DOI:
10.1039/C7RA00794A
(Paper)
RSC Adv., 2017,
7, 17732-17740
Efficient one-pot synthesis of 3,4-dihydropyrimidin-2(1H)-ones catalyzed by a new heterogeneous catalyst based on Co-functionalized Na+-montmorillonite†
Received
18th January 2017
, Accepted 10th March 2017
First published on 22nd March 2017
Abstract
In the present study, we report a simple, facile and efficient solvent-free protocol for the synthesis of 3,4-dihydropyrimidin-2(1H)-ones by the three component condensation of aldehyde, ethyl acetoacetate and urea through using Co@imine-Na+-MMT as a new, environmentally friendly and reusable heterogeneous catalyst. The structure of the synthesized catalyst was characterized by various techniques, including IR, SEM, TGA, EDX, AAs, DR-UV-vis and XRD analyses. Green reaction conditions, high quantitative yields and the easy isolation of products, easy separation and recovery of the catalyst and short reaction times are some of the advantages of our procedure.
1. Introduction
Montmorillonite (MMT) is one of the phyllosilicate minerals that has received considerable attention due to its unique features such as layered structure, large surface area, thermal and mechanical stability and high ion-exchange capacity. To date, montmorillonite has been used in various fields such as pharmaceuticals, decolorizing agents and supported catalysts.1–7 The building blocks of this kind of clay consist of a central octahedral aluminum sheet [Al(OH6)3−] sandwiched by two tetrahedral sheets of silica (Si2O52−).8 As a result of these structural features, a permanent negative charge develops in the MMT layers, which is normally balanced by alkali or earth alkaline cations such as Na+, K+, and Ca2+.9 Normally, montmorillonite layers have a thickness of approximately 1 nm and lateral dimensions of about 30 nm.10 Generally, broken bonds on the edge of the layered silicate lead to the formation of hydroxyl groups, which can be functionalized to admit catalyst moieties.11–13 For example, Shirini et al. reported the synthesis of 4,4-(arylmethylene)-bis-(3-methyl-1-phenyl-1H-pyrazol-5-ol) derivatives in the presence of Na+-MMT-[pmim]HSO4.14 Also, Sarvi et al. have presented syntheses of different xanthenes and coumarins in the presence of AHS@MMT.15
Dihydropyrimidinones (DHPMs) are an outstanding class of heterocyclic compounds. Many properties, such as antibacterial, antioxidant, anti-HIV and anticancer activities have been reported for these compounds.16,17 Hitherto, various derivatives of these structural motifs have exhibited calcium channel modulatory effect in treatment of cardiovascular diseases.18 3,4-Dihydropyrimidin-2(1H)-ones can be prepared by one-pot condensation of an aldehyde, β-ketoester and urea under strongly acidic conditions.19 The multicomponent Biginelli reaction can proceed in the presence of Ln(OTf)3,20 NiCl2·5H2O,21 Fe(OTs)3·6H2O,22 Bi(NO3)3,23 Fe(OTs)3·6H2O,24 Fe3O4 NPs,25 Mn(OAc)3·2H2O,26 p-dodecylbenzenesulfonic acid,27 bakers' yeast,28 (NH4)2CO3,29 silica sulfuric acid,30 sulfated tungstate,31 Cu(OTf)2,32 CD-SO3H,33 FeCl3·6H2O34 IRMOF-3,35 chiral phosphoric acid,36 bentonite/PS-SO3H,37 Ce(LS)3,38 NH4OAc39 as a catalyst. Although these strategies provide an improvement in the preparation of these heterocyclic molecules, some of these methods suffer from such disadvantages as harsh reaction conditions, the need for excess amounts of the catalyst, the use of toxic organic solvents, low yield and prolonged reaction time. Therefore, investigations for development of more efficient, simpler, and milder procedures to overcome these problems are still required (Scheme 1).
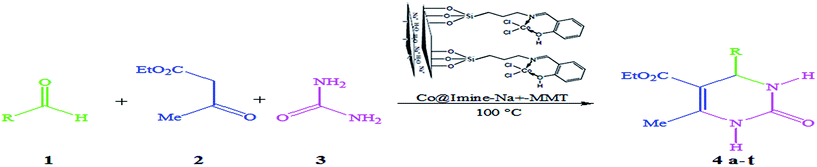 |
| Scheme 1 One-pot three-component reaction of different aldehydes, ethyl acetoacetate and urea catalyzed by Co@imine-Na+-MMT nanocatalyst. | |
2. Experimental
2.1. Material and method
Chemical reagents in high purity were purchased from Fluka or Merck and Southern Clay Products companies. 3-Aminopropyltriethoxysilane (APTES) with 99% purity, 2-hydroxybenzaldehyde with 99.9% purity and dichloromethane with 99.8% purity were bought from Sigma-Aldrich, USA. Toluene was purchased from Shanghai Experiment Reagent Co., Ltd (Shanghai, China).
