DOI:
10.1039/C7RA00913E
(Paper)
RSC Adv., 2017,
7, 15112-15115
Highly emissive carbazole-based gold(I) complex with a long room-temperature phosphorescence lifetime and self-reversible mechanochromism characteristics†
Received
21st January 2017
, Accepted 20th February 2017
First published on 7th March 2017
Abstract
We report a highly emissive carbazole-based mononuclear gold(I) complex. Its solid shows a long room-temperature phosphorescence lifetime up to 29.91 ms. Furthermore, the luminogen exhibits self-reversible mechanochromic behavior.
The synthesis of efficient luminogenic materials is a hot and popular research topic.1 Of particular interest are those luminescent materials that exhibit a luminescence response to external mechanical stimuli because of their potential practical applications in various areas, such as fluorescence sensors, data storage and optoelectronic devices.2 In recent years, mechanical-stimuli-responsive materials have been generally regarded as extremely “smart” materials; this is because the external mechanical force is one of the most common stimuli, and it can be easily modulated.3 Bright solid-state luminescence and an obvious color contrast upon grinding are two very important factors for the practical application of mechanochromic materials.4 To date, a lot of mechanochromic luminogens based on organic compounds have been reported.5 In contrast, metal complexes with mechanochromic behavior are rare. In the last decade, gold(I) chemistry has attracted substantial interest,6 and gold(I) complexes with mechanochromism properties have received a lot of attention.7 However, the number of gold(I) complexes exhibiting mechanochromism behavior is still insufficient. Furthermore, the majority of reported mechanochromic gold(I) complexes are known to a contain pentafluorophenyl unit.8
It is well known that compounds with carbazole skeletons are very valuable candidates in the domain of optoelectronic devices.9 Unfortunately, the notorious aggregation-caused quenching (ACQ) phenomenon leads to the poor solid-state emission efficiency of numerous luminescent molecules.10 Indeed, the luminous efficiency of many carbazole-based luminescent molecules in the solid state generally decreases with the formation of crystalline state, which is disadvantageous for efficient application of these carbazole-based derivatives. To date, carbazole-based mechanochromic gold(I) complexes are quite scarce.11 Therefore, it is urgent and challenging to develop highly solid-state emissive carbazole-based mechanochromic gold(I) complexes. On the other hand, it is meaningful to synthesize luminescent molecules with a long room-temperature phosphorescence (RTP) lifetime.12 Meanwhile, it is also valuable for fundamental research and practical applications of mechanochromism materials to achieve spontaneous recovery of their mechanochromic luminescence without using any stimuli.13 For example, self-reversible mechanochromism materials can be used to realize dynamic random access memory (DRAM).14
In this article, we demonstrate a highly emissive carbazole-based gold(I) complex 1 with a long RTP lifetime (Scheme 1). The luminogen 1 exhibits mechanochromic behavior involving the luminescence changes between yellow and yellow-green emissions. More interestingly, the mechanochromic luminescence of 1 can be restored to the initial color spontaneously without applying other stimuli.
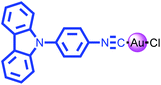 |
| Scheme 1 The structure of complex 1. | |
Gold(I) complex 1 was synthesized as a white solid in accordance with the simple and reasonable synthetic strategy shown in Scheme S1 (ESI†). Intermediate product 1-d as starting material reacted with AuCl(tht) (tht = thiophane) to afford the target mononuclear gold(I) complex 1 in 81% yield. The corresponding information about the synthetic procedures and characterization of luminogen 1 can be found in the ESI.†
