DOI:
10.1039/C7RA01111C
(Paper)
RSC Adv., 2017,
7, 34699-34704
Evaluation of the anti-inflammatory properties of telmesteine on inflammation-associated skin diseases†
Received
25th January 2017
, Accepted 22nd June 2017
First published on 11th July 2017
Abstract
Telmesteine, a useful agent for respiratory tract disorders, has been reported to be a critical active ingredient in topical compositions for dermatitis. The present study was designed to elucidate the in vitro and in vivo anti-inflammatory properties of telmesteine. LPS-induced NO production in RAW264.7 cells was significantly decreased after telmesteine treatment. TPA-induced skin inflammation as assessed by skin edema and pro-inflammatory cytokines (IL-1β, IL-6, and TNF-α) was significantly decreased after telmesteine topical treatment. Further analysis results demonstrated that the anti-inflammation properties of telmesteine were associated with its ability to inhibit activation of nuclear factor kappa-κB (NF-κB) by blocking phosphoinositide 3-kinase/protein kinase B (PI3K/Akt)/IκB kinase (IKK) activities.
Introduction
Skin is the inherent barrier between the human body and the environment and one of its major functions is to defend the body from various intrinsic or extrinsic stimuli.1 When stimulated, the structure and function of skin are changed, which is usually translated into inflammation.2 It has been established that inflammation is associated with various skin diseases such as atopic dermatitis, psoriasis, rosacea, and cancer.3 Inflammation is a complex pathophysiological process.4,5 Various signaling molecules produced by activated leukocytes and nuclear factor-κB (NF-κB) have a critical role in the inflammatory process.6,7
The mouse skin model has been widely used to study the molecular changes implicated in skin disease. 12-O-Tetradecanoylphorbol-13-acetate (TPA), a potent tumor promoter, induces skin inflammation, edema, and epidermal hyperplasia.8,9 Topical application of TPA on mouse skin up-regulates the gene expression of proliferation and inflammation including cyclooxygenase (COX-2), inducible nitric oxide synthase (iNOS), and ODC. Experimental evidence has shown that TPA induces inflammatory expression by the activation of NF-κB, through activation of inhibitor κB (IκB) kinases. Up-regulation of phosphatidylinositol 3-kinase (PI3K)/AKT signaling also involves TPA-stimulated NF-κB transcriptional activity.
For many cutaneous diseases associated with chronic inflammation, such as acne, rosacea and atopic dermatitis, symptonic management of skin flare-up by anti-inflammatory agents is beneficial for quality of life. However, long term application of steroids is constricted due to side effects of skin atrophy, increased opportunistic infections and steroid-induced dermatitis.10 Use of calcenurin inhibitors like tacrolimus and pimecrolimus overcame some drawbacks of steroids, but their long term side effects are still unknown, although study indicated that up to 4 years of tacrolinus treatment of atopic dermatitis is safe.11 As a consequence, anti-inflammatory agents with reduced or little immunosuppression may be an option for chronic cutaneous inflammations therapy algorithm.
Telmesteine (Fig. 1A), ((−)-3-ethyl hydrogen (R)-3,4-thiazolidinedicarboxylate) has been conventionally used as a mucolytic in the treatment of respiratory-tract disorders through oral administration of 300 mg dosage two or three times daily.12 Telmestaine also inhibits elastase and collagenase and may be useful in the treatment of skin aging and psoriasis.13,14 In recent years, telmesteine has been incorporated into topical formulations for dermatitis.14,15 However, the mechanisms underlying these topical anti-inflammatory effects of telmesteine on TPA-induced skin inflammation remain to be elucidated.
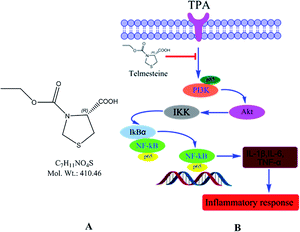 |
| Fig. 1 Chemical structure (A) and a model of telmesteine modulation of TPA-induced signal transduction pathways in mouse skin in vivo (B). | |
Herein, we investigate some of the in vitro and in vivo anti-inflammatory properties of telmesteine. Our results showed that telmesteine treatment resulted in an obviously decline of LPS-induced NO production in RAW264.7 cells and TPA-induced skin inflammation as assessed by skin edema and pro-inflammatory cytokines interleukin (IL)-1β, IL-6, and necrosis factor-alpha (TNF-α). Further analysis results demonstrated that the anti-inflammation properties of telmesteine were associated with its ability to inhibit activation of NF-κB by blocking phosphoinositide 3-kinase/protein kinase B (PI3K/Akt)/IκB kinase (IKK) activities.
