,GPC) deviated from its corresponding theoretical value (Mn,th) gradually, indicating that the controllability of the polymerization system decreased correspondingly. When no FeBr2 was added, the polymerization exhibited loss of control, as the molecular weight distribution reached up to 2.07 and the Mn,GPC deviated significantly from Mn,th. In this polymerization system, DMI acted as both the ligand and the solvent, so the volume of DMI should have a significant influence on the polymerization. From Table 1, we can see that when the volume of DMI was relatively low (0.2 mL), the rate of the polymerization was relatively slow. When the amount of DMI was increased to more than 0.8 mL, the controllability of the polymerization was not desirable (entries 7–9 in Table 1). When VDMI = 0.5 mL, the polymerization could be controlled well (entry 1 in Table 1). When no DMI was added, the molecular weight distribution reached up to 2.00, which meant that the whole polymerization system was not controlled (entry 6 in Table 1). It is worth noting that even though no EBPA was added, the polymerization could still proceed (entry 10 in Table 1) because the monomer (MMA) can react with the iron catalyst and generate MMA-Br, which acts as an initiator in the polymerization system.17 However, the molecular weight (111
000 g mol−1) was uncontrollable in this case. Furthermore, when the experiment was conducted without irradiation, no polymer was found after 24 hours (entry 11 in Table 1), indicating that UV irradiation plays an important role in the polymerization system.
Table 1 Effect of the amount of the Fe-based catalyst on the photo-induced ATRP of MMAa
Entry |
x |
VDMI (mL) |
Time (h) |
Conv. (%) |
Mn,thb (g mol−1) |
Mn,GPC (g mol−1) |
Mw/Mn |
Polymerization conditions: R = [MMA]0 : [EBPA]0 : [FeBr2]0 = 100 : 1 : x, VMMA = 1.0 mL, at room temperature under UV irradiation (900 μW cm−2) at 360 nm. Mn,th = ([M]0/[I]0) × Mn,MMA × conv. % + Mn,EBPA. No EBPA was added. Without irradiation. |
1 |
1.0 |
0.5 |
26 |
91.2 |
9400 |
9500 |
1.30 |
2 |
0.5 |
0.5 |
24 |
70.2 |
7200 |
8000 |
1.74 |
3 |
0.2 |
0.5 |
24 |
99.9 |
10 200 |
14 100 |
2.27 |
4 |
0.1 |
0.5 |
24 |
99.9 |
10 200 |
13 200 |
1.67 |
5 |
0 |
0.5 |
24 |
99.9 |
20 200 |
70 100 |
2.07 |
6 |
1.0 |
0 |
24 |
34.0 |
3600 |
7700 |
2.00 |
7 |
1.0 |
0.2 |
30 |
52.1 |
5400 |
4800 |
1.28 |
8 |
1.0 |
0.8 |
30 |
72.1 |
7400 |
7000 |
1.52 |
9 |
1.0 |
1.0 |
30 |
92.4 |
9500 |
8200 |
1.46 |
10c |
1.0 |
0.5 |
30 |
31.5 |
— |
111 000 |
1.43 |
11d |
1.0 |
0.5 |
24 |
0 |
— |
— |
— |
3.2 Effect of the amount of initiator on polymerization
In order to investigate the effect of polymerization degree, we kept the amount of MMA, FeBr2 and the solvent constant, but changed the amount of initiator to conduct the polymerization. The results are shown in Table 2. It can be seen that even when the target degree of polymerization was up to 1000 (x = 0.1, entry 3 in Table 2), the resultant molecular weight distribution was still narrow (Mw/Mn = 1.28) and Mn,GPC (40
800 g mol−1) was close to its corresponding Mn,th (35
000 g mol−1), indicating kind controllability of the polymerization system. Therefore, this method could be employed to synthesized polymers with relatively high molecular weight.