2.1.1. Functionalization of Na+-MMT with 3-aminopropyltriethoxysilane (APTES@Na+-MMT). Na+-MMT (2 g) was dispersed in dry toluene (50 mL) by sonication for 40 min, and then 3-aminopropyltriethoxysilane (APTES, 4 mL) was introduced to the above-mentioned solution. The mixture was refluxed for 24 h under nitrogen atmosphere. After 24 h, the functionalized Na+-MMT (APTES@Na+-MMT) was filtered and washed thoroughly, using the mixture of H2O/ethanol and APTES@Na+-MMT dried under vacuum oven.
2.1.2. Preparation of imine@Na+-MMT. To prepare imine@Na+-MMT, APTES@Na+-MMT (2 g) was dispersed in 20 mL of CH2Cl2 for 30 min. Subsequently, salicylaldehyde (0.245 g) was added to the reaction mixture and the mixture was refluxed for 8 h. Finally, imine@Na+-MMT was separated by filtration, washed with water (20 mL), and then dried under vacuum oven. Amount of N in the imine@Na+-MMT was 2.3 wt%.
2.1.3. Preparation of Co@imine-Na+-MMT. Two g of imine@Na+-MMT was suspended in CH2Cl2 (20 mL). Afterwards, CoCl2 (0.25 mmol) was added to 10 mL of CH2Cl2, and the reaction mixture was stirred for 24 h under a nitrogen atmosphere. After 24 h, Co@imine-Na+-MMT was separated by filtration, and the product was washed with water to remove unreacted metal precursors. Amount of N in the Co@imine-Na+-MMT was 1.7 wt%. All stages of the Co@imine-Na+-MMT synthesis is shown in Scheme 2.
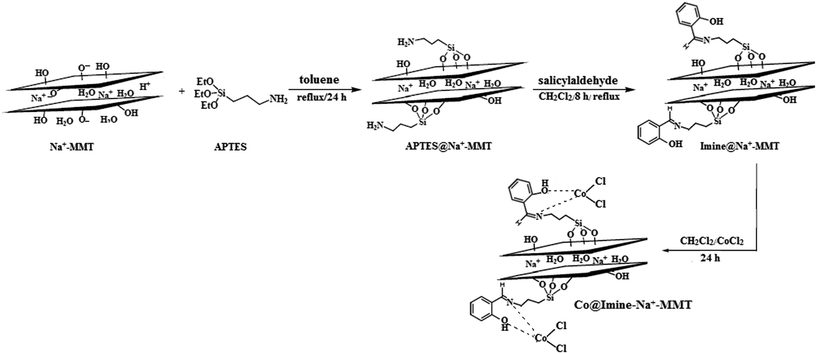 |
| Scheme 2 All stages of the Co@imine-Na+-MMT synthesis. | |
2.2. Instrumental measurements
The purity determination of the product and reaction monitoring were carried out by TLC on silica gel 60 F254 (0.25 mm thick). NMR spectra were measured by a Bruker DRX-400 AVANCE spectrometer (1H NMR: 400 MHz; 13C NMR: 100 MHz). FTIR spectra were obtained in KBr pallets on a PerkinElmer PXI spectrometer. The low- and broad-angle X-ray diffraction (XRD) measurements were recorded in the 2θ range of 2–80° at room temperature on a Philips X-pert diffractometer (Holland), using Ni-filtered Co-Kα radiation (λ = 1.54 Å). Scanning election microphotograph (SEM-EDX) was carried out on a SEM-Philips XL30. Thermogravimetric analyses (TGA) were performed at the temperature ranging from room temperature to 700 °C in a nitrogen flow by using a Linseis SATPT instrument. Diffuse reflection spectroscopy was recorded on a Sinco S4100, Korea. Elemental analyses were done, using a Carlo-Erba EA1110CNNO-S analyzer and agreed (within 0.30) with the calculated values.
3. Results and discussion
3.1. Synthesis and characterization of catalyst
3.1.1. IR analysis of Co@imine-Na+-MMT. The corresponding FT-IR spectra of the pure Na+-MMT, APTES@Na+-MMT, imine@Na+-MMT and Co@imine-Na+-MMT samples in the region of 400–4000 cm−1 are presented in Fig. 1. In the case of pure Na+-MMT, the absorption peak at 3632 cm−1 is related to the free hydroxyl units bonded to the aluminum or magnesium; however the peaks appearing in the 3441 and 1638 cm−1 region can be attributed to the OH stretching vibration of the adsorbed water. The bands at 1044 and 918 cm−1 are collectively attributed to Si–O stretching vibrations. In the case of APTES@Na+-MMT, the observed peaks at 1636 and 3437 cm−1 are assigned to the –NH2 bending and stretching vibrations, respectively. In addition, the absorption peaks at 2938 cm−1 are assigned to the C–H stretching vibrations of propyl groups of APTES. The bands at 1400–1500 cm−1 in the imine@Na+-MMT are related to the aromatic C
C stretching while the absorption peak in the 1651 cm−1 region can be assigned to the C
N double bond. The shift of absorption band related to the C
N band from 1651 cm−1 in imine@Na+-MMT to 1648 cm−1 in Co@imine-Na+-MM confirms the coordination of Schiff base ligand. Furthermore, the spectra of the Co@imine-Na+-MM and recovered Co@imine-Na+-MMT were compared. The spectra are virtually identical which confirm the fine reusability of the catalyst.
