DOI:
10.1039/C7RA00664K
(Paper)
RSC Adv., 2017,
7, 16561-16564
Oxidative cross-coupling reaction by scandium catalysis for synthesis of α-alkyl α-amino acid ester derivatives†
Received
16th January 2017
, Accepted 6th March 2017
First published on 15th March 2017
Abstract
A novel oxidative cross-coupling reaction between N-arylglycine esters and alkyl boronic acid esters for synthesis of α-alkyl α-amino acid esters in the presence of scandium catalysis using silver salt as an oxidant has been developed. The mild reaction has an excellent functional group tolerance and gives the desired α-alkyl α-amino acid esters in a moderate to excellent yield.
In the past decade, the oxidative coupling reaction has become a new method to afford C–C bonds from C–H bonds due to it being atom economical and environmentally friendly.1 Meanwhile, α-amino acids play an important role in natural products and are the key structural motifs of numerous natural products.2 Therefore, the oxidative coupling reaction of glycine derivatives has gained significant attention for the synthesis of a series of α-substituted α-amino acid derivatives.3 For example, Li’s group designed the first method for an oxidative dehydrogenative coupling reaction to synthesize α-amino glycine derivatives from N-glycine derivatives by direct C–C bond formation.3a Subsequently, Huang’s group disclosed a cross-dehydrogenative coupling reaction with N-arylglycine esters to synthesize α-amino glycine derivatives under the cooperative catalysis of copper salt.3b Recently, the oxidative coupling of N-glycine derivatives has been well developed under stoichiometric amounts of chemical oxidants such as DTBP, DDQ, TBHP and 2,2,6,6-tetramethylpiperidine-1-oxoammonium tetra-fluoroborate (T+BF4−).4 Furthermore, transition metal-catalyzed synthesis of chiral α-amino acid derivatives by direct C–H oxidative cross-coupling has been reported in the past few years.5 For example, Wang’s group developed a significant method of chiral Lewis acid controlled enantioselective alkylation of α-Csp3–H bonds to synthesize chiral alkyl α-amino acid derivatives.6 Another effective pathway to synthesize chiral α-amino acids has been reported by palladium-catalyzed C–H functionalization.7 Recently, Yang’s group found a novel pathway for the synthesis of chiral α-amino acid derivatives from aryl boronic acids by Pd(II)-catalysis with direct C–H oxidation (1, Scheme 1),5g but only α-aryl α-amino acid ester derivatives were obtained. However, for the synthesis of benzyl α-amino acid derivatives by the oxidative cross-coupling reaction of glycine derivatives, very few examples have been reported to date.8 Based on this considerable progress, in this paper, we describe a novel strategy for the cross-coupling reaction between N-arylglycine esters and boronic acid esters for the synthesis of α-alkyl α-amino acid esters in the presence of Sc(III)-catalyst (2, Scheme 1).
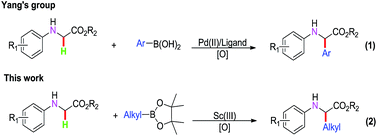 |
| Scheme 1 Reaction of glycine derivatives with metal boron reagents. | |
In an initial study, we chose N-arylglycine esters 1a as the model substrates with benzylboronic acid esters9 2a as alkylation reagents, PPh3 as the ligand, and BQ as the oxidant in the presence of 10 mol% Cu(OTf)2 in DCE at 80 °C. We were pleased to find that the desired product α-alkyl α-amino acid ester 3a was observed in 32% yield (Table 1, entry 1). Encouraged by this result, screening several oxidants (Table 1, entries 2–9) showed that Ag2CO3 was the best choice, and the yield of 3a improved to 70% (Table 1, entry 6). When the oxidant was increased to 2 equiv., the yield of 3a reached 78% (Table 1, entry 11). Subsequently, various solvents were examined and DCE was still found to be the best choice (Table 1, entries 12–16). Then, a variety of different Lewis acids were investigated, and Sc(OTf)3 proved to be the best with 85% yield for coupling product 3aa (Table 1, entries 17–21). Furthermore, the control experiment showed that only 15% yield of the desired product was obtained in the absence of Sc(OTf)3 (Table 1, entries 22–23).