Table 2 Effect of the amount of initiator on the photo-induced ATRP of MMAa
Entry |
x |
Conv. (%) |
Mn,thb (g mol−1) |
Mn,GPC (g mol−1) |
Mw/Mn |
Polymerization conditions: R = [MMA]0 : [EBPA]0 : [FeBr2]0 = 100 : x : 1, VMMA = 1.0 mL, at room temperature under UV irradiation (900 μW cm−2) at 360 nm, time = 24 h. Mn,th = ([M]0/[I]0) × Mn,MMA × conv. % + Mn,EBPA. |
1 |
0.5 |
57.5 |
11 700 |
11 600 |
1.28 |
2 |
0.25 |
48.9 |
19 800 |
20 300 |
1.28 |
3 |
0.1 |
40.6 |
40 800 |
35 000 |
1.28 |
3.3 Polymerization kinetics of photo-induced Fe-based ATRP of MMA
In order to deeply investigate the polymerization behavior, the polymerization kinetics of the system with the molar ratio of [MMA]0
:
[EBPA]0
:
[FeBr2]0 = 100
:
1
:
1 was conducted at room temperature under UV irradiation (900 μW cm−2) at 360 nm. The first-order curve consisting of time and ln([M]0/[M]), shown in Fig. 1A, indicates that the propagation radical concentration remained approximately constant during polymerization. Furthermore, the molecular weights (Mn,GPCs) were close to the corresponding theoretical values (Mn,ths) and increased linearly with the monomer conversion, while maintaining narrow molecular weight distributions at the same time (Fig. 1B). Moreover, the GPC elution curves (Fig. 1C) show a distinct shift from low molecular weights to high molecular weights during the polymerization. All of these results confirmed the “living” features of this photo-induced Fe-based ATRP system. In addition, the possible polymerization mechanism of photo-induced Fe-based ATRP is shown in Scheme 1, which is based a reversible dynamic equilibrium between the active Fe(II) species and the deactivator Fe(III) species under irradiation of UV light.
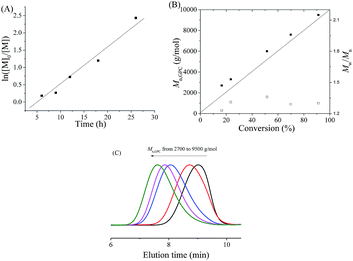 |
| Fig. 1 ln([M]0/[M]) as a function of time (A); evolution of number-average molecular weight (Mn,GPC) and molecular weight distribution (Mw/Mn) versus conversion (B); GPC elution curves (C) for Fe-based photo-induced ATRP of MMA. Polymerization conditions: [MMA]0 : [EBPA]0 : [FeBr2]0 = 100 : 1 : 1, VMMA = 1.0 mL, VDMI = 0.5 mL, at room temperature under UV irradiation (900 μW cm−2) at 360 nm. | |
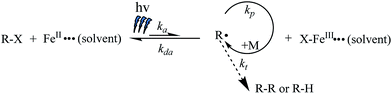 |
| Scheme 1 Possible polymerization mechanism of photo-induced Fe-based ATRP under irradiation of UV light. | |
3.4 Analysis of chain-end and chain extension
The chain-end of the resultant PMMA was analyzed by 1H NMR spectroscopy in DMSO-d6. The chemical shifts at δ = 0.5–2.0 ppm (d in Fig. 2A) were assigned to methylene group in PMMA and methyl group in PMMA and the initiator moieties of EBPA. The chemical shifts at δ = 4.0 ppm (c in Fig. 2A) and 7.2–7.4 ppm (b in Fig. 2A) were attributed to the methylene and phenyl groups in the initiator moieties of EBPA. The chemical shift at δ = 3.6 ppm (a in Fig. 2A) was assigned to the methyl ester groups in PMMA. The signal at δ = 3.78 ppm (a* in Fig. 2A) was assigned to methyl ester groups at the chain-end. Further, MALDI-TOF MS was conducted to analyze the structure of PMMA. As shown in Fig. 2B, the main series of peaks was assigned to PMMA with EBPA moieties attached to the end. The interval (100.201 of m/z) between every two peaks represents the MMA unit.