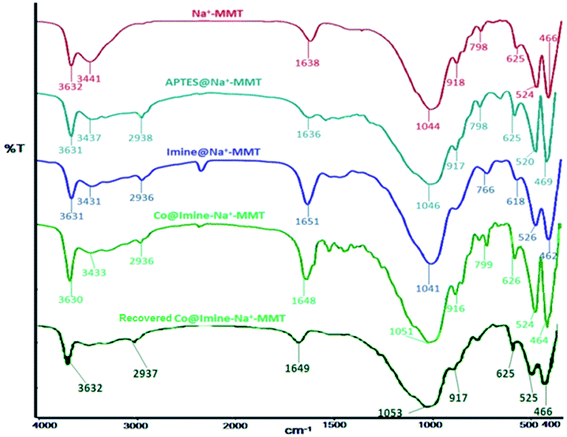 |
| Fig. 1 FTIR spectra of Na+-MMT, APTES@Na+-MMT, imine@Na+-MMT, Co@imine-Na+-MMT and recovered Co@imine-Na+-MMT. | |
3.1.2. Thermal studies of Co@imine-Na+-MM. The TG curve of Na+-MMT displays a weight loss of approximately 8% up to a temperature of 150 °C which is concerned with the loss of the physically adsorbed water. Additionally, there is a slight weight loss between 150 and 600 °C, probably corresponding to the breaking of the bonded H2O within the gallery. TGA of Co@imine-Na+-MMT illustrates approximately 9% weight loss up to 330 °C due to the adsorbed water in the clay. Furthermore, the second weight loss (15%) appearing at 350 °C is associated with the decomposition of the anchored alkyl chain of imines (Fig. 2).
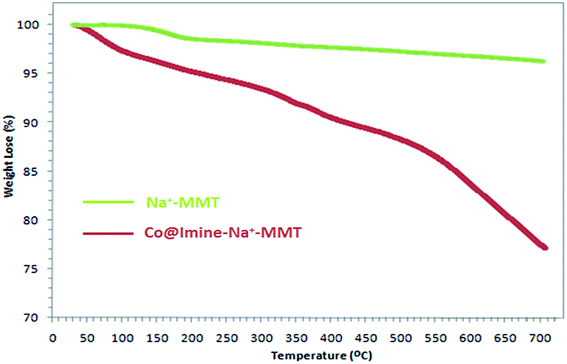 |
| Fig. 2 TGA curves of Na+-MMT and Co@imine-Na+-MMT. | |
3.1.3. SEM analysis of Co@imine-Na+-MM. Scanning electron microscopy (SEM) was investigated to determine the morphology and size details, as shown in Fig. 3a and b. The SEM images for the catalysts clearly showed variations in the surface of these nanoparticles. In the case of Co@imine-Na+-MMT with disappearance of the lumpy shape of Na+-MMT, some layers were formed as a uniform structure, which could be attributed to the anchoring of the organic and metal groups. According to Fig. 3b, Co@imine-Na+-MMT has flaky shapes with nano dimension of 40–50 nm in size.
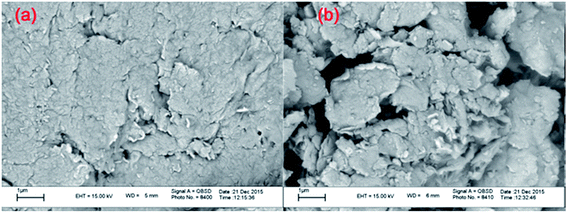 |
| Fig. 3 SEM images of Na+-MMT (a) and Co@imine-Na+-MMT (b). | |
3.1.4. EDX analysis of Co@imine-Na+-MMT. The chemical identity of the Co@imine-Na+-MMT nanoparticles was corroborated by EDX analysis (Fig. 4). The EDX analysis of the nanocatalysts indicated expected elements such as Fe, Mg, Al, C, O and Co of Co@imine-Na+-MMT. In addition, the Co content of the nanoparticles was determined to be about 0.5 wt% by the atomic absorption spectroscopy (AAS) analysis.