We initially evaluated the luminescence behavior of 1 in various states. As can be seen in Fig. 1, luminogen 1 (1.0 × 10−5 mol L−1) in pure DMF exhibited very weak luminescence under 365 nm UV light, and its absolute luminescence quantum yield was 2.2%, likely due to the rotation of carbazole unit, which consumes the bulk of the energy of the excited state.15 However, the solid sample of 1 showed a bright yellow luminescence under 365 nm UV light with an absolute luminescence quantum yield up to 33.8%, the bright yellow emission of complex 1 in solid state is possibly triggered by the synergistic effects of the restricted intramolecular rotation of carbazole unit and the formation of intermolecular gold–gold interactions.16 As shown in Fig. 2, the solid sample of luminogen 1 was surveyed by scanning electron microscope (SEM), and the SEM image demonstrated the possible crystalline character of the corresponding solid powder. Next, the solid-state emission lifetime of 1 was tested. As presented in Fig. 3, the room-temperature solid-state emission lifetime of 1 was as high as 29.91 ms. Thus, the luminescence of 1 belonged to a long RTP emission.17 Multiple weak intermolecular interactions and intermolecular gold–gold interactions possible promote the formation of room-temperature phosphorescence behavior.18
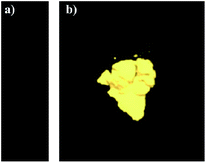 |
| Fig. 1 (a) Luminescence image of 1 (1.0 × 10−5 mol L−1) in pure DMF. Excitation wavelength: 365 nm. (b) Luminescence image of the as-synthesized solid sample 1. Excitation wavelength: 365 nm. | |
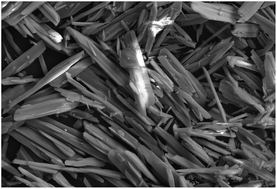 |
| Fig. 2 SEM image (20 μm) of the solid sample 1. | |
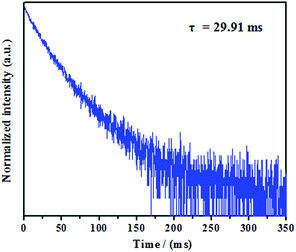 |
| Fig. 3 Decay curve of emission at 546 nm. Delay time = 0.2 ms. Excitation wavelength: 365 nm. | |
Subsequently, the mechanochromic behavior of 1 was investigated by solid-state photoluminescence (PL) spectroscopy. As shown in Fig. 4, the luminescence spectrum of solid sample 1 showed two emission bands with λmax at 436 nm, 460 nm, 546 nm and 594 nm, corresponding to a bright yellow emission under irradiation with 365 nm UV light. Interestingly, after mechanical grinding using a mortar, the yellow-emitting solid sample is changed into a yellow-green-emitting solid sample, and two emission bands with λmax at 468 nm, 546 nm and 594 nm were observed.
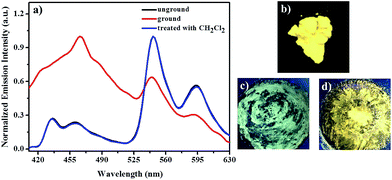 |
| Fig. 4 (a) PL spectra of complex 1 before grinding, after grinding, and after treatment with dichloromethane vapor. Excitation wavelength: 365 nm. Photographic images of luminogen 1 under 365 nm UV light: (b) the as-synthesized sample. (c) The entirely ground sample. (d) The sample after treatment with dichloromethane vapor. | |
After fuming with dichloromethane solvent vapor for 30 s, the initial intense yellow luminescence reappeared. In addition, this mechanochromic luminescence transition of 1 can be repeated many times between the yellow luminescence and yellow-green luminescence by alternating grinding and fuming processes (Fig. 5). More importantly, as can be seen in Fig. 6, the yellow-green-emitting solid powder can be robustly self-recovered back to yellow-emitting solid powder within 10 h, and two new emission bands with λmax at 472 nm, 564 nm and 596 nm can be observed clearly. This self-reversible mechanochromic phenomenon is very beneficial for realizing volatile optical memory.
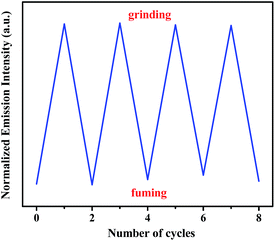 |
| Fig. 5 Reversible grinding–fuming processes of the photoluminescence of complex 1 at 468 nm. | |
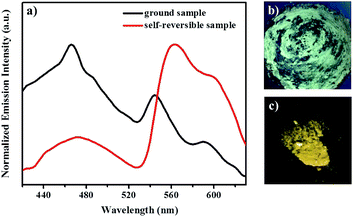 |
| Fig. 6 (a) PL spectra of complex 1 at different conditions. Excitation wavelength: 365 nm. Photographic images of luminogen 1 under 365 nm UV light: (b) the entirely ground sample; (c) 10 h after grinding. | |
It is conjectured that the corresponding mechanochromic mechanism should be related with the transformation of molecular morphology. In order to further verify this proposed mechanism responsible for the observed mechanochromism phenomenon, the structural transformation of the powder sample of luminogen 1 was tested by X-ray diffraction (XRD) measurements. As shown in Fig. 7, the XRD pattern for the freshly synthesized form of 1 exhibited a lot of sharp and intense peaks, which suggested the crystalline nature of the initial solid sample. As a consequence, 1 exhibited obvious crystallization-induced emission enhancement (CIEE) behavior. In contrast, after grinding, the intense crystalline peaks disappeared and a pattern with weak diffraction peaks was observed, indicating the amorphous nature of the ground sample. Moreover, upon treatment of ground solid sample 1 with dichloromethane solvent vapor, the recovery of the amorphous-to-crystal phase could be realized. Hence, the powder XRD measurement results indicate that the mechanochromic property of 1 is due to the switchable morphology transition between the crystalline and amorphous phases.