Material and methods
Material
Telmesteine (99%) was purchased from BOC Sciences. TPA was obtained from the Henan Cancer Hospital (Henan, China). IL-1β, IL-6, TNF-α, anti-rabbit, anti-mouse horseradish peroxidase (HRP)-conjugated secondary antibodies, anti-Ser536 p65, antiphospho-Ser536 p65 antibodies, anti-Ser32 IκB-α, anti-phospho-Ser32 IκB-α, anti-IKK, anti-Ser473 Akt, antiphospho-Ser473 Akt, anti-PI3K p85 and anti-phospho-PI3K p85 were purchased from Beyotime Biotechnology Co. (Beijing, China). The other chemicals used were in the purest form available commercially.
Effects of telmesteine on lipopolysaccharide (LPS)-induced NO in LPS-induced RAW264.7 cells
MTT and NO assays were carried out to evaluate the in vitro anti-inflammatory properties of telmesteine. RAW264.7 cells (2.0 × 105 cells per mL) were cultured for 1 d, followed by LPS (100 ng mL−1) treatment in the presence of different concentrations of telmesteine (5, 10, 15, 20 μg mL−1). After incubation for 1 d, an MTT stock solution was added to the medium, followed by incubation for 1 h. The absorbance was measured at 540 nm. The nitrite as a stable end product of NO was measured using the Griess reaction. Sample (100 μL) mixed with Griess reagent 100 μL (0.1% N-(1-naphthyl) ethylenediamide dihydrochloride and 1% sulfanilamide in 5% phosphoric acid), followed by spectrophotometric measurement at 550 nm. Nitrite concentrations in supernatants were counted based on the sodium nitrite standard curve.
Animals
Female BALB/c mices at 5–6 weeks old were obtained from Guangdong Medical Laboratory Animal Center (Guangzhou, China). All animals were housed in a controlled atmosphere (25 ± 1 °C at 50% relative humidity) and with a 12 h light–12 h dark cycle. Animals had free access to food and water at all times. Animals were maintained in accordance with the guidelines of the Guangdong Medical Laboratory Animal Center, China, and approved by the institutional ethical committee (IEC) of Wuyi University.
TPA-induced ear edema in mice
For TPA-induced mouse ear edema model,16,17 both mice ears were topical treated with acetone or telmesteine with different concentrations respectively, 30 min prior to TPA (0.008 nM in acetone) treatment. Then the mice were sacrificed after 6 h. Finally, ear punches of 6 mm diameter from mice ears were taken and weighed. The inhibitory effects (IE) = [(TPA alone) − (test compound plus TPA)]/[(TPA alone) − (acetone alone)] × 100%.
Histological appearance of mouse ears
Mice ears were removed in toto, fixed in 10% formalin, decalcified in EDTA buffer, subjected to a series progression of dehydration, and then embedded in paraffin. Samples were serially sectioned into 4 μm and processed routinely for hematoxylin and eosin (H&E) staining. The histological changes were observed under a microscope.
Determination of IL-1β, IL-6 and TNF-α
The expression of IL-1β, IL-6 and TNF-α were measured as described previously.16,17 Briefly, after incubated with 1.2% H2O2 in PBS, the deparaffinized skin sections were treated with the primary antibody of proliferating cell nuclear antigen at 4 °C overnight. After washed with PBS, the sections were incubated with a biotin-conjugated horseradish peroxidase antibody at 25 °C for one hour. Finally, the 3,3-diaminobenzidine tetrahydrochloride reactions were applied to produce the brown label in the epidermal tissue. The peroxidase was detected. For each section, the numbers of positive staining cells were counted in five different fields at both ends as well as in the middle.