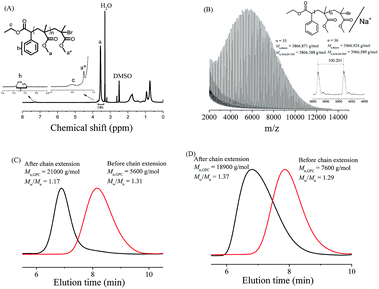 |
| Fig. 2 1H NMR spectrum (A) and MALDI-TOF MS (B) of the resultant PMMA. Polymerization conditions of sample PMMA (Mn,GPC = 6900 g mol−1, Mn,NMR = 6400 g mol−1, Mw/Mn = 1.35): [MMA]0 : [EBPA]0 : [FeBr2]0 = 100 : 1 : 1, VMMA = 1.0 mL, VDMI = 0.5 mL, time = 15 h, at room temperature under UV irradiation (900 μW cm−2) at 360 nm. GPC traces of PMMA before and after chain extension under both photo-induced (C) and thermal (D) conditions. Chain extension conditions via photochemical reaction: [MMA]0 : [PMMA]0 : [FeBr2]0 = 100 : 0.4 : 1, VMMA = 1.0 mL, VDMI = 0.5 mL, time = 54 h, at room temperature under UV irradiation (900 μW cm−2) at 360 nm. Chain extension conditions via thermal polymerization reaction: [MMA]0 : [PMMA]0 : [Fe(acac)3]0 :[PPh3]0 : [AsAc]0 = 100 : 0.4 : 1 : 3 : 4, VMMA = 1.0 mL, Vtoluene = 1.0 mL, T = 70 °C, time = 24 h. | |
Finally, chain extension experiments were conducted to verify the living features of the photo-induced Fe-based ATRP of MMA. Firstly, we conducted a chain extension experiment using the resultant PMMA as the macroinitiator (Mn,GPC = 5600 g mol−1, Mw/Mn = 1.31) to carry out the photo-induced Fe-based ATRP of MMA. As shown in Fig. 2C, the Mn,GPC increased from 5600 g mol−1 to 21
000 g mol−1 after chain extension. Secondly, a thermal AGET ATRP of MMA was conducted using the resultant PMMA as the macroinitiator (Mn,GPC = 7600 g mol−1, Mw/Mn = 1.29). As shown in Fig. 2D, the Mn,GPC increased from 7600 g mol−1 to 18
900 g mol−1 after chain extension. These two chain extension experiments indicate that the PMMA obtained via the photo-induced Fe-based ATRP had a high degree of chain-end functionality.
4. Conclusions
A highly efficient photo-induced Fe-based ATRP of MMA was developed using “green” DMI as both the ligand and solvent without any additional ligands. The rate of the polymerization was relatively fast and well controlled polymers could be facilely obtained.
Acknowledgements
The financial support from the National Natural Science Foundation of China (No. 21174096, 21674071), the Project of Science and Technology Development Planning of Jiangsu Province (No. BK20141192) and the Project Funded by the Priority Academic Program Development of Jiangsu Higher Education Institutions (PAPD) are gratefully acknowledged.
Notes and references
-
(a) W. A. Braunecker and K. Matyjaszewski, Prog. Polym. Sci., 2007, 32, 93 CrossRef CAS;
(b) A. Goto and T. Fukuda, Prog. Polym. Sci., 2004, 29, 329 CrossRef CAS;
(c) K. Matyjaszewski and T. P. Davis, Handbook of Radical Polymerization, John Wiley & Sons, Inc., Hoboken, NJ, 2002 CrossRef;
(d) R. Poli, L. E. N. Allan and M. P. Shaver, Prog. Polym. Sci., 2014, 39, 1827 CrossRef CAS;
(e) V. Sciannamea, R. Jerome and C. Detrembleur, Chem. Rev., 2008, 108, 1104 CrossRef CAS PubMed;
(f) T. Öztürk, M. N. Atalar, M. Göktaş and B. Hazer, J. Polym. Sci.: Part A: Polym. Chem., 2013, 51, 2651 CrossRef.