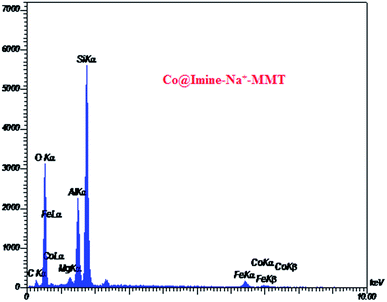 |
| Fig. 4 The energy-dispersive X-ray spectroscopy (EDX) of the Co@imine-Na+-MMT. | |
3.1.5. XRD analysis of Co@imine-Na+-MMT. Fig. 5a displays the low-angle XRD (X-ray diffraction) patterns of the Na+-MMT used, and Co@imine-Na+-MMT to illustrate the changes in the distance between the montmorillonite layers (d001). The interlayer distance in the Na+-MMT (11.42 Å) increases in the course of chemical modifications up to 12.45 Å for Co@imine-Na+-MMT. This change can point that Co species are intercalated into the interlayer of montmorillonite with the assistance of the organic groups. Additionally, comparison of the broad-angle X-ray diffraction patterns of Na+-MMT and Co@imine-Na+-MMT demonstrates that peak patterns of Na+-MMT were preserved (Fig. 5b). This clearly shows that the crystalline structure and morphology of the montmorillonite nanoparticles remained intact upon organic functionalization as well as metal complex formation in Na+-MMT. It should be noted that no peak dedicated to Co species appeared in the patterns of Co@imine-Na+-MMT compared those of Na+-MMT, probably due to the tiny size of Co species.
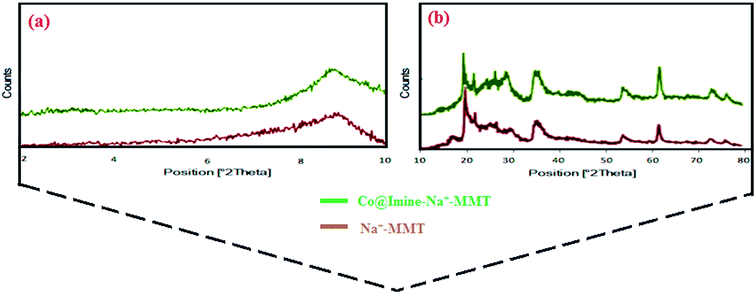 |
| Fig. 5 XRD patterns of the Na+-MMT and Co@imine-Na+-MMT. | |
3.1.6. DR-UV-vis analysis of Co@imine-Na+-MMT. Diffuse Reflectance Spectra (DRS) of imine@Na+-MMT and Co@imine-Na+-MMT was obtained (Fig. 6), and a comparison between them revealed that the foundation remains unchanged during the metal anchoring process.
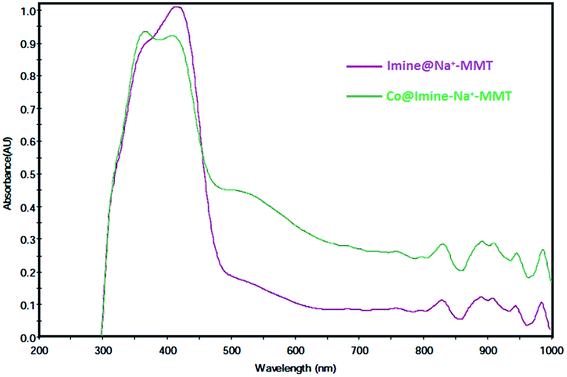 |
| Fig. 6 Diffuse reflectance spectroscopy (DRS) of Na+-MMT and Co@imine-Na+-MMT. | |
3.2. Synthesis of 3,4-dihydropyrimidin-2(1H)-ones in the presence of Co@imine-Na+-MMT
A mixture of aldehyde (1 mmol), ethyl acetoacetate (1 mmol), urea (1.2 mmol), and Co@imine-Na+-MMT (8 mg) was stirred at 100 °C under solvent-free conditions. After the completion of the reaction (monitored by TLC), EtOH (10 mL) was added to the mixture, and the catalyst was filtered off. The pure product was achieved via recrystallization from ethanol. The structures of the products were characterized by the comparison of their melting points, and NMR spectroscopic data with those of the related literature.
In continuation of our efforts to perform organic transformations with the aid of green synthetic methodologies,40–43 we decided to survey the synthesis of dihydropyrimidin-2(1H)-one compounds with diverse substituents from the reaction of ethyl acetoacetate, aldehyde and urea under solvent-free conditions by using Co@imine-Na+-MMT as a novel, eco-friendly, reusable and promising nanocatalysts.