Assays of p65, p-p65, IKK, IκBα, p-IκBα, PI3k p85, p-PI3k p85, AKt and p-AKt
The assay of NF-κB transcriptional activity and PI3K/Akt/IKK signaling pathway were detected as described in our previous work.18 Briefly, the paraffin skin sections were made and then processed routinely for staining. The histological changes were examined under microscope.
Scoring the expression of biomarkers
For each sample, ≥5 ducts per histologic type were scored independently by two experienced investigators not aware of the identity of the specimens (×200). Expression of IL-1β, IL-6, TNF-α, p65, p-p65, IκBα, p-IκBα, IKK, Akt, p-Akt, PI3K p85, and p-PI3K p85 were detected by the following semi-quantitative scoring system.19 The intensity of staining was scored as follows: 0, no staining; 1+, faint; 2+, moderate; 3+, strong. 1+, 2+, and 3+ were recorded as 1, 2, and 3 points, respectively. The extent of staining was graded as follows: 0, no staining; 1+, ≤25% of cells positive; 2+, 26% to 50% of cells positive; 3+, ≥51% of cells positive.20
Statistical analysis
The results are presented as mean ± standard error of mean (S.E.M.). The statistical significance between the groups was calculated by one-way analysis of variance ANOVA followed by Dunnett t test. P values less than 0.05 (P < 0.05), were considered indicative of significance.
Results and discussion
Effects of telmesteine on LPS-induced NO release
NO, an important information molecule, is synthesized by NO synthase (NO) catalyzing L-arginine. In the inflammatory process, activated macrophages secrete large amounts of NO, which results in the injury of the surrounding tissue. The in vitro anti-inflammatory property of telmesteine was determined in LPS-induced RAW264.7 cells. As shown in Fig. 2A, telmesteine at concentration of 5, 10, 15, and 20 μg mL−1 had no obvious effect on RAW264.7 cells viability after LPS treatment (100 ng mL−1). Compared with controls, RAW264.7 cells treated with LPS alone showed significant induction of NO, while telmesteine treatment could significantly decreased the LPS-induced NO in RAW264.7 (Fig. 2B).
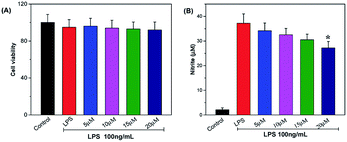 |
| Fig. 2 Effects of telmesteine on LPS-induced cell viability (A) and NO in LPS-induced RAW264.7 cells (B). Data was expressed as mean ± S.E.M. (n = 3) *P < 0.05 was compared with LPS-treated group. | |
Effects of telmesteine on TPA-induced mouse ear edema
The first hallmark of skin inflammation skin is the increased skin thickening, which also is an indicator of processes occurring in skin inflammation, such as edema, increased vascular permeability, and proliferation of epidermal keratinocytes.21,22 The in vivo anti-inflammatory effect of telmesteine was evaluated in a TPA-induced mouse ear edema model. As shown in Fig. 3, topical application of TPA (0.008 nM) alone would result in a markedly increase of mouse ear edema, then the average weight of TPA-treated ear punches increases from 6.8 mg to 17.3 mg. Notably, topical application of telmesteine (5–20 μM) markedly inhibited TPA-induced skin inflammation, with dose-dependently affecting ear edema. Importantly, the 20 μM dose caused a 90.6% decrease in the average weight of the ear punches.
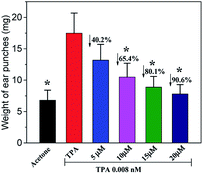 |
| Fig. 3 Effects of telmesteine on TPA-induced mouse ears edema. The values were represented as the mean ± S.E.M. (n = 3). *P < 0.05 was compared with TPA-treated mice. | |
Histological appearance of mouse ears
Fig. 4 illustrated the histological appearances of acetone, TPA, and telmesteine (5–20 μM) groups, respectively. Compared to acetone-treated mice (Fig. 3a), topical application of TPA alone (Fig. 3b) caused an obviously inflammation response with clear evidences of ear edema and inflammatory cells infiltration. Moreover, topical application of telmesteine (5–20 μM) (Fig. 4c–f) significantly decreased inflammatory cells infiltration. And telmesteine at 20 μM caused the most markedly morphological alterations, compared to TPA treated alone (Fig. 4f).