-
(a) M. Kato, M. Kamigaito, M. Sawamoto and T. Higashimura, Macromolecules, 1995, 28, 1721 CrossRef CAS;
(b) J. S. Wang and K. Matyjaszewski, J. Am. Chem. Soc., 1995, 117, 5614 CrossRef CAS;
(c) K. Matyjaszewski and J. H. Xia, Chem. Rev., 2001, 101, 2921 CrossRef CAS PubMed;
(d) M. Ouchi, T. Terashima and M. Sawamoto, Chem. Rev., 2009, 109, 4963 CrossRef CAS PubMed;
(e) M. F. Cunningham, Prog. Polym. Sci., 2008, 33, 365 CrossRef CAS;
(f) W. W. He, H. J. Jiang, L. F. Zhang, Z. P. Cheng and X. L. Zhu, Polym. Chem., 2013, 4, 2919 RSC;
(g) L. J. Bai, L. F. Zhang, Z. P. Cheng and X. L. Zhu, Polym. Chem., 2012, 3, 2685–2697 RSC;
(h) H. Arslan, N. Yeşilyurt and B. Hazer, J. Appl. Polym. Sci., 2007, 106, 1742 CrossRef CAS;
(i) T. Öztürk, S. S. Yilmaz, B. Hazer and Y. Z. Menceloglu, J. Polym. Sci.: Part A: Polym. Chem., 2010, 48, 1364 CrossRef.
-
(a) D. Q. Qin, S. H. Qin, X. P. Chen and K. Y. Qiu, Polymer, 2000, 41, 7347 CrossRef CAS;
(b) J. S. Wang and K. Matyjaszewski, Macromolecules, 1995, 28, 7572 CrossRef CAS;
(c) Y. P. Wang, X. W. Pei, X. Y. He and K. Yuan, Eur. Polym. J., 2005, 41, 1326 CrossRef CAS;
(d) Z. P. Cheng, X. L. Zhu, G. J. Chen, W. J. Xu and J. M. Lu, J. Polym. Sci., Part A: Polym. Chem., 2002, 40, 3823 CrossRef CAS;
(e) Z. P. Cheng, X. L. Zhu, L. F. Zhang, N. C. Zhou and J. Y. Chen, J. Macromol. Sci., Part A: Pure Appl. Chem., 2003, A40, 371 CrossRef CAS;
(f) G. H. Zhu, L. F. Zhang, X. Q. Pan, W. Zhang, Z. P. Cheng and X. L. Zhu, Macromol. Rapid Commun., 2012, 33, 2121 CrossRef CAS PubMed.
-
(a) L. J. Bai, L. F. Zhang, J. L. Pan, J. Zhu, Z. P. Cheng and X. L. Zhu, Macromolecules, 2013, 46, 2060 CrossRef CAS;
(b) W. Jakubowski and K. Matyjaszewski, Macromolecules, 2005, 38, 4139 CrossRef CAS;
(c) L. Mueller, W. Jakubowski, K. Matyjaszewski, J. Pietrasik, P. Kwiatkowski, W. Chaladaj and J. Jurczak, Eur. Polym. J., 2011, 47, 730 CrossRef CAS.
-
(a) T. Guo, L. F. Zhang, X. Q. Pan, X. H. Li, Z. P. Cheng and X. L. Zhu, Polym. Chem., 2013, 4, 3725 RSC;
(b) K. Mukumoto, Y. Wang and K. Matyjaszewski, ACS Macro Lett., 2012, 1, 599 CrossRef CAS;
(c) L. F. Zhang, J. Miao, Z. P. Cheng and X. L. Zhu, Macromol. Rapid Commun., 2010, 31, 275 CrossRef CAS PubMed;
(d) G. H. Zhu, L. F. Zhang, Z. B. Zhang, J. Zhu, Y. F. Tu, Z. P. Cheng and X. L. Zhu, Macromolecules, 2011, 44, 3233 CrossRef CAS;
(e) X. W. Jiang, J. Wu, L. F. Zhang, Z. P. Cheng and X. L. Zhu, Macromol. Rapid Commun., 2014, 35, 1879 CrossRef CAS PubMed;
(f) B. J. Zhang, X. W. Jiang, L. F. Zhang, Z. P. Cheng and X. L. Zhu, Polym. Chem., 2015, 6, 6616 RSC;
(g) X. W. Jiang, L. F. Zhang, Z. P. Cheng and X. L. Zhu, Macromol. Rapid Commun., 2016, 37, 1337 CrossRef CAS PubMed.