In order to optimize the reaction conditions for the synthesis of 3,4-dihydro-5-etoxycarbonyl-4-(4-nitrophenyl)-6-methylpyrimidine-2(1H)-one (4d), a systematic study, which considered different variables affecting the reaction yield, was conducted in the reaction of ethyl acetoacetate, 4-nitrobenzaldehyde, and urea (molar ratio 1
:
1
:
1.2) as the model reaction. The results are indicated in Table 1. The primary optimization results showed that the ambient of the catalyst was not suitable to formation of the desired product 4d under solvent-free conditions even at 100 °C (entry 1). In the next phase of study, systematic screening of the reaction conditions was evaluated in the presence of different catalyst amounts (entries 2–4). After several screening experiments with different amounts of catalysts, it was proved that the reaction in the presence of 10 mg of the catalysts under solvent-free conditions resulted in the corresponding product 4d in the highest yield (entry 3). Increasing and decreasing the amount of catalysts required for the reaction did not considerably affect the duration of the reaction and the product yield (entries 2 and 4). However, the trial reaction in the presence of solvents such as H2O, EtOH, DMF, DMSO, CH2Cl2, CHCl3, and toluene acquired the desired product 4d in good to moderate yields but at long reaction times in compared to the solvent-free conditions (entries 5–11). Among the surveyed solvents, using EtOH improved the yield of the chemical reaction under study (entry 6). Moreover, to determine the role of catalysts and temperature under solvent-free conditions, the reaction was surveyed with and without heating with the same amount of catalysts (10 mg) (entries 12–14). It was observed that the use of heating leads to faster reaction and a higher yield.
Table 1 Optimization of the reaction conditions in different solventsa
Entry |
Solvent |
Amount of catalyst (mg) |
Temperature (°C) |
Reaction time (min) |
Yieldb (%) |
Reaction conditions: a mixture of 4-nitrobenzaldehyde (1 mmol), ethyl acetoacetate (1 mmol), urea (1.2 mmol) and Co@imine-Na+-MMT (10 mg). Isolated yield. |
1 |
— |
— |
100 |
60 |
— |
2 |
— |
5 |
100 |
10 |
90 |
3 |
— |
10 |
100 |
10 |
96 |
4 |
— |
15 |
100 |
10 |
94 |
5 |
H2O |
10 |
Reflux/100 °C |
30 |
49 |
6 |
EtOH |
10 |
Reflux/78 °C |
30 |
82 |
7 |
DMF |
10 |
Reflux/153 °C |
30 |
38 |
8 |
DMSO |
10 |
Reflux/189 °C |
30 |
42 |
9 |
CH2Cl2 |
10 |
Reflux/40 °C |
30 |
40 |
10 |
CHCl3 |
10 |
Reflux/61 °C |
30 |
41 |
11 |
Toluene |
10 |
Reflux/110 °C |
30 |
76 |
12 |
— |
10 |
— |
10 |
35 |
13 |
— |
10 |
80 |
10 |
85 |
14 |
— |
10 |
90 |
10 |
92 |
In order to generalize the effectiveness and the acceptability of the optimum conditions, a variety of appropriate aldehydes (2a–t) were selected for the reaction with ethyl acetoacetate (1) and urea (3); in addition, the desired products were obtained in good-to-excellent yields without problems which may be related to the use of solvents such as pollution, handling and safety. The optimized results are listed in Table 2. It was found that aromatic aldehydes containing both electron-withdrawing (entries 2–13) and electron-donating groups (entries 15–19) showed pretty good reactivity with the ethyl acetoacetate and urea under the optimized reaction conditions.
Table 2 The Co@imine-Na+-MMT catalyzed three-component Biginelli couplinga
Entry |
R |
Yieldb (%) |
Time (min) |
Mpc (°C) found |
Mp (°C) (Lit) |
Reaction conditions: aldehyde (1 mmol), ethyl acetoacetate (1 mmol), urea (1.2 mmol) and Co@imine-Na+-MMT (10 mg), 100 °C. Isolated yield. Melting points are uncorrected. |
4a |
C6H5– |
92 |
10 |
203–204 |
202–203 (ref. 44) |
4b |
2-(NO2)–C6H4– |
94 |
10 |
219–221 |
220–222 (ref. 26) |
4c |
3-(NO2)–C6H4 |
94 |
10 |
225–226 |
226–228 (ref. 44) |
4d |
4-(NO2)–C6H4– |
96 |
10 |
209–211 |
208–211 (ref. 50) |
4e |
2-(Cl)–C6H4– |
95 |
10 |
216–218 |
215–218 (ref. 24) |
4f |
3-(Cl)–C6H4– |
94 |
10 |
192–194 |
192–193 (ref. 35) |
4g |
4-(Cl)–C6H4– |
95 |
10 |
212–214 |
212–214 (ref. 21) |
4h |
2,4-(Cl)2–C6H3– |
96 |
10 |
251–253 |
251–252 (ref. 47) |
4i |
3,4-(Cl)2–C6H3– |
94 |
10 |
222–224 |
222–223 (ref. 49) |
4j |
4-(F)–C6H4– |
93 |
10 |
177–179 |
175–177 (ref. 19) |
4k |
4-(CF3)–C6H4– |
95 |
10 |
176–178 |
173–175 (ref. 45) |
4l |
3-(Br)–C6H4– |
91 |
10 |
185–188 |
185–186 (ref. 48) |
4m |
2-(HO)–C6H4– |
91 |
10 |
199–200 |
199–201 (ref. 46) |
4n |
4-(HO)–C6H4– |
92 |
10 |
230–232 |
231–233 (ref. 44) |
4o |
2-(OCH3)–C6H4– |
93 |
10 |
259–261 |
259–260 (ref. 30) |
4p |
3-(OCH3)–C6H4– |
91 |
10 |
207–209 |
207–208 (ref. 23) |
4q |
4-(OCH3)–C6H4– |
93 |
10 |
202–204 |
203–204 (ref. 44) |
4r |
3,4-(OCH3)2–C6H3– |
91 |
10 |
173–176 |
174–176 (ref. 49) |
4s |
3-(CH3O)–4-(HO)– |
91 |
10 |
232–234 |
233–235 (ref. 44) |
4t |
4-(CH3)–C6H4– |
92 |
10 |
215–217 |
216–217 (ref. 45) |
Furthermore, to illustrate the catalytic efficiency and the capability of Co@imine-Na+-MMT, Table 3 compares our results in the synthesis of 3,4-dihydro-5-etoxycarbonyl-4-(4-phenyl)-6-methylpyrimidine-2(1H)-one (4a) with those of other methodologies which have employed other earlier homogeneous and heterogeneous catalysts. It is crystal clear that a suitable methodology in terms of product yield, reaction time, and especially catalyst amount has been developed.