Table 1 Optimization of the reaction conditionsa

|
Entry |
Catalyst |
Ligand |
Oxidant |
Solvent |
Yieldb |
Reaction conditions: 1a (0.3 mmol), 2a (1.3 equiv.), catalyst (10 mol%), ligand (10 mol%) and oxidant (1.1 equiv.) were stirred in solvent (1 mL) at 80 °C under Ar for 20 h. Yield of the isolated product. Oxidant (1.5 equiv.). Oxidant (2.0 equiv.). |
1 |
Cu(OTf)2 |
PPh3 |
BQ |
DCE |
32% |
2 |
Cu(OTf)2 |
PPh3 |
DDQ |
DCE |
ND |
3 |
Cu(OTf)2 |
PPh3 |
TBHP |
DCE |
Trace |
4 |
Cu(OTf)2 |
PPh3 |
PhI(OAc)2 |
DCE |
Trace |
5 |
Cu(OTf)2 |
PPh3 |
DCP |
DCE |
43% |
6 |
Cu(OTf)2 |
PPh3 |
Ag2CO3 |
DCE |
70% |
7 |
Cu(OTf)2 |
PPh3 |
AgOAc |
DCE |
68% |
8 |
Cu(OTf)2 |
PPh3 |
Ag2O |
DCE |
53% |
9 |
Cu(OTf)2 |
PPh3 |
AgNO3 |
DCE |
ND |
10c |
Cu(OTf)2 |
PPh3 |
Ag2CO3 |
DCE |
72% |
11d |
Cu(OTf)2 |
PPh3 |
Ag2CO3 |
DCE |
78% |
12d |
Cu(OTf)2 |
PPh3 |
Ag2CO3 |
CH3CN |
11% |
13d |
Cu(OTf)2 |
PPh3 |
Ag2CO3 |
NMP |
ND |
14d |
Cu(OTf)2 |
PPh3 |
Ag2CO3 |
TOL |
13% |
15d |
Cu(OTf)2 |
PPh3 |
Ag2CO3 |
DMF |
15% |
16d |
Cu(OTf)2 |
PPh3 |
Ag2CO3 |
Dioxane |
23% |
17d |
Zn(OTf)2 |
PPh3 |
Ag2CO3 |
DCE |
81% |
18d |
Ni(OTf)2 |
PPh3 |
Ag2CO3 |
DCE |
33% |
19d |
Al(OTf)2 |
PPh3 |
Ag2CO3 |
DCE |
79% |
20d |
Fe(OTf)2 |
PPh3 |
Ag2CO3 |
DCE |
56% |
21d |
Sc(OTf)3 |
PPh3 |
Ag2CO3 |
DCE |
85% |
22d |
|
|
Ag2CO3 |
DCE |
10% |
23 |
Sc(OTf)3 |
|
|
DCE |
15% |
Under the optimized reaction conditions (Table 1, entry 21), the reaction scope was examined as shown in Table 2. We first surveyed various N-para-bromophenyl protected glycine esters. It was found that various alkyl esters including methyl 1b and tert-butyl 1c were well tolerated in the oxidative C–H functionalization with N-arylglycine esters 1a, affording α-alkyl α-amino acid esters 3a–3c in 78–85% yields (Table 2, entries 3a–3c). Meanwhile, different substituents on the aniline fragment, which bear electron-withdrawing or electron-donating groups, afforded the corresponding desired products in moderate to excellent yields (Table 2, entries 3d–3g). However, the electronic effect in this transformation was very notable, for example, when the aniline fragment changed to N-para-methoxyphenyl, the corresponding product was obtained only in 22% yield; no substituent on the aniline fragment afforded the desired product in moderate yield (Table 2, entries 3g–3h). In order to further expand the substrate scope, we selected the N-para-bromophenyl glycine ethyl ester as the substrate, and prepared a few substrates containing substituents with electron-donating or electron-withdrawing effects on other positions of the benzyl group from the available starting materials. The corresponding α-amino acid ester products were obtained in good to excellent yields (Table 2, entries 3i–3p). Furthermore, the naphthylboronic acid ester could also undergo transformation and afford the product in 68% yield (Table 2, 3l).