-
(a) N. J. Treat, H. Sprafke, J. W. Kramer, P. G. Clark, B. E. Barton, J. R. de Alaniz, B. P. Fors and C. J. Hawker, J. Am. Chem. Soc., 2014, 136, 16096 CrossRef CAS PubMed;
(b) G. M. Miyake and J. C. Theriot, Macromolecules, 2014, 47, 8255 CrossRef CAS;
(c) X. C. Pan, M. Lamson, J. J. Yan and K. Matyjaszewski, ACS Macro Lett., 2015, 4, 192 CrossRef CAS;
(d) X. Pan, C. Fang, M. Fantin, N. Malhotra, W. Y. So, L. A. Peteanu, A. A. Isse, A. Gennaro, P. Liu and K. Matyjaszewski, J. Am. Chem. Soc., 2016, 138, 2411 CrossRef CAS PubMed;
(e) J. C. Theriot, C. H. Lim, H. Yang, M. D. Ryan, C. B. Musgrave and G. M. Miyake, Science, 2016, 352, 1082 CrossRef CAS PubMed;
(f) X. D. Liu, L. F. Zhang, Z. P. Cheng and X. L. Zhu, Polym. Chem., 2016, 7, 689 RSC;
(g) R. M. Pearson, C. Lim, B. G. McCarthy, C. B. Musgrave and G. M. Miyake, J. Am. Chem. Soc., 2016, 138, 11399 CrossRef CAS PubMed;
(h) A. Allushi, S. Jockusch, G. Yilmaz and Y. Yagci, Macromolecules, 2016, 49, 7785 CrossRef CAS;
(i) J. Wang, L. Yuan, Z. Wang, M. A. Rahman, Y. Huang, T. Zhu, R. Wang, J. Cheng, C. Wang, F. Chu and C. Tang, Macromolecules, 2016, 49, 7709 CrossRef CAS;
(j) J. Yan, X. Pan, M. Schmitt, Z. Wang, M. R. Bockstaller and K. Matyjaszewski, ACS Macro Lett., 2016, 5, 661 CrossRef CAS;
(k) S. Jockusch and T. Yagci, Polym. Chem., 2016, 7, 6039 RSC;
(l) Z. C. Huang, Y. Gu, X. D. Liu, L. F. Zhang, Z. P. Cheng and X. L. Zhu, Macromol. Rapid Commun., 2016 DOI:10.1002/marc.201600461d.
-
(a) J. Queffelec, S. G. Gaynor and K. Matyjaszewski, Macromolecules, 2000, 33, 8629 CrossRef CAS;
(b) W. X. Wang, Z. B. Zhang, Z. P. Cheng, J. Zhu, N. C. Zhou and X. L. Zhu, Polym. Chem., 2012, 3, 2731 RSC;
(c) L. J. Bai, W. X. Wang, M. H. Wang, J. M. Sun and H. Chen, Chin. J. Polym. Sci., 2015, 33, 1260 CrossRef CAS;
(d) X. W. Jiang, J. Wu, L. F. Zhang, Z. P. Cheng and X. L. Zhu, Macromol. Rapid Commun., 2016, 37, 143 CrossRef CAS PubMed;
(e) M. Q. Ding, X. W. Jiang, J. Y. Peng, L. F. Zhang, Z. P. Cheng and X. L. Zhu, Macromol. Rapid Commun., 2015, 36, 538 CrossRef CAS PubMed;
(f) X. W. Jiang, Y. J. Luo, Z. Li, L. F. Zhang, Z. P. Cheng and X. L. Zhu, Polym. Chem., 2015, 6, 6394 RSC;
(g) Z. C. Huang, J. Chen, L. F. Zhang, Z. P. Cheng and X. L. Zhu, Polymers, 2016, 8(3), 59 CrossRef.
-
(a) G. Moineau, M. Minet, P. Dubois, P. Teyssie, T. Senninger and R. Jerome, Macromolecules, 1999, 32, 27 CrossRef CAS;
(b) C. Moineau, M. Minet, P. Teyssie and R. Jerome, Macromolecules, 1999, 32, 8277 CrossRef CAS;
(c) R. M. Johnson, P. S. Corbin, C. Ng and C. L. Fraser, Macromolecules, 2000, 33, 7404 CrossRef CAS.