Table 3 Comparison the results of the synthesis of 3,4-dihydro-5-etoxycarbonyl-4-(4-phenyl)-6-methylpyrimidine-2(1H)-one using different catalysts
Entry |
Catalyst and conditions |
Reaction time (h) |
Yield (%) |
Ref. |
3 |
BF3·OEt2/CuCl/THF/reflux |
18 |
71 |
23 |
1 |
Silica sulfuric acid/EtOH/heat |
6 |
91 |
30 |
2 |
Sulfated tungstate/solvent-free/80 °C |
1 |
92 |
31 |
4 |
Cu(OTf)2/EtOH/100 °C/MW |
1 |
95 |
32 |
5 |
CD-SO3H/solvent-free/80 °C |
2 |
89 |
33 |
8 |
IRMOF-3/solvent-free/reflux |
5 |
89 |
34 |
9 |
Chiral phosphoric acid/CH2Cl2/25 °C |
4 days |
77 |
36 |
12 |
SnCl2-nano SiO2/EtOH/reflux |
40 min |
92 |
51 |
13 |
Co@imine-Na+-MMT/solvent-free/100 °C |
10 min |
92 |
This work |
In the next phase of the survey, to check the recovery and reuse cycle of the catalyst, the reaction of 4-nitrobenzaldehyde, ethyl acetoacetate and urea under the optimized reaction conditions was selected as a model reaction. After separating and washing the retained catalyst with ethanol, the above-mentioned solvent was evaporated, and the catalyst was dried and reused for the same reactions. This process was performed over five periods, and all reactions led to the desired products with negligible change in terms of the reaction time and yield, obviously showing the practical recyclability of this catalyst (Fig. 7). However, the yield of product was reduced from 96% to 94% after the 5th run.
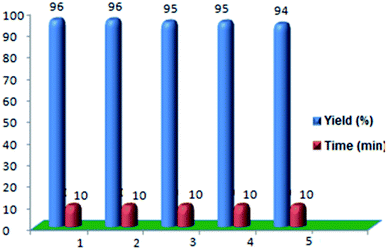 |
| Fig. 7 Reusability of the Co@imine-Na+-MMT. | |
A reasonable pathway for the reaction of ethyl acetoacetate, aldehyde, and urea or thiourea conducted in the presence of Co@imine-Na+-MMT is presented in Scheme 2. Due to the 3d empty orbital in the cobalt ion, the activated aldehyde (2), and ethyl acetoacetate (1) can be created through a coordinative bond and stabilized by cobalt. The dihydropyrimidin-2(1H)-one synthesis probably begins via initial formation of the acylimine intermediate (5) by nucleophilic addition of urea (3) to the activated aldehyde (2). The resulting adduct (5) undergoes dehydration to give the complex (6). In this stage, activated ethyl acetoacetate (1) attacks the complex (6). Furthermore, an open-chain ureide (7) is created which undergoes intramolecular cyclization to afford the final product (4) (Scheme 3).