Table 2 Scope of synthesis of various α-amino acid estersab
Reaction conditions: 1a (0.3 mmol), 2a (1.3 equiv.), Sc(OTf)3 (10 mol%), PPh3 (10 mol%) and Ag2CO3 (2.0 equiv.) were stirred in DCE (2.5 mL) at 80 °C under Ar for 20 h. Yield of the isolated products. |
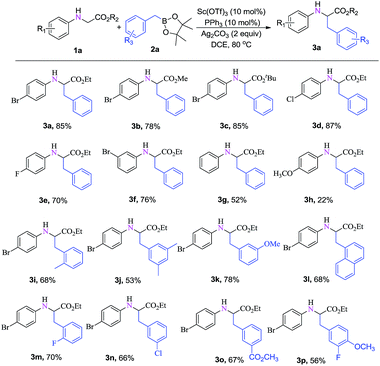 |
Moreover, to extend the scope of this catalytic system, we carried out direct functionalization of the α-peptido C–H bonds under the optimized reaction conditions. We were pleased to find that the corresponding α-alkylation product 4aa was obtained in good yield (Scheme 2). We believe that the yield of the product will increase accordingly, after appropriate optimization of the catalytic system. This work is currently under way in our laboratory.
 |
| Scheme 2 Extending the scope of the catalytic system. | |
To investigate the mechanism of this transformation, experiments were carried out. The desired product was not observed when 1.0 equivalent of the radical-trapping reagent 2,2,6,6-tetramethyl-1-piperidinyloxy (TEMPO) was used under standardized reaction conditions. At the same time, we found that when 2,6-di-tert-butyl-4-methylphenol (BHT), a radical scavenger, was introduced into the reaction system, the yield of product 3a decreased dramatically from 85% to 8% (Scheme 3). To our delight, BHT trapped the key intermediate, which was detected by GC-MS (see ESI†). This result suggests that the reaction may proceed via a radical mechanism (Scheme 4). First, benzylboronic acid ester 2a forms the intermediate benzyl radical 5 under the oxidation of Ag2CO3; at the same time, radical 6 is generated by abstracting an α hydrogen atom from N-arylglycine ester 1a under oxidant. Then the product 3aa is obtained through the radical addition reaction (Scheme 4, path A). Then, single-electron transfer (SET) occurs from 6 to cation 7, which can tautomerize to the iminium ion. At the same time, Sc(OTf)3 with the ligand PPh3 reacts as a Lewis acid with 7 to form the active species intermediate 1A. Then intermediate 1B is produced though Petasis-type addition of benzylic boronic acid 2a to the iminium intermediate.6,10 Finally, product 3aa is obtained upon dissociation in the presence of HOTf, and the active scandium catalyst is regenerated and enters the next catalytic cycle synchronously (Scheme 4, path B).
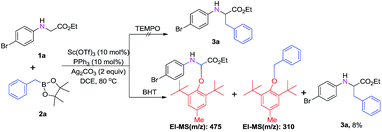 |
| Scheme 3 Radical-trapping experiment. | |
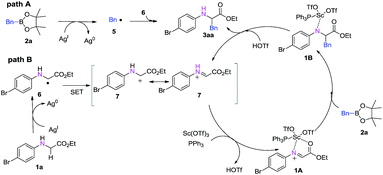 |
| Scheme 4 Proposed mechanism. | |
In conclusion, we have developed a mild and economical Sc(OTf)3-catalyzed oxidative cross-coupling reaction for the synthesis of a series of α-alkyl α-amino acid ester derivatives. Further applications of this approach to other substrates and enantioselective reactions are being investigated in our laboratory.
Acknowledgements
This work was financially supported by the National Natural Science Foundation of China (No. 21462035), the Fundamental Research Funds for the Central Universities (31920160050), the Scientific Research Foundation of Northwest University for Nationalities (xbmuyjrc201603), and the Gansu Provincial Council for Science and Technology (144GKCA031).