 |
| Fig. 4 Histological changes of TPA-induced mouse ear pretreated with apigenin (5–20 μM). Magnification 200×. | |
Effect of telmesteine on TPA-induced pro-inflammatory cytokines expressions
Cytokines are produced by cells induced with immunogen, mitogen and other stimulants. They have a variety of functions on regulation of immunity, hematopoiesis, cell growth and damaged tissue repairation.23–25 The pro-inflammatory cytokines produce various signaling molecules, which mediate pathogenesis of inflammation.26–28 Thus we assessed the inhibitory effects of telmesteine by analyzing the expression changes of IL-1β, IL-6 and TNF-α using immunohistochemical analysis. As illustrated in Fig. 4b, topical application of TPA obviously increased the expressions of IL-1β, IL-6 and TNF-α compared with acetone-treated mice. In contrast, pretreatments with telmesteine notably reduced the expressions of TPA-reduced IL-1β, IL-6 and TNF-α (Fig. 5c–f). In addition, the IL-1β, IL-6 and TNF-α upregulation induced by TPA administration were significantly inhibited by topical treated with telmesteine (20 μM).
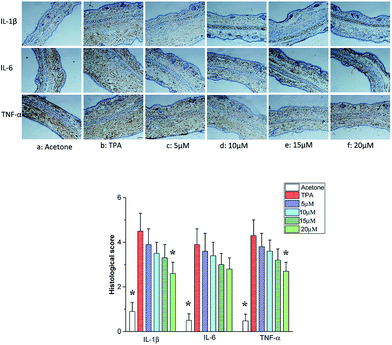 |
| Fig. 5 Effects of telmesteine on TPA-induced expressions of IL-1β, IL-6 and TNF-α. Magnification 200×. Data was expressed as mean ± S.E.M. (n = 3) *P < 0.05 was compared with TPA-treated mice. | |
Effects of telmesteine on TPA-induced NF-κB activation by targeting IKK
NF-κB contained with Rel, p65, RelB, p50 and p52 is a early transcription factor can response to the harmful stimuli on cells at the first time. NF-κB has been recognized as one of the most important molecular targets in the prevention of inflammation and cancer.29,30 Many molecules involved in inflammation are regulated by NF-κB, including TNF-α, IL-1β, IL-2, IL-6, IL-8, IL-12, iNOS and COX-2. Notably, numerous reports have demonstrated that the increase of nuclear factor NF-κB activation plays a critical role in the development and maintenance of cutaneous inflammatory diseases, such as psoriasis, atopic dermatitis, rosacea, and contact dermatitis.31,32 Therefore, drugs with inhibition on nuclear factor NF-κB activation are potential interest in the treatment of skin inflammatory diseases. Thus, we evaluated the effects of telmesteine on TPA-stimulated NF-κB activation in mouse skin. After stimulated by TPA (Fig. 6b), one of its functional active subunit-p65 mainly distributed in the cytoplasm is markedly evoked nuclear translocation to the nucleus.33,34 However the significantly increased expression of p65 is strongly inhibited by telmesteine pretreatment in a dose-dependent manner (Fig. 6c–f). Furthermore, telmesteine administration also significantly reduced the expression of phosphor-p65, which contributes to its transcriptional activity (Fig. 6c–f). These results indicated that telmesteine significantly suppressed TPA-stimulated NF-κB promoter activity. Also the inhibition of NF-κB activation by telmesteine might explain its effects on the expression of IL-1β, IL-6 and TNF-α in mouse skin induced by TPA.