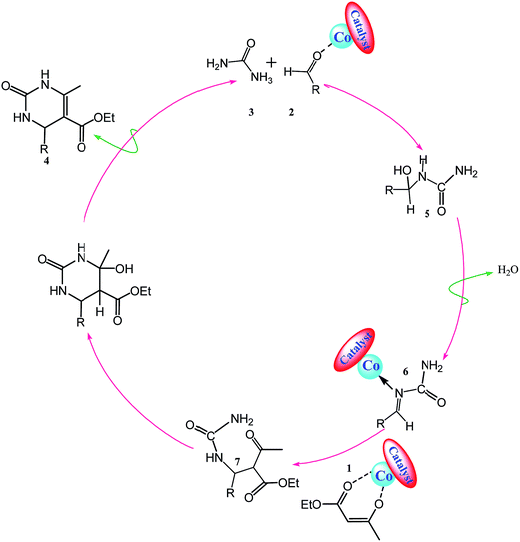 |
| Scheme 3 The proposed mechanism for the synthesis dihydropyrimidin-2(1H)-ones in the presence of Co@imine-Na+-MMT. | |
4. Conclusions
In conclusion, we have introduced a simple, facile, and efficient protocol for the synthesis of a wide range of biologically and pharmacologically 3,4-dihydropyrimidin-2(1H)-ones in the presence of Co@imine-Na+-MMT as a new, environmentally friendly and reusable heterogeneous catalyst via a one-pot Knoevenagel condensation of aldehyde, ethyl acetoacetate and urea under solvent-free conditions.
References
- K. Majdzadeh-Ardakani, A. H. Navarchian and F. Sadeghi, Carbohydr. Polym., 2010, 79, 547 CrossRef CAS.
- S. Kantevari, S. V. N. Vuppalapati and L. Nagarapu, Catal. Commun., 2007, 8, 1857 CrossRef CAS.
- J. S. Yadav, B. V. S. Reddy, B. Eeshwaraiah and M. Srinivas, Tetrahedron, 2004, 60, 1767 CrossRef CAS.
- B. S. Kumar, A. Dhakshinamoorthy and K. Pitchumani, Catal. Sci. Technol., 2014, 4, 2378 CAS.
- F. Shirini, M. Mamaghani and S. V. Atghia, Catal. Commun., 2011, 12, 1088 CrossRef CAS.
- D. S. Tong, C. H. Zhou, M. Y. Li, W. H. Yu, J. Beltramini, C. X. Lin and Z. P. Xu, Appl. Clay Sci., 2010, 48, 569 CrossRef CAS.
- D. Zhang, C. H. Zhou, C. X. Lin, D. S. Tong and W. H. Yu, Appl. Clay Sci., 2010, 50, 1 CrossRef CAS.
- Crystal Structures of clay and their X-ray identification, Monograph, ed. G. W. Brindly and G. Brown, Mineralogical Society, London, 1980, pp. 125–195 Search PubMed.
- E. P. Giannelis, R. Krishnamoorti and E. Manias, Adv. Polym. Sci., 1999, 118, 108 Search PubMed.
- M. Okamoto, S. Mallapragada and B. Narasimhan, Biodegradable Polymer/Layered Silicate Nanocomposites: a review, Handbook of Biodegradable Polymeric Materials and Their Applications, American Scientific Publishers, Valencia, California, 2005, pp. 1–45 Search PubMed.
- H. Shi, T. Lan and T. Pinnavaia, Chem. Mater., 1996, 8, 1584 CrossRef CAS.
- S. Yariv and H. Cross, in Clays and Clay Minerals, ed. M. Dekker, New York, 2002, pp. 463–566 Search PubMed.
- O. Ozdemir, B. Armagan, M. Turan and M. S. Celik, Dyes Pigm., 2004, 62, 49 CrossRef CAS.
- F. Shirini, M. Seddighi, M. Mazloumi, M. Makhsous and M. Abedini, J. Mol. Liq., 2015, 208, 291 CrossRef CAS.
- S. Sarvi-Beigbaghlou, K. Marjani, A. Habibi and S. V. Atghia, RSC Adv., 2016, 6, 20306 RSC.
- C. O. Kappe, Eur. J. Med. Chem., 2000, 35, 1043 CrossRef CAS PubMed.
- S. S. Kim, B. S. Choi, J. H. Lee, K. K. Lee, T. H. Lee, Y. H. Kim and S. Hyunik, Synlett, 2009, 599 CAS.
- K. S. Atwal, B. N. S. Wanson, S. E. Unger, D. M. Floyd, S. Mereland, A. Hedberg and B. C. J. O'Reilly, Med. Chem., 1991, 34, 806 CrossRef CAS PubMed.
- P. G. Biginelli, Gazz. Chim. Ital., 1893, 23, 360 Search PubMed.
- Y. Huang, F. Yang and C. Zhu, J. Am. Chem. Soc., 2005, 127, 16386 CrossRef CAS PubMed.
- U. B. More, Asian J. Chem., 2012, 24, 1906 CAS.
- J. T. Starcevich, T. J. Laughlin and R. S. Mohan, Tetrahedron Lett., 2013, 54, 983 CrossRef CAS.
- M. M. Khodaei, A. R. Khosropour and M. Beygzadeh, Synth. Commun., 2004, 34, 1551 CrossRef CAS.
- C. O. Kappe, D. Kumar and R. S. Varma, Synthesis, 1999, 10, 1799 CrossRef.