-
(a) J. H. Xia, H. J. Paik and K. Matyjaszewski, Macromolecules, 1999, 32, 8310 CrossRef CAS;
(b) L. E. N. Allan, J. P. MacDonald, A. M. Reckling, C. M. Kozak and M. P. Shaver, Macromol. Rapid Commun., 2012, 33, 414 CrossRef CAS PubMed;
(c) L. J. Bai, L. F. Zhang, Z. B. Zhang, J. Zhu, N. C. Zhou, Z. P. Cheng and X. L. Zhu, J. Polym. Sci., Part A: Polym. Chem., 2011, 49, 3980 CrossRef CAS;
(d) W. W. He, L. F. Zhang, J. Miao, Z. P. Cheng and X. L. Zhu, Macromol. Rapid Commun., 2012, 33, 1067 CrossRef CAS PubMed;
(e) J. L. Pan, Z. Li, L. F. Zhang, Z. P. Cheng and X. L. Zhu, Chin. J. Polym. Sci., 2014, 32, 1010 CrossRef CAS;
(f) J. Wu, X. W. Jiang, L. F. Zhang, Z. P. Cheng and X. L. Zhu, Polymers, 2016, 8(2), 29 CrossRef;
(g) X. D. Liu, Q. Chen, G. B. Yang, L. F. Zhang, Z. Liu, Z. P. Cheng and X. L. Zhu, J. Mater. Chem. B, 2015, 3, 2786 RSC.
-
(a) L. J. Bai, W. X. Wang, H. Chen, L. F. Zhang, Z. P. Cheng and X. L. Zhu, RSC Adv., 2015, 5, 62577 RSC;
(b) K. Nishizawa, M. Ouchi and M. Sawamoto, Macromolecules, 2013, 46, 3342 CrossRef CAS;
(c) H. Schroeder, K. Matyjaszewski and M. Buback, Macromolecules, 2015, 48, 4431 CrossRef CAS;
(d) Z. G. Xue, D. He and X. L. Xie, Polym. Chem., 2015, 6, 1660 RSC.
-
(a) S. Nakanishi, M. Kawamura, H. Kai, R. H. Jin, Y. Sunada and H. Nagashima, Chem.–Eur. J., 2014, 20, 5802 CrossRef CAS PubMed;
(b) L. F. Zhang, Z. P. Cheng, Y. T. Lu and X. L. Zhu, Macromol. Rapid Commun., 2009, 30, 543 CrossRef CAS PubMed.
-
(a) Z. J. Deng, J.
N. Guo, L. H. Qiu, C. Yuan, Y. X. Zhou and F. Yan, J. Polym. Sci., Part A: Polym. Chem., 2013, 51, 664 CrossRef CAS;
(b) S. M. Zhu and D. Y. Yan, Macromol. Rapid Commun., 2000, 21, 1209 CrossRef CAS.
- M. Q. Ding, X. W. Jiang, J. Y. Peng, L. F. Zhang, Z. P. Cheng and X. L. Zhu, Green Chem., 2015, 17, 271 RSC.
-
(a) Y. Wang and K. Matyjaszewski, Macromolecules, 2010, 43, 4003 CrossRef CAS;
(b) Z. G. Xue, J. Zhou, D. He, F. Wu, D. F. Yang, Y. S. Ye, Y. G. Liao, X. P. Zhou and X. L. Xie, Dalton Trans., 2014, 43, 16528 RSC.
- B. P. Fors and C. J. Hawker, Angew. Chem., Int. Ed., 2012, 51, 8850 CrossRef CAS PubMed.
- D. Konkolewicz, K. Schroder, J. Buback, S. Bernhard and K. Matyjaszewski, ACS Macro Lett., 2012, 1, 1219 CrossRef CAS.
- X. C. Pan, N. Malhotra, J. N. Zhang and K. Matyjaszewski, Macromolecules, 2015, 48, 6948 CrossRef CAS.
-
(a) A. Takaragi, M. Minoda, T. Miyamoto, H. Q. Liu and L. N. Zhang, Cellulose, 1999, 6, 93 CrossRef CAS;
(b) K. I. Yamashita, M. Tsuboi, M. S. Asano and K. Sugiura, Synth. Commun., 2012, 42, 170 CrossRef CAS;
(c) Y. F. Tie, D. J. Wu, R. Q. Sun, P. Y. Shen and Y. L. Wang, Chin. J. Synth. Chem., 2000, 8, 301 CAS.
|
This journal is © The Royal Society of Chemistry 2017 |
Click here to see how this site uses Cookies. View our privacy policy here.