Notes and references
-
(a) S. H. Cho, J. Y. Kim, J. Kwak and S. Chang, Chem. Soc. Rev., 2011, 40, 5068–5083 RSC;
(b) S. A. Girard, T. Knauber and C.-J. Li, Angew. Chem., Int. Ed., 2014, 53, 74–100 CrossRef CAS PubMed;
(c) C.-J. Li, Acc. Chem. Res., 2009, 42, 335–344 CrossRef CAS PubMed;
(d) C.-J. Li and Z. Li, in Pure and Applied Chemistry, vol. 78, 2006, p. 935 Search PubMed;
(e) C. J. Scheuermann, Chem.–Asian J., 2010, 5, 436–451 CrossRef CAS PubMed;
(f) C. S. Yeung and V. M. Dong, Chem. Rev., 2011, 111, 1215–1292 CrossRef CAS PubMed.
-
(a) R. M. Williams and J. A. Hendrix, Chem. Rev., 1992, 92, 889–917 CrossRef CAS;
(b) S. Hunt, in Chemistry and Biochemistry of the Amino Acids, ed. G. C. Barrett, Chapman and Hall, London, 1985, p. 55 Search PubMed;
(c) R. M. Williams and C. M. Burnett, New Tricks in Amino Acid Synthesis: Applications to Complex Natural Products, ACS, Washington, DC, 2009, p. 420 Search PubMed;
(d) Amino Acids, Peptides and Proteins in Organic Chemistry, ed. B. H. Andrew, Wiley-VCH, Weinheim, Germany, 2011 Search PubMed.
-
(a) L. Zhao and C.-J. Li, Angew. Chem., Int. Ed., 2008, 47, 7075–7078 CrossRef CAS PubMed;
(b) J. Xie and Z.-Z. Huang, Angew. Chem., Int. Ed., 2010, 49, 10181–10185 CrossRef CAS PubMed;
(c) J. S. Bandar and T. H. Lambert, J. Am. Chem. Soc., 2013, 135, 11799–11802 CrossRef CAS PubMed;
(d) J. T. Binder, C. J. Cordier and G. C. Fu, J. Am. Chem. Soc., 2012, 134, 17003–17006 CrossRef CAS PubMed;
(e) C. Huo, C. Wang, M. Wu, X. Jia, H. Xie and Y. Yuan, Adv. Synth. Catal., 2014, 356, 411–415 CrossRef CAS;
(f) C. Huo, M. Wu, F. Chen, X. Jia, Y. Yuan and H. Xie, Chem. Commun., 2015, 51, 4708–4711 RSC;
(g) C. Huo, H. Xie, F. Chen, J. Tang and Y. Wang, Adv. Synth. Catal., 2016, 358, 724–730 CrossRef CAS;
(h) S. Kotha, Acc. Chem. Res., 2003, 36, 342–351 CrossRef CAS PubMed;
(i) C. Nájera and J. M. Sansano, Chem. Rev., 2007, 107, 4584–4671 CrossRef PubMed;
(j) S. Saito, T. Tsubogo and S. Kobayashi, J. Am. Chem. Soc., 2007, 129, 5364–5365 CrossRef CAS PubMed;
(k) M. Salman, Z.-Q. Zhu and Z.-Z. Huang, Org. Lett., 2016, 18, 1526–1529 CrossRef CAS PubMed;
(l) B. M. Trost and F. Miege, J. Am. Chem. Soc., 2014, 136, 3016–3019 CrossRef CAS PubMed;
(m) M. Wan, H. Lou and L. Liu, Chem. Commun., 2015, 51, 13953–13956 RSC;
(n) E. W. Werner, T.-S. Mei, A. J. Burckle and M. S. Sigman, Science, 2012, 338, 1455–1458 CrossRef CAS PubMed;
(o) Z.-Y. Xue, Q.-H. Li, H.-Y. Tao and C.-J. Wang, J. Am. Chem. Soc., 2011, 133, 11757–11765 CrossRef CAS PubMed;
(p) Z.-Q. Zhu, P. Bai and Z.-Z. Huang, Org. Lett., 2014, 16, 4881–4883 CrossRef CAS PubMed;
(q) C. Huo, Y. Yuan, F. Chen and Y. Wang, Adv. Synth. Catal., 2015, 357, 3648–3654 CrossRef CAS;
(r) C. Huo, H. Xie, C. Yang, J. Dong and Y. Wang, Tetrahedron Lett., 2016, 57, 2179–2182 CrossRef CAS;
(s) L. Li, Q. Wang, P. Liu, H. Meng, X.-L. Kan, Q. Liu and Y.-L. Zhao, Org. Biomol. Chem., 2016, 14, 165–171 RSC;
(t) G. Liu, J. Qian, J. Hua, F. Cai, X. Li and L. Liu, Org. Biomol. Chem., 2016, 14, 1147–1152 RSC;
(u) X.-X. Liu, Z.-Y. Wu, Y.-Q. He, X.-Q. Zhou, T. Hu, C.-W. Ma and G.-S. Huang, Adv. Synth. Catal., 2016, 358, 2385–2391 CrossRef CAS;
(v) X. Yang, L. Li, Y. Li and Y. Zhang, J. Org. Chem., 2016, 81, 12433–12442 CrossRef CAS PubMed.