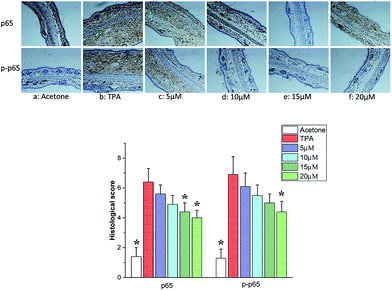 |
| Fig. 6 Effects of telmesteine on TPA-induced expressions of p65 and p-p65. Magnification 200×. Data was expressed as mean ± S.E.M. (n = 3) *P < 0.05 was compared with TPA-treated mice. | |
Several experimental evidences convincingly certificate that phosphorylation of IκB proteins including IκBα is essential for the activation of NF-κB. IκB degradation evokes NF-κB to translocate to the nucleus. The IκB kinase (IKK) is a complex of IKKα, IKKβ and IKKγ, which plays important roles in phosphorylating IκBα and activating NF-κB.35–37 As shown in Fig. 7b, TPA treatment resulted in the phosphorylation of IκBα protein accompanied with its degradation. Telmesteine pretreatment significantly suppressed the degradation and phosphorylation of IκBα (Fig. 7c–f). Likewise, telmesteine pretreatment significantly inhibited the TPA-induced IκB kinases (IKK) activity in a dose-dependent manner (Fig. 7c–f).
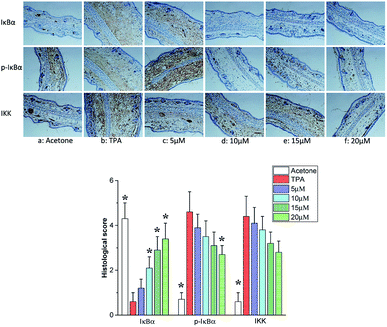 |
| Fig. 7 Effects of telmesteine on TPA-induced expressions of IκBα, p-IκBα and IKK. Magnification 200×. Data was expressed as mean ± S.E.M. (n = 3) *P < 0.05 was compared with TPA-treated mice. | |
Inhibitory effects of telmesteine on TPA-induced activation of PI3K/Akt
NF-κB molecule is also known to be regulated by PI3K/Akt signaling pathway, besides the regulation by IκBα and IKK. PI3K, an intracellular phosphatidylinositol kinase constituted with regulatory subunit of P85 and catalytic subunit of p110 plays an important role in the pathogenesis of inflammatory, tumor and metabolic diseases.38,39 AKT called protein kinase B is the direct target protein of PI3K.40 Activated PI3K/Akt signaling pathway results in the NF-κB activation though the enhancement of IκB phosphorylation and degradation.41 Furthermore, PI3K/Akt signaling pathway is closely related to the regulation and release of cytokines such as IL, TNF-α and IFN.42 Herein, the effects of telmesteine on PI3K/Akt signaling pathway were investigated. As shown in Fig. 8, TPA application caused markedly increases on expressions of p85, p-p85, Akt, and p-Akt. All our above data indicated that telmesteine exerts its anti-inflammatory properties by inhibiting activation of NF-κB by blocking PI3K/Akt/IKK activities (Fig. 1B).
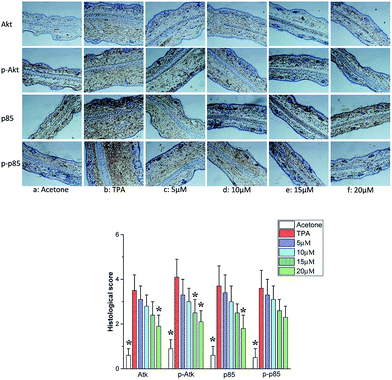 |
| Fig. 8 Effects of telmesteine on TPA-induced expressions of p85, p-p85, Akt and p-Akt. Magnification 200×. Data was expressed as mean ± S.E.M. (n = 3) *P < 0.05 was compared with TPA-treated mice. | |
Summary
In summary, the present study provides evidence that telmesteine exhibits strong anti-inflammatory properties. Telmesteine treatment could decrease LPS-induced NO production in RAW264.7 cells. Also telmesteine treatment could inhibit TPA-induced inflammatory (skin edema and pro-inflammatory cytokines (IL-1β, IL-6, and TNF-α)) in mice. In addition, our data indicated that telmesteine exerts its anti-inflammatory properties by inhibiting activation of NF-κB by blocking PI3K/Akt/IKK activities. All results suggests telmesteine can be used as a potent anti-inflammatory agent for skin inflammatory and anti-inflammatory materials in cosmetics. Currently, the development of application of telmesteine in cosmetics is ongoing in our lab.