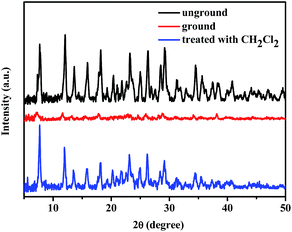 |
| Fig. 7 XRD patterns of luminogen 1: unground, ground and after treatment with dichloromethane vapor. | |
In summary, a carbazole-based gold(I) complex was synthesized. This luminogen exhibits a bright yellow luminescence and a long RTP lifetime, which is as high as 29.91 ms. Furthermore, it also shows reversible mechanochromic behavior involving the luminescence changes between yellow and yellow-green emissions. More interestingly, the mechanochromic yellow-green luminescence of 1 can be restored to the initial yellow color spontaneously without using other stimuli. To the best of our knowledge, this carbazole-based mononuclear gold(I) complex is the first example of metal-bearing luminogen with a long RTP lifetime and self-reversible mechanochromic behavior. Further studies on the design of new luminogens with a long RTP lifetime and self-reversible multi-stimuli responsive behaviors are in progress.
Acknowledgements
The authors are grateful for the financial support from the National Natural Science Foundation of China (51373072, 201362013, 21662015, and 21262015), the Project of Jiangxi Sci-Tech Innovative Team (20142BCB24012), the Science Fund of Natural Science Foundation of Jiangxi Province (20142BAB203005), and the Project of the Science Fund of Jiangxi Education Office (GJJ160764).
Notes and references
-
(a) A. C. Grimsdale, K. L. Chan, R. E. Martin, P. G. Jokisz and A. B. Holmes, Chem. Rev., 2009, 109, 897–1091 CrossRef CAS PubMed;
(b) Y. Hong, J. W. Y. Lam and B. Z. Tang, Chem. Soc. Rev., 2011, 40, 5361–5388 RSC;
(c) J. Mei, Y. Hong, J. W. Y. Lam, A. Qin, Y. Tang and B. Z. Tang, Adv. Mater., 2014, 26, 5429–5479 CrossRef CAS PubMed;
(d) Z. Chen, D. Wu, X. Han, J. Liang, J. Yin, G.-A. Yu and S. H. Liu, Chem. Commun., 2014, 50, 11033–11035 RSC;
(e) Z. Chen, D. Wu, X. Han, Y. Nie, J. Yin, G.-A. Yu and S. H. Liu, RSC Adv., 2014, 4, 63985–63988 RSC;
(f) Z. Chen, J. Liang, X. Han, J. Yin, G.-A. Yu and S. H. Liu, Dyes Pigm., 2015, 112, 59–66 CrossRef CAS;
(g) Z. Chen, X. Han, J. Zhang, D. Wu, G.-A. Yu, J. Yin and S. H. Liu, RSC Adv., 2015, 5, 15341–15349 RSC.
-
(a) Z. Chi, X. Zhang, B. Xu, X. Zhou, C. Ma, Y. Zhang, S. Liu and J. Xu, Chem. Soc. Rev., 2012, 41, 3878–3896 RSC;
(b) G.-G. Shan, H.-B. Li, J.-S. Qin, D.-X. Zhu, Y. Liao and Z.-M. Su, Dalton Trans., 2012, 41, 9590–9593 RSC;
(c) X. Zhang, Z. Chi, Y. Zhang, S. Liu and J. Xu, J. Mater. Chem. C, 2013, 1, 3376–3390 RSC;
(d) S. Xue, X. Qiu, Q. Sun and W. Yang, J. Mater. Chem. C, 2016, 4, 1568–1578 RSC.
- M.-J. Teng, X.-R. Jia, S. Yang, X.-F. Chen and Y. Wei, Adv. Mater., 2012, 24, 1255–1261 CrossRef CAS PubMed.
-
(a) Y. Qi, Y. Wang, Y. Yu, Z. Liu, Y. Zhang, G. Du and Y. Qi, RSC Adv., 2016, 6, 33755–33762 RSC;
(b) H.-J. Kim, D. R. Whang, J. Gierschner, C. H. Lee and S. Y. Park, Angew. Chem., Int. Ed., 2015, 54, 4330–4333 CrossRef CAS PubMed.