- M. N. Esfahani, S. J. Hoseini and F. Mohammadi, Chin. J. Catal., 2011, 32, 1484 CrossRef.
- K. A. Kumar, M. Kasthuraiah, C. S. Reddy and C. D. Reddy, Tetrahedron Lett., 2001, 42, 7873 CrossRef.
- M. A. Bigdeli, G. Gholami and E. Sheikhhosseini, Chin. Chem. Lett., 2011, 22, 903 CrossRef CAS.
- A. Kumar and R. A. Maurya, Tetrahedron Lett., 2007, 48, 4569 CrossRef CAS.
- F. Tamaddon, Z. Razmi and A. A. Jafari, Tetrahedron Lett., 2010, 51, 1187 CrossRef CAS.
- P. Salehi, M. Dabiri, M. A. Zolfigol and M. A. B. Fard, Tetrahedron Lett., 2003, 44, 2889 CrossRef CAS.
- S. D. Salim and K. G. Akamanchi, Catal. Lett., 2011, 12, 1153 CrossRef CAS.
- S. Asghari, M. Tajbakhsh, B. Jafarzadeh-Kenari and S. Khaksar, Chin. Chem. Lett., 2011, 22, 127 CrossRef CAS.
- M. Zeinali-Dastmalbaf, A. Davoodnia, M. M. Heravi, N. Tavakoli-Hoseini, A. Khojastehnezhad and H. A. Zamani, Bull. Korean Chem. Soc., 2011, 32, 656 CrossRef CAS.
- S. Rostamnia and A. Morsali, RSC Adv., 2014, 4, 10514 RSC.
- J. Lu and H. Ma, Synlett, 2000, 63 CAS.
- X.-H. Chen, X.-Y. Xu, H. Liu, L.-F. Cun and L.-Z. Gong, J. Am. Chem. Soc., 2006, 128, 14802 CrossRef CAS PubMed.
- R. Javad-Kalbasi, A. R. Massah and B. Daneshvarnejad, Appl. Clay Sci., 2012, 55, 1 CrossRef.
- Y. Qiu, H. Sun, Z. Ma and W. Xia, J. Mol. Catal. A: Chem., 2014, 392, 76 CrossRef CAS.
- E. Ramu, V. Kotra, N. Bansal, N. Bansal, R. Varala and S. R. Adapa, J. Chem, 2008, 1, 188 CAS.
- K. Rad-Moghadam, S. C. Azimi and E. Abbaspour-Gilandeh, Tetrahedron Lett., 2013, 54, 4633 CrossRef CAS.
- E. Abbaspour-Gilandeh, M. Aghaei-Hashjin, A. Yahyazadeh and H. Salemi, RSC Adv., 2016, 6, 55444 RSC.
- E. Abbaspour-Gilandeh, S. C. Azimi and A. Mohammadi-Barkchai, RSC Adv., 2014, 4, 54854 RSC.
- E. Abbaspour-Gilandeh and S. C. Azimi, Iran. Chem. Commun., 2015, 3, 218 CAS.
- R. Ghosh, S. Maiti and A. Chakraborty, J. Mol. Catal. A: Chem., 2004, 217, 47 CrossRef CAS.
- Y. Ma, C. Qian, L. Wang and M. Yang, J. Org. Chem., 2000, 65, 3864 CrossRef CAS PubMed.
- D. Russowsky, F. A. Lopes, V. S. S. Silva, K. F. S. Canto, M. G. Montes and M. N. Godoi, J. Braz. Chem. Soc., 2004, 165 CrossRef CAS.
- A. D. Patil, N. V. Kumar, W. C. Kokke, M. F. Bean, A. J. Freyer, C. D. Brossi, S. Mai, A. Truneh, D. J. Faulkner, B. Carte, A. L. Breen, R. P. Hertzberg, R. K. Johnson, J. W. Westly and B. C. M. Potts, J. Org. Chem., 1995, 60, 1182 CrossRef CAS.
- N. S. Nandurkar, M. J. Bhanushali, M. D. Bhor and B. M. Bhanage, J. Mol. Catal. A: Chem., 2007, 271, 14 CrossRef CAS.
- J. S. Yadav, B. V. S. Reddy, R. Srinivas, C. Venugopal and T. Ramalingam, Synthesis, 2001, 1341 CrossRef CAS.
- K. K. Pasunooti, H. Chai, C. N. Jensen, B. K. Gorityala, S. Wang and X.-W. Liu, Tetrahedron Lett., 2011, 52, 80 CrossRef CAS.
- J. Safaei-Ghomi, R. Teymuri and A. Ziarati, Monatsh. Chem., 2013, 144, 1865 CrossRef CAS.
Footnote |
† Electronic supplementary information (ESI) available. See DOI: 10.1039/c7ra00794a |
|
This journal is © The Royal Society of Chemistry 2017 |
Click here to see how this site uses Cookies. View our privacy policy here.