Acknowledgements
Financial support was provided by the Natural Science Foundation of Guangdong Province (2016A030310441 and 2016A030313006), the Youth Team Fund of Wuyi University (2016td01), and Youth Fund of Wuyi University (2015zk03 and 2015zk05).
Notes and references
- R. Medeiros, M. F. Otuki, M. C. W. Avellar and J. B. Calixto, Eur. J. Pharmacol., 2007, 559, 227–235 CrossRef CAS PubMed.
- A. K. Maurya, M. Singh, V. Dubey, S. Srivastava, S. Luqman and D. U. Bawankule, Curr. Pharm. Biotechnol., 2014, 15, 173–181 CAS.
- A. B. Gokhle, A. S. Damre, K. R. Kulkarni and M. N. Saraf, Phytomedicine, 2002, 9, 433–437 CrossRef.
- J. Wu and C. C. Chu, J. Mater. Chem. B, 2013, 1, 353–360 RSC.
- J. Wu and C. C. Chu, Acta Biomater., 2012, 8, 4314–4323 CrossRef CAS PubMed.
- G. K. Kumar, R. Dhamotharan, N. M. Kulkarni, M. Y. A. Mahat, J. Gunasekaran and M. Ashfaque, Eur. J. Pharmacol., 2011, 662, 63–69 CrossRef PubMed.
- J. K. Kundu, Y. K. Shin and Y. J. Surh, Biochem. Pharmacol., 2006, 72, 1506–1515 CrossRef CAS PubMed.
- H. Hvid, I. Teige, P. H. Kvist, L. Svensson and K. Kemp, Int. Immunol., 2008, 20, 1097–1106 CrossRef CAS PubMed.
- M. Zielińska, T. B. Haddou, G. Cami-Kobeci, M. Sałaga, A. Jarmuż, M. Padysz, R. Kordek, M. Spetea, S. M. Husbands and J. Fichna, Eur. J. Pharmacol., 2015, 765, 582–590 CrossRef PubMed.
- K. Hoffmann, T. Auer, M. Stiicker, A. Hoffmann and P. Altmeyer, J. Eur. Acad. Dermatol. Venereol., 1998, 10, 137–142 CrossRef CAS PubMed.
- J. M. Hanifin, A. S. Paller, L. Eichenfield, R. Clark, N. Korman, G. Weinstein, I. Caro, E. Jaracz and M. J. Rico, J. Am. Acad. Dermatol., 2005, 53, S186–S194 CrossRef PubMed.
- S. C. Sweetman, B. Pharm and F. R. Pharm, The Pharmaceutical Press, 2009, p. 1573 Search PubMed.
- C. Rumio and M. Mastrodonato, J. Am. Acad. Dermatol., 2008, 58, AB22 CrossRef.
- R. Narins, C. Maas, Z. P. Lorenc and G. D. Monheit, J. Am. Acad. Dermatol., 2008, 58, AB19 Search PubMed.
- M. Mastrodonato, US Pat. 8431601 B2, 2013.
- V. Stefano, D. M. Paolo, S. Rossana and L. Luisa, J. Drugs Dermatol., 2009, 8, 537–539 Search PubMed.
- W. F. Liu, Y. L. Li, K. Zhang and Z. Y. Du, Food Funct., 2015, 6, 3712–3719 CAS.
- W. F. Liu, Y. L. Li, Y. Yue, K. Zhang, Q. Chen, H. Q. Wang, Y. J. Lu, M. T. Huang, X. Zheng and Z. Y. Du, Bioorg. Med. Chem. Lett., 2015, 25, 3044–3051 CrossRef CAS PubMed.
- M. C. Kraan, J. J. Haringman, M. J. Ahern, F. C. Breedveld, M. D. Smith and P. P. Tak, Rheumatology, 2000, 39, 43–49 CrossRef CAS PubMed.
- N. Ouyang, J. L. Williams, G. J. Tsioulias, J. J. Gao, M. J. Iatropoulos, L. Kopelovich, K. Kashfi and B. Rigas, Cancer Res., 2006, 66, 4503–4511 CrossRef CAS PubMed.