-
(a) P. Liu, Y. Li, H. Wang, Z. Wang and X. Hu, Tetrahedron Lett., 2012, 53, 6654–6656 CrossRef CAS;
(b) P. Liu, Z. Wang, J. Lin and X. Hu, Eur. J. Org. Chem., 2012, 2012, 1583–1589 CrossRef CAS;
(c) H. Richter and O. García Mancheño, Org. Lett., 2011, 13, 6066–6069 CrossRef CAS PubMed;
(d) R. Rohlmann, T. Stopka, H. Richter and O. García Mancheño, J. Org. Chem., 2013, 78, 6050–6064 CrossRef CAS PubMed;
(e) Z.-Q. Wang, M. Hu, X.-C. Huang, L.-B. Gong, Y.-X. Xie and J.-H. Li, J. Org. Chem., 2012, 77, 8705–8711 CrossRef CAS PubMed;
(f) W.-T. Wei, R.-J. Song and J.-H. Li, Adv. Synth. Catal., 2014, 356, 1703–1707 CrossRef CAS;
(g) L. Zhao, O. Baslé and C.-J. Li, Proc. Natl. Acad. Sci. U. S. A., 2009, 106, 4106–4111 CrossRef CAS PubMed.
-
(a) M.-X. Cheng, R.-S. Ma, Q. Yang and S.-D. Yang, Org. Lett., 2016, 18, 3262–3265 CrossRef CAS PubMed;
(b) Y.-Y. Huang, C. Cai, X. Yang, Z.-C. Lv and U. Schneider, ACS Catal., 2016, 6, 5747–5763 CrossRef CAS;
(c) Z. Meng, S. Sun, H. Yuan, H. Lou and L. Liu, Angew. Chem., Int. Ed., 2014, 53, 543–547 CrossRef CAS PubMed;
(d) S. Stockerl and O. G. Mancheno, Org. Chem. Front., 2016, 3, 277–280 RSC;
(e) S. Sun, C. Li, P. E. Floreancig, H. Lou and L. Liu, Org. Lett., 2015, 17, 1684–1687 CrossRef CAS PubMed;
(f) S. Sun, Y. Mao, H. Lou and L. Liu, Chem. Commun., 2015, 51, 10691–10694 RSC;
(g) X.-H. Wei, G.-W. Wang and S.-D. Yang, Chem. Commun., 2015, 51, 832–835 RSC;
(h) Z. Xie, X. Zan, S. Sun, X. Pan and L. Liu, Org. Lett., 2016, 18, 3944–3947 CrossRef CAS PubMed;
(i) J. Chen, X. Lu, W. Lou, Y. Ye, H. Jiang and W. Zeng, J. Org. Chem., 2012, 77, 8541–8548 CrossRef CAS PubMed;
(j) A. Córdova, Acc. Chem. Res., 2004, 37, 102–112 CrossRef PubMed;
(k) N. Gommermann, C. Koradin, K. Polborn and P. Knochel, Angew. Chem., Int. Ed., 2003, 42, 5763–5766 CrossRef CAS PubMed;
(l) M. Hatano, T. Horibe and K. Ishihara, J. Am. Chem. Soc., 2009, 132, 56–57 CrossRef PubMed;
(m) K. R. Knudsen, T. Risgaard, N. Nishiwaki, K. V. Gothelf and K. A. Jørgensen, J. Am. Chem. Soc., 2001, 123, 5843–5844 CrossRef CAS;
(n) S. Kobayashi, T. Hamada and K. Manabe, J. Am. Chem. Soc., 2002, 124, 5640–5641 CrossRef CAS PubMed;
(o) S. Kobayashi, Y. Mori, J. S. Fossey and M. M. Salter, Chem. Rev., 2011, 111, 2626–2704 CrossRef CAS PubMed;
(p) C. Koradin, K. Polborn and P. Knochel, Angew. Chem., Int. Ed., 2002, 41, 2535–2538 CrossRef CAS;