-
(a) Y. Dong, B. Xu, J. Zhang, X. Tan, L. Wang, J. Chen, H. Lv, S. Wen, B. Li, L. Ye, B. Zou and W. Tian, Angew. Chem., Int. Ed., 2012, 51, 10782–10785 CrossRef CAS PubMed;
(b) R. Misra, T. Jadhav, B. Dhokale and S. M. Mobin, Chem. Commun., 2014, 50, 9076–9078 RSC;
(c) G.-F. Zhang, M. P. Aldred, Z.-Q. Chen, T. Chen, X. Meng and M.-Q. Zhu, RSC Adv., 2015, 5, 1079–1082 RSC;
(d) B. Xu, J. He, Y. Mu, Q. Zhu, S. Wu, Y. Wang, Y. Zhang, C. Jin, C. Lo, Z. Chi, A. Lien, S. Liu and J. Xu, Chem. Sci., 2015, 6, 3236–3241 RSC;
(e) J. Wei, B. Liang, X. Cheng, Z. Zhang, H. Zhang and Y. Wang, RSC Adv., 2015, 5, 71903–71910 RSC;
(f) J. Yang, Q. Guo, X. Wen, X. Gao, Q. Peng, Q. Li, D. Ma and Z. Li, J. Mater. Chem. C, 2016, 4, 8506–8513 RSC;
(g) R. M. Ongungal, A. P. Sivadas, N. S. Saleesh Kumar, S. Menon and S. Das, J. Mater. Chem. C, 2016, 4, 9588–9597 RSC.
-
(a) W. J. Hunks, M. C. Jennings and R. J. Puddephatt, Inorg. Chem., 2000, 39, 2699–2702 CrossRef CAS PubMed;
(b) G. J. Hutchings, M. Brust and H. Schmidbaur, Chem. Soc. Rev., 2008, 37, 1759–1765 RSC;
(c) R. Benavente, P. Espinet, S. Lentijo, J. M. Martín-Álvarez, J. A. Miguel and M. P. Rodríguez-Medina, Eur. J. Inorg. Chem., 2009, 2009, 5399–5406 CrossRef;
(d) W. Lu, W.-M. Kwok, C. Ma, C. T.-L. Chan, M.-X. Zhu and C.-M. Che, J. Am. Chem. Soc., 2011, 133, 14120–14135 CrossRef CAS PubMed;
(e) E. R. T. Tiekink, Coord. Chem. Rev., 2014, 275, 130–153 CrossRef CAS.
-
(a) M. Osawa, I. Kawata, S. Igawa, M. Hoshino, T. Fukunaga and D. Hashizume, Chem.–Eur. J., 2010, 16, 12114–12126 CrossRef CAS PubMed;
(b) H. Ito, M. Muromoto, S. Kurenuma, S. Ishizaka, N. Kitamura, H. Sato and T. Seki, Nat. Commun., 2013, 4, 2009–2013 Search PubMed;
(c) T. Seki, K. Sakurada and H. Ito, Angew. Chem., Int. Ed., 2013, 52, 12828–12832 CrossRef CAS PubMed;
(d) P. Baranyai, G. Marsi, C. Jobbágy, A. Domján, L. Oláh and A. Deák, Dalton Trans., 2015, 44, 13455–13459 RSC;
(e) T. Seki, T. Ozaki, T. Okura, K. Asakura, A. Sakon, H. Uekusa and H. Ito, Chem. Sci., 2015, 6, 2187–2195 RSC.
-
(a) H. Ito, T. Saito, N. Oshima, N. Kitamura, S. Ishizaka, Y. Hinatsu, M. Wakeshima, M. Kato, K. Tsuge and M. Sawamura, J. Am. Chem. Soc., 2008, 130, 10044–10045 CrossRef CAS PubMed;
(b) J. Liang, F. Hu, X. Lv, Z. Chen, Z. Chen, J. Yin, G.-A. Yu and S. H. Liu, Dyes Pigm., 2012, 95, 485–490 CrossRef CAS;
(c) J. Liang, Z. Chen, L. Xu, J. Wang, J. Yin, G.-A. Yu, Z.-N. Chen and S. H. Liu, J. Mater. Chem. C, 2014, 2, 2243–2250 RSC;
(d) Z. Chen, P.-S. Huang, Z. Li, J. Yin, G.-A. Yu and S. H. Liu, Inorg. Chim. Acta, 2015, 432, 192–197 CrossRef CAS;
(e) Z. Chen, J. Zhang, M. Song, J. Yin, G.-A. Yu and S. H. Liu, Chem. Commun., 2015, 51, 326–329 RSC;
(f) Z. Chen, Z. Li, L. Yang, J. Liang, J. Yin, G.-A. Yu and S. H. Liu, Dyes Pigm., 2015, 121, 170–177 CrossRef CAS;
(g) Z. Chen, Y. Nie and S. H. Liu, RSC Adv., 2016, 6, 73933–73938 RSC.