- B. J. Nickoloff, Y. Ben-Neriah and E. Pikarsky, J. Invest. Dermatol., 2005, 124, 10–14 CrossRef PubMed.
- T. S. Rao, J. L. Currie, A. F. Shaffer and P. C. Isakson, Inflammation, 1993, 17, 723–741 CrossRef CAS PubMed.
- C. Hauser, J. M. Dayer, F. Jaunin, B. de Rochemonteix and J. H. Saurat, Cell. Immunol., 1986, 100, 89–96 CrossRef CAS PubMed.
- A. Kock, T. Schwarz, R. Kirnbauer, A. Urbanski, P. Perry, J. C. Ansel and T. A. Luger, J. Exp. Med., 1990, 172, 1609–1614 CrossRef CAS PubMed.
- T. S. Kupper, D. W. Ballard, A. O. Chua, J. S. McGuire, P. M. Flood, M. C. Horowitz, R. Langdon, L. Lightfoot and U. Gubler, J. Exp. Med., 1986, 164, 2095–2100 CrossRef CAS PubMed.
- S. Singh and B. B. Aggarwal, J. Biol. Chem., 1995, 270, 10631–10639 CrossRef CAS PubMed.
- N. G. Carlson, W. A. Wieggel, J. Chen, A. Bacchi, S. W. Rogers and L. C. Gahring, J. Immunol., 1999, 163, 3963–3968 CAS.
- M. T. Huang, G. Ghai and C. T. Ho, Compr. Rev. Food Sci. Food Saf., 2004, 3, 127–139 CrossRef CAS.
- S. S. Han, Y. S. Keum, H. J. Seo, K. S. Chun, S. S. Lee and Y. J. Surh, Cancer Lett., 2001, 164, 119–126 CrossRef CAS PubMed.
- S. O. Kim, J. K. Kundu, Y. K. Shin, J. H. Park, M. H. Cho, T. Y. Kim and Y. J. Surh, Oncogene, 2005, 24, 2558–2567 CrossRef CAS PubMed.
- E. Zandi, D. M. Rothwarf, M. Delhase, M. Hayakawa and M. Karin, Cell, 1997, 91, 243–252 CrossRef CAS PubMed.
- Z. W. Li, W. Chu, Y. Hu, M. Delhase, T. Deerinck, M. Ellisman, R. Johnson and M. Karin, J. Exp. Med., 1999, 189, 1839–1845 CrossRef CAS PubMed.
- P. Viatour, M. P. Merville, V. Bours and A. Chariot, Trends Biochem. Sci., 2005, 1, 43–51 CrossRef PubMed.
- Y. Kim and S. M. Fischer, J. Biol. Chem., 1998, 273, 27686–27694 CrossRef CAS PubMed.
- M. Karin and M. Delhase, Semin. Immunol., 2000, 12, 85–98 CrossRef CAS PubMed.
- C. L. Carpenter and L. C. Cantley, Curr. Opin. Cell Biol., 1996, 8, 153–158 CrossRef CAS PubMed.
- M. Karin, Oncogene, 1999, 18, 6867–6874 CrossRef CAS PubMed.
- J. K. Kundu and Y. J. Surh, Mutat. Res., Fundam. Mol. Mech. Mutagen., 2005, 591, 123–146 CrossRef CAS PubMed.
- L. C. Cantley, Science, 2002, 296, 1655–1657 CrossRef CAS PubMed.
- K. M. Nicholson and N. G. Anderson, Cell. Signalling, 2002, 14, 381–395 CrossRef CAS PubMed.
- H. Shimamura, Y. Terada, T. Okado, T. Hiroyuki, S. Inoshita and S. Sadak, J. Am. Soc. Nephrol., 2003, 14, 1427–1434 CrossRef CAS PubMed.
- S. K. Selvaraj, R. K. Giri, N. Perelman, C. Johnson, P. Malik and V. K. Kalra, Blood, 2003, 102, 1515–1524 CrossRef CAS PubMed.
Footnote |
† Electronic supplementary information (ESI) available. See DOI: 10.1039/c7ra01111c |
|
This journal is © The Royal Society of Chemistry 2017 |
Click here to see how this site uses Cookies. View our privacy policy here.