(q) T. Beisel, A. M. Diehl and G. Manolikakes, Org. Lett., 2016, 18, 4116–4119 CrossRef CAS PubMed;
(r) A. E. Taggi, A. M. Hafez and T. Lectka, Acc. Chem. Res., 2002, 36, 10–19 CrossRef PubMed;
(s) B. M. Trost and L. R. Terrell, J. Am. Chem. Soc., 2002, 125, 338–339 CrossRef PubMed;
(t) C. Wei and C.-J. Li, J. Am. Chem. Soc., 2002, 124, 5638–5639 CrossRef CAS PubMed;
(u) C. Wei, J. T. Mague and C.-J. Li, Proc. Natl. Acad. Sci. U. S. A., 2004, 101, 5749–5754 CrossRef CAS PubMed;
(v) C.-Y. Zhou, S.-F. Zhu, L.-X. Wang and Q.-L. Zhou, J. Am. Chem. Soc., 2010, 132, 10955–10957 CrossRef CAS PubMed;
(w) Z. Xie, X. Liu and L. Liu, Org. Lett., 2016, 18, 2982–2985 CrossRef CAS PubMed.
- G. Zhang, Y. Zhang and R. Wang, Angew. Chem., Int. Ed., 2011, 50, 10429–10432 CrossRef CAS PubMed.
-
(a) K. Chen, F. Hu, S.-Q. Zhang and B.-F. Shi, Chem. Sci., 2013, 4, 3906–3911 RSC;
(b) J. He, S. Li, Y. Deng, H. Fu, B. N. Laforteza, J. E. Spangler, A. Homs and J.-Q. Yu, Science, 2014, 343, 1216–1220 CrossRef CAS PubMed;
(c) Q. Zhang, K. Chen, W. Rao, Y. Zhang, F.-J. Chen and B.-F. Shi, Angew. Chem., Int. Ed., 2013, 52, 13588–13592 CrossRef CAS PubMed;
(d) S.-Y. Zhang, Q. Li, G. He, W. A. Nack and G. Chen, J. Am. Chem. Soc., 2013, 135, 12135–12141 CrossRef CAS PubMed.
- Z. Xie, J. Jia, X. Liu and L. Liu, Adv. Synth. Catal., 2016, 358, 919–925 CrossRef CAS.
-
(a) A. Giroux, Tetrahedron Lett., 2003, 44, 233–235 CrossRef CAS;
(b) T. Liu, X. Shao, Y. Wu and Q. Shen, Angew. Chem., Int. Ed., 2012, 51, 540–543 CrossRef CAS PubMed.
-
(a) T. Thaima and S. G. Pyne, Org. Lett., 2015, 17, 778–781 CrossRef CAS PubMed;
(b) R. Frauenlob, C. Carcía, G. A. Bradshaw, H. M. Burke and E. Bergin, J. Org. Chem., 2012, 77, 4445–4449 CrossRef CAS PubMed;
(c) F. Yang, K.-G. Ji, S. Ali and Y.-M. Liang, J. Org. Chem., 2011, 76, 8329–8335 CrossRef CAS PubMed;
(d) S. Kobayashi and S. Nagayama, J. Org. Chem., 1997, 62, 232–233 CrossRef CAS PubMed;
(e) B. Kang, A. W. Miller, S. Goyal and S. T. Nguyen, Chem. Commun., 2009, 3928–3930 RSC;
(f) S. Lee, W. L. Lee and J. Yun, Adv. Synth. Catal., 2015, 357, 2219–2222 CrossRef CAS.
Footnote |
† Electronic supplementary information (ESI) available. See DOI: 10.1039/c7ra00664k |
|
This journal is © The Royal Society of Chemistry 2017 |
Click here to see how this site uses Cookies. View our privacy policy here.