-
(a) H. Huang, Y. Wang, B. Wang, S. Zhuang, B. Pan, X. Yang, L. Wang and C. Yang, J. Mater. Chem. C, 2013, 1, 5899–5908 RSC;
(b) C. Tang, R. Bi, Y. Tao, F. Wang, X. Cao, S. Wang, T. Jiang, C. Zhong, H. Zhang and W. Huang, Chem. Commun., 2015, 51, 1650–1653 RSC.
- J. Mei, N. L. C. Leung, R. T. K. Kwok, J. W. Y. Lam and B. Z. Tang, Chem. Rev., 2015, 115, 11718–11940 CrossRef CAS PubMed.
-
(a) Z. Chen, L. Yang, Y. Hu, D. Wu, J. Yin, G.-A. Yu and S. H. Liu, RSC Adv., 2015, 5, 93757–93764 RSC;
(b) Z. Chen, J. Liang, Y. Nie, X. Xu, G.-A. Yu, J. Yin and S. H. Liu, Dalton Trans., 2015, 44, 17473–17477 RSC;
(c) Z. Chen, Z. Li, F. Hu, G.-A. Yu, J. Yin and S. H. Liu, Dyes Pigm., 2016, 125, 169–178 CrossRef CAS.
-
(a) Z. An, C. Zheng, Y. Tao, R. Chen, H. Shi, T. Chen, Z. Wang, H. Li, R. Deng, X. Liu and W. Huang, Nat. Mater., 2015, 14, 685–690 CrossRef CAS PubMed;
(b) Y. Gong, G. Chen, Q. Peng, W. Z. Yuan, Y. Xie, S. Li, Y. Zhang and B. Z. Tang, Adv. Mater., 2015, 27, 6195–6201 CrossRef CAS PubMed;
(c) P. Xue, J. Sun, P. Chen, P. Wang, B. Yao, P. Gong, Z. Zhang and R. Lu, Chem. Commun., 2015, 51, 10381–10384 RSC.
- P. S. Hariharan, N. S. Venkataramanan, D. Moon and S. P. Anthony, J. Phys. Chem. C, 2015, 119, 9460–9469 CAS.
- D. Tu, P. Leong, Z. Li, R. Hu, C. Shi, K. Y. Zhang, H. Yan and Q. Zhao, Chem. Commun., 2016, 52, 12494–12497 RSC.
- J. Luo, Z. Xie, J. W. Y. Lam, L. Cheng, H. Chen, C. Qiu, H. S. Kwok, X. Zhan, Y. Liu, D. Zhu and B. Z. Tang, Chem. Commun., 2001, 1740–1741 RSC.
- J. Liang, Z. Chen, J. Yin, G.-A. Yu and S. H. Liu, Chem. Commun., 2013, 49, 3567–3569 RSC.
-
(a) M. Osawa, I. Kawata, S. Igawa, A. Tsuboyama, D. Hashizume and M. Hoshino, Eur. J. Inorg. Chem., 2009, 2009, 3708–3711 CrossRef;
(b) Z. Yang, Z. Mao, X. Zhang, D. Ou, Y. Mu, Y. Zhang, C. Zhao, S. Liu, Z. Chi, J. Xu, Y.-C. Wu, P.-Y. Lu, A. Lien and M. R. Bryce, Angew. Chem., Int. Ed., 2016, 55, 2181–2185 CrossRef CAS PubMed.
- G. Bergamini, A. Fermi, C. Botta, U. Giovanella, S. D. Motta, F. Negri, R. Peresutti, M. Gingras and P. Ceroni, J. Mater. Chem. C, 2013, 1, 2717–2724 RSC.
Footnote |
† Electronic supplementary information (ESI) available: Detailed experimental section, NMR spectra, mass spectra and characterization datas mentioned in the paper. See DOI: 10.1039/c7ra00913e |
|
This journal is © The Royal Society of Chemistry 2017 |
Click here to see how this site uses Cookies. View our privacy